1.17 Venous thromboembolism in pelvic trauma
1 Introduction
Among hospitalized patients, those who have sustained major trauma are at greatest risk for venous thromboembolism (VTE) [1–3]. Objectively documented deep vein thrombosis (DVT) is detected in 40–80% of patients with major trauma who do not receive thromboprophylaxis [1]. Death from pulmonary embolism (PE), reported in up to 2% of patients following major trauma [1, 4, 5], often occurs without warning as patients are recovering from their injuries. Thromboembolic complications of trauma require therapeutic anticoagulation with its attendant-bleeding risks, may delay hospital discharge or lead to readmissions, and substantially increase the cost of care [3, 6–8]. Due to the established high thromboembolic risks and published prophylaxis guidelines, VTE in trauma also has important medicolegal implications.
This chapter reviews the risks of DVT and PE, as well as the diagnosis, treatment, and prevention of VTE in adult patients with pelvic trauma. Since the work of Tile et al [9] was published in 2003, new literature reviews have been conducted, extensive revisions have been made, and the references updated. Practical algorithms for the diagnosis and prophylaxis of thromboembolic complications after pelvic fractures also are presented.
2 Epidemiology of venous thromboembolism in pelvic trauma
There is considerable evidence that patients with pelvic and other lower extremity fractures have a particularly high risk of thromboembolic complications [3, 10–14]. The prevalence of DVT in patients with pelvic fracture receiving no prophylaxis has been assessed in several small prospective studies ( Table 1.17-1 ) [1, 15–19]. The author′s group used bilateral contrast venography to assess DVT in 349 trauma patients with an Injury Severity Score of at least 9, none of whom received mechanical or anticoagulant thromboprophylaxis [1]. In this study, among the subgroup of 100 patients with pelvic fractures, DVT was found in 61% and proximal DVT was detected in 29%. Because this was designed as a screening study, many thrombi were small and of questionable clinical significance. However, when these venographic DVT rates are compared with those obtained after hip and knee arthroplasty or hip fracture repair, the study clearly demonstrated that patients with pelvic trauma should be considered at high risk for DVT. The combined rate of proximal DVT was 10% in the two studies [17, 18] that used Doppler ultrasound (DUS) to screen patients with pelvic fracture who were not receiving thromboprophylaxis. In a study from India [19], DVT was detected in 27% of 56 patients who did not receive thromboprophylaxis after surgical repair of isolated acetabular fractures and who were screened with computed tomographic (CT) venography 5 days after surgery.
Deep vein thrombosis has been reported to be the most common complication associated with operative repair of acetabular fractures, occurring in 27% of 131 patients despite perioperative use of combined anticoagulant and mechanical thromboprophylaxis [20]. Serial DUS studies of the leg veins identified DVT in 15% of patients with pelvic fractures who were given mechanical prophylaxis [21]. In another study [13], 19% of 57 patients with pelvic fracture who received low-molecular-weight heparin (LMWH) thromboprophylaxis were found to have DVT on DUS screening. More than 90% of venous thrombi occur ipsilateral to the lower extremity injury [19, 22, 23].
Several investigators [23–28] have used magnetic resonance venography (MRV) to assess DVT in patients with pelvic fractures. Montgomery et al [23, 24] obtained MRV in 101 patients with acetabular fracture; a total of 34% were considered to have preoperative proximal DVT based on MRV. Half of the thrombi were located in the pelvic veins: internal iliac (29%), external iliac (12%), and common iliac (8%). If these MRV abnormalities truly represent thrombi, most would not be detected by the more commonly used DUS. Preoperative screening with MRV in 644 patients with pelvic trauma identified DVT in 16%, with 57% of these found in the pelvic veins [28]. Another study [25], which compared two mechanical prophylaxis options in patients with pelvic fracture, also reported that almost half of abnormalities identified as proximal DVT by MRV or DUS were in the pelvic veins. However, the diagnostic accuracy of MRV as a screening test in asymptomatic patients with pelvic fracture remains uncertain. Of concern is a small study [26] in which patients with pelvic trauma had postoperative MRV as well as selective pelvic venography in those with positive test results. None of the four patients with positive MRVs was found to have DVT by venography. In another study [27] that screened 316 patients with pelvic fracture for asymptomatic DVT, MRV was 27 times more likely to be nondiagnostic than DUS. Interobserver agreement in the interpretation of MRV in patients with pelvic fractures is also poor [29]. Therefore, more evidence is required before MRV can be accepted as an accurate diagnostic or screening test for pelvic vein thrombosis.
Before the routine use of thromboprophylaxis, PE was the most common cause of death after pelvic fractures [30]. The risk of symptomatic PE in patients who have had a pelvic fracture has been reported as 2–12% [27, 31–36], and fatal PE has been reported in up to 10% [27, 31–35, 37–40]. A retrospective review [31] of 60 consecutive patients with minor pelvic fractures (ie, isolated, stable injuries not requiring surgery and allowing mobilization within 48 hours of injury) reported PE in 12% and fatal PE in 10%. In another retrospective review [32], clinically suspected PE was confirmed by pulmonary angiography in 8% of 108 patients with pelvic fracture, most of whom had received prophylaxis. The PE rate in patients with pelvic trauma was almost five times higher than in trauma patients without pelvic fractures. Among 973 patients with fractures of the pelvis or acetabulum who received prophylaxis with subcutaneous heparin plus compression devices, the rates of PE and fatal PE were 1.7% and 0.3%, respectively [27].
The risk of symptomatic PE associated with pelvic fracture was shown to be about five times higher than that observed in patients without a pelvic fracture among more than 9,000 trauma patients given DVT prophylaxis [14, 41]. In a statewide study [42] of 318,554 trauma patients, PE was diagnosed three times more often following pelvic fractures than in the entire trauma cohort.
Symptomatic VTE and fatal PE are most common 2–5 weeks after trauma although, rarely, trauma patients may have fatal PE within a few days of injury while some patients may present with symptomatic VTE several months after injury [5, 32, 43–45]. Of particular concern to the pelvic surgeon is the development of major but clinically silent DVT between the time of injury and delayed surgical repair with massive PE occurring intraoperatively [46].
2.1 Risk factors for venous thromboembolism in trauma and pelvic fracture patients
The risk factors predisposing general trauma patients to VTE have been addressed in numerous studies ( Table 1.17-2 ) [1, 3, 47–56]. Age is an independent risk factor for thrombosis in adult trauma patients [1, 42, 48, 53, 57]. Although VTE is uncommon in pediatric patients with pelvic fractures [58], in the adult trauma population, young age itself is not an adequate protection against important thromboembolic complications. Prophylaxis should not be withheld simply because the patient is younger than some arbitrary age threshold. Spinal cord injury or the presence of a lower extremity fracture are associated with a high thromboembolic risk [1, 8, 14, 48–50, 53, 54, 56, 59, 60]. The need for one or more surgical interventions [1, 8, 52], use of a femoral venous line [52] or the presence of lower extremity venous injury [61], and the extent and duration of immobilization [1, 49] are additional risk factors for VTE. The risk of VTE was not found to be independently related to injury severity in some studies [1, 16, 56], although it was in others [3, 8, 14, 42, 48, 50, 54, 57]. These findings suggest that specific types of injuries are likely to be more important than injury severity in predicting VTE risk.
Increasing age* |
Spinal cord injury |
Lower extremity or pelvic fracture* |
Major head injury |
Lower extremity venous injury |
Femoral venous line |
Postinjury shock state |
Surgical procedure(s) performed |
Blood transfusion |
Prolonged immobility |
Previous VTE |
Morbid obesity* |
Delayed thromboprophylaxis* |
* Factors specifically shown to be important in patients with pelvic fracture. |
Few studies of patients with pelvic fracture have specifically addressed risk factors for VTE, although increasing age [10, 17, 21, 25, 62], the presence of concomitant lower extremity fractures [1, 10, 34, 42, 45, 62], morbid obesity [63], and delayed initiation of thromboprophylaxis [64, 65] have been shown to be important. The severity of the pelvic fracture does not appear to predict the risk of VTE [1, 10, 31]. Among the 100 patients with pelvic fracture who did not receive prophylaxis that the author investigated with contrast venography, the rates of DVT and proximal DVT did not differ between those who had major injuries (defined by an Abbreviated Injury Scale for the pelvis of ≥ 3 or requiring either external or open fixation) and those who had more minor pelvic injuries. Deep vein thrombosis was found in 40% of patients with isolated pelvic fractures or with additional nonorthopedic injuries, but the rate increased to 84% in patients with concomitant lower extremity fractures. Factors that have not been consistently shown to be predictive of VTE following pelvic fracture include the presence of other nonorthopedic injuries [10] or the method of fracture treatment [10, 17, 25]. The DVT rates in the 100 patients with pelvic fracture assessed by the author and managed with external fixation, internal fixation, or nonoperatively were 46%, 56%, and 66%, respectively. In contrast, a multicenter German analysis [66] of 1,266 acetabular fractures reported DVT and PE in 2.7% and 1.2% of patients managed surgically and 0.7% and 0.2% of those managed nonoperatively, respectively.
The timing of the initiation of thromboprophylaxis has an important impact on VTE rates. Among 103 patients who underwent surgical repair of pelvic fractures, proximal DVT was detected by day 10–14 DUS in only 3% of those who started LMWH prophylaxis within 24 hours of injury and in 22% of patients who started LMWH later [64]. Patients with pelvic fractures who were initiated on thromboprophylaxis with rivaroxaban more than 24 hours after injury had four times as many DVTs than those who started rivaroxaban within 24 hours [65].
Applying a formal VTE risk assessment tool [47, 67] or identifying risk factors for individual patients is not particularly useful because all patients with pelvic fractures have a moderate or high risk of VTE. There is no evidence that altering prophylaxis for patients with pelvic fracture with different estimated risks reduces adverse outcomes [13, 38]. As will be discussed further, the use of routine thromboprophylaxis in these patients is recommended and is associated with a low frequency of VTE [17, 68].
2.2 Pathogenesis of venous thromboembolism
Patients with pelvic fractures are at high risk for VTE because of the simultaneous occurrence of each of the three factors (ie, venous stasis, endothelial injury, and coagulation activation) described by Virchow in 1856 ( Table 1.17-3 ) [69–72]. Pelvic fracture and other types of major trauma are profound stimuli for activation of the coagulation system and increased thrombin generation [73–75]. Immobilization is particularly common after pelvic and lower extremity injuries and may be prolonged, leading to venous stasis that contributes to local thrombus generation and extension [76]. Finally, endothelial injury of the pelvic or leg veins also occurs commonly following lower extremity trauma and its repair [77, 78]. Whether the relatively common inherited coagulation disorders play any role in the risk of VTE in trauma patients is unknown but they are unlikely to be clinically important [79].
Venous thrombosis is generally believed to commence at or shortly after injury. Most venous thrombi in surgical patients begin in the deep veins of the calf, do not extend proximally, and remain asymptomatic ( Fig 1.17-1 ) [1, 30, 70, 72, 80, 81]. However, up to 20% of postoperative calf thrombi extend into the proximal veins [70, 80, 82–85]. Proximal thrombi are much more serious than calf DVT because at least 50% of proximal thrombi lead to PE [70, 80, 84], and approximately 10% of patients with PE die of this complication [70]. The corollary—that is, without proximal DVT, clinically significant PE is uncommon—is generally true [70, 72]. In trauma patients, most proximal DVT and PE are also clinically silent [1, 32, 81, 86]. In orthopedic trauma, DVT is more likely to originate in the proximal leg veins because of venous injury adjacent to the site of fracture [87]. It is likely that almost all hemodynamically important PE arise in the proximal deep veins, particularly the popliteal and femoral veins [88]. Some investigators believe that PE may not always arise in the deep leg veins but may also arise in the pulmonary arteries themselves, especially in patients with severe thoracic injury [53, 55].

The frequency and clinical significance of pelvic vein thrombosis in patients with pelvic fractures are unknown. Studies [23, 24] using MRV suggest that these patients are predisposed to isolated iliac vein thrombosis, which may be particularly relevant if fixation is delayed because intraoperative manipulation may result in embolization from these large thrombi. However, the frequency of these thrombi is in dispute, and clinically important pelvic vein thrombosis may be uncommon in these patients if appropriate prophylaxis has been given.
After a proximal DVT, postthrombotic syndrome will develop in 25–50% of patients and is characterized by chronic or intermittent leg swelling, sometimes accompanied by pain, hyperpigmentation, ulceration [89]. The risk of postthrombotic syndrome also appears to be increased in patients with asymptomatic DVT [90]. Furthermore, patient and societal costs associated with VTE and postthrombotic syndrome are substantial [91–93].
3 Diagnosis of clinically suspected venous thromboembolism
Despite appropriate thromboprophylaxis, a clinical suspicion of DVT or PE is common in patients with serious injuries. The diagnosis of VTE in trauma is challenging because frequent lower extremity and chest injuries produce clinical manifestations that closely mimic DVT or PE. A clinical diagnosis of DVT or PE, therefore, is unreliable in trauma patients [1, 19, 94]. Formal clinical prediction rules for DVT or PE have never been assessed in the trauma setting, and D-dimer testing is too nonspecific to be of use in these patients because virtually all results will be positive [75]. Definitive imaging studies are always required to confirm or exclude suspected VTE. Excellent reviews [95–98] of the diagnosis of DVT and PE can be found elsewhere. Although few data specific to investigation of VTE in trauma patients have been produced, a brief review from the perspective of patients with pelvic trauma is presented here.
For clinically suspected DVT, compression DUS is the diagnostic test of choice ( Fig 1.17-2 ) [96–100]. Among patients with suspected DVT, the sensitivity and specificity of DUS both exceed 95% [99, 100]. Imaging studies of the proximal veins alone are recommended to avoid false-positive results associated with calf vein imaging and because the clinical significance and management of isolated calf DVT remains uncertain [83–85, 101]. Furthermore, therapeutic anticoagulation involves risk, and the author expects appropriate thromboprophylaxis to prevent or substantially reduce extension of calf DVT. If a technically adequate DUS of the proximal leg veins cannot be performed because of the patient′s injuries or if there is a clinical suspicion of pelvic vein thrombosis and DUS does not adequately visualize these veins, alternatives are contrast venography, contrast-enhanced CT or possibly MRV, although data validating the latter two diagnostic modalities are limited for this indication [26, 102, 103]. These procedures also require transport to the medical imaging department and are expensive; contrast venography and CT require iodinated contrast whereas MRI may be incompatible with some of the metallic hardware in trauma patients.
Clinically suspected PE is more challenging largely because of the high frequency of chest wall injuries, pulmonary contusion, pleural effusion, atelectasis, fat emboli, systemic inflammatory response syndrome, and pneumonia in trauma patients. Although a chest x-ray may identify some of these other conditions, the presence of an abnormality on a plain x-ray does not exclude the diagnosis of PE. Ventilation-perfusion lung scans are of little value in patients with major trauma because they are usually not diagnostic and do not have the potential to provide an alternate diagnosis in the patients who do not have PE. Therefore, the management of trauma patients with suspected PE generally should be based on a CT pulmonary angiogram or DUS of the leg veins [96, 104, 105] ( Fig 1.17-3 ).
In a trauma patient with suspected PE, the first diagnostic decision is whether to order a CT pulmonary angiogram or DUS. The author starts with DUS of the proximal veins in a patient with suspected PE who also has clinically suspected DVT, a contraindication to CT pulmonary angiography (eg, contrast allergy, renal insufficiency, or a degree of instability that precludes transport from the intensive care unit to the medical imaging department) or a high bleeding risk. If DUS shows a proximal DVT, treatment is indicated. However, if results are negative, there is less urgency to investigate (or treat) since it is unlikely that a significant PE will develop, at least in the short term, especially if the patient is receiving anticoagulant thromboprophylaxis [104]. Although some authors [23, 106] recommend MRI to detect both proximal leg vein thrombi and pelvic vein thrombi, the author does not recommend this strategy because both the accuracy of MRV and the clinical significance of MRV findings are uncertain.


For most patients with suspected PE, the initial test is multidetector CT pulmonary angiography. If a technically adequate CT pulmonary angiogram does not show any intraluminal filling defects, PE has been ruled out. Thus, no further investigations for PE are required, and thromboprophylaxis should be continued [95–97, 105]. If the CT pulmonary angiogram shows definite PE in one or more segmental or larger pulmonary arteries, then PE is confirmed and treatment should be initiated [95–97, 105]. Additional advantages of CT pulmonary angiography include an acquisition time of less than 30 seconds and the ability to identify alternative conditions that may account for the patient′s clinical presentation, such as pulmonary contusion, fat embolism, pleural or pericardial effusion, pneumonia, diaphragmatic injury, or a subphrenic process.
With the availability of multiple-row detector CT scans, PE is being diagnosed with substantially greater frequency in trauma patients [53, 107–110]. Specifically, the detection of subsegmental PE has dramatically increased, although the clinical significance of this entity remains unknown [107–109]. Among 90 patients with serious injuries who had a routine CT pulmonary angiogram 2 days after trauma, 24% were found to have asymptomatic PE [111]. Whether the small-filling defects reported as “pulmonary emboli” truly represent embolized venous thrombi is uncertain, and the clinical consequences of these abnormalities are also unknown. Trauma surgeons must be aware of these uncertainties and should make individual decisions about how to manage patients with small filling defects on CT pulmonary angiography. The author considers all subsegmental filling defects to be “nondiagnostic” and/or of questionable significance; therefore, the author does not advocate therapeutic anticoagulation in such patients unless they have proximal DVT. This is particularly relevant to orthopedic trauma because of the high rate of small filling defects in these patients [111, 112]. In this situation, the author generally obtains bilateral proximal DUS and bases management on the results of this additional test. If proximal DVT is detected, the patient is treated, while, if no proximal DVT is detected, thromboprophylaxis is continued without additional testing or treatment.
4 Treatment of venous thromboembolism in patients with pelvic trauma
With the use of aggressive thromboprophylaxis in trauma patients, there are few symptomatic thromboembolic events. Any such breakthrough episodes usually occur at least several days after injury when the risk of bleeding, even with therapeutic anticoagulation, is generally low. The treatment of proximal DVT and major PE, therefore, is usually similar to that in nontrauma patients with therapeutic doses of subcutaneous LMWH, followed by several months of full-dose oral anticoagulation with warfarin or with the factor Xa inhibitor, rivaroxaban [113–115]. The dose of LMWH is based on body weight with no need for laboratory monitoring or subsequent dosage adjustment [113]. LMWH produces a predictable anticoagulant effect, is at least as effective as intravenous heparin, results in less bleeding, and is associated with substantial cost savings. For these reasons, LMWH is the preferred modality for early anticoagulation in most stable trauma patients with VTE [113]. Several LMWHs have been adequately studied for this indication, including dalteparin 100 U/kg SC twice daily or 200 U/kg SC once daily, enoxaparin 1 mg/kg SC twice daily or 1.5 mg/kg SC once daily, and tinzaparin 175 U/kg SC once daily. Patients who are clinically unstable or require interruptions in anticoagulation for invasive procedures, or those who have severe renal insufficiency (creatinine clearance < 30 mL/min) usually should be managed with intravenous heparin because the anticoagulant effect is readily reversible with discontinuation of the infusion and since heparin does not bioaccumulate in renal failure. If intravenous heparin is used, a 5,000 U bolus (or no bolus) followed by an initial infusion rate of 20 U/kg/h is recommended. The heparin dose should be adjusted to achieve an activated partial thromboplastin time 2.0–3.0 times the control value [113]. The use of a heparin-dosing nomogram is recommended because it usually results in more rapid therapeutic anticoagulation than physician-ordered heparinization. Daily platelet counts should be monitored in all patients receiving full-dose heparin to screen for heparin-induced thrombocytopenia, which is a life-threatening complication [116]. If the platelet count falls more than 30% without an alternative, more plausible explanation, the possibility of heparin-induced thrombocytopenia should be investigated and all sources of heparin discontinued.
Patients with recent DVT or PE do not require bed rest or reduced mobilization, and physiotherapy can normally continue [113, 117]. However, in patients with an acute iliofemoral DVT, aggressive leg manipulation should be limited for several days after anticoagulation has been initiated.
If no further invasive procedures requiring interruption of anticoagulation are planned, most trauma patients with VTE can be given an oral vitamin K antagonist such as warfarin simultaneously with LMWH or intravenous heparin and with an overlap of at least 5 days until the International Normalized Ratio (INR) has been in the therapeutic range of 2.0–3.0 for 2 days [113]. For stable patients who do not have severe renal dysfunction, an alternative to warfarin is the oral factor Xa inhibitor, rivaroxaban, which is given as a fixed dose (15 mg twice daily for 3 weeks followed by 20 mg once daily) without the need for overlapping LMWH or for laboratory monitoring [113, 115]. With a traumaassociated proximal DVT or PE, therapeutic anticoagulation should be continued for approximately 3 months if the patient is reasonably mobile by that time [113].
Clinical guidelines recommend the use of inferior vena cava filters (IVCF) in patients with confirmed proximal DVT and an absolute contraindication to acute anticoagulation [113, 118], even though no clinical trials have assessed this filter indication. In fact, there is only a single published randomized trial of IVCF use. This study evaluated the benefit of inserting a permanent IVCF in 400 patients who were also being anticoagulated for proximal DVT [119]. Although patients in whom an IVCF was inserted had fewer combined asymptomatic and symptomatic PE at 12 days, neither symptomatic PE nor mortality was reduced. Furthermore, patients who had an IVCF inserted in addition to anticoagulation experienced significantly more recurrent DVTs. The author uses only removable filters and only in situations in which therapeutic anticoagulation cannot be safely given to a patient with an acute proximal DVT [120]. Anticoagulation should be started as soon as the bleeding risk allows, usually after only a few days, because the IVCF does not prevent extension of the DVT for which it was inserted. The author has the filter removed promptly once therapeutic anticoagulation has been started, nearly always during the same hospitalization.
Upper extremity venous thrombosis, usually related to the presence of a central venous line, can generally be treated with subtherapeutic anticoagulation, and treatment duration is often shorter than for DVT arising in the leg [113]. A central venous line should be removed in a patient with line-related DVT if the line is no longer required or not functional, or if infection related to the line is suspected; however, the catheter should not be removed simply because of the presence of the DVT.
5 Prevention of venous thromboembolism in pelvic trauma
The most effective strategy to reduce symptomatic and fatal thromboembolic complications is the routine use of aggressive thromboprophylaxis [121–126]. The rationale for this approach is as follows: VTE occurs commonly after pelvic trauma, clinical screening for asymptomatic DVT is ineffective, and several hundred randomized trials demonstrate that thromboprophylaxis effectively and safely prevents clinically important VTE in various patient groups [122]. The Agency for Healthcare Research and Quality has ranked the appropriate use of thromboprophylaxis the top patient safety strategy for hospital patients based on its impact on outcomes and the strength of evidence supporting this practice [127]. Among 13,000 admissions to six regional trauma centers, 17% of the preventable deaths were attributed to PE that was related to failure to use thromboprophylaxis [128]. Among 2,593 patients at 18 trauma centers, the use of thromboprophylaxis was associated with a 45% reduction in all-cause mortality; patients who received heparin prophylaxis had a 76% decrease in mortality [129].
A number of options for prophylaxis warrant consideration in patients with pelvic fracture ( Table 1.17-4 ). Only four small randomized trials [18, 25, 64, 130] of thromboprophylaxis specifically conducted in patients with pelvic fractures have been published; however, data from clinical trials [122, 125] in other high-risk patient groups should help guide thromboprophylaxis decisions in pelvic trauma until further high-quality studies are performed in these patients.
Mechanical methods of thromboprophylaxis |
Active or passive leg movements, physiotherapy |
Graduated support stockings (GCSs) |
Sequential compression devices (SCDs) |
Venous foot pumps |
Anticoagulant methods of thromboprophylaxis |
Low-molecular-weight heparin (LMWH) |
Fondaparinux |
Oral vitamin K antagonist (warfarin) |
Oral factor Xa inhibitor (rivaroxaban) |
Combined anticoagulant and mechanical thromboprophylaxis |
LMWH and SCDs and/or GCSs |
Depending on the nature of the injuries, active or passive leg movements and mobilization may be of general benefit to trauma patients but these strategies alone are inadequate protection against VTE.
5.1 Mechanical methods of thromboprophylaxis
Mechanical thromboprophylaxis methods using graduated compression stockings (GCSs), sequential compression devices (SCDs), or venous foot pumps (VFPs) are widely used in trauma patients primarily because they do not cause bleeding. These methods all reduce venous stasis and increase flow velocity in the proximal deep veins but they do not increase endogenous fibrinolytic function [131]. The use of GCSs and/or SCDs has been shown to reduce the incidence of asymptomatic DVT in nontrauma patients who are at low or moderate thromboembolic risk (such as following general or neurological surgery) [122, 132, 133]. However, mechanical methods of thromboprophylaxis have not been evaluated nearly as rigorously as anticoagulant methods, especially in higher-risk patients including those who have sustained major trauma. In fact, GCSs have not been formally evaluated in trauma patients. In patients who sustain trauma, the effectiveness of mechanical thromboprophylaxis is controversial. Several studies and a metaanalysis [18, 22, 57, 134–136] show that mechanical thromboprophylaxis offers little or no protection compared to no prophylaxis. In studies that show some efficacy, mechanical thromboprophylaxis tends to prevent small calf thrombi without reducing the frequency of more clinically relevant proximal thrombi [122].
Fisher et al [18] randomized 73 patients with pelvic fractures to either postoperative thigh-length sequential compression devices or no thromboprophylaxis. Proximal DVT was diagnosed by serial DUS in 10.5% of control subjects and 5.7% of patients using SCDs (P = .67). Another trial randomized 107 patients who underwent surgical repair of pelvic fractures to bilateral thigh-length SCDs or bilateral foot plus calf compression devices [25]. Deep vein thrombosis was diagnosed in 19% of patients using the thigh-length SCDs and in 9% of patients wearing the foot plus calf devices (P = .3).
Pneumatic compression devices cannot be used in 35% of limbs because of lower extremity injuries, external fixators, plaster casts, or severe edema [137], and some patients do not tolerate them. If not consistently and properly used, these devices provide little or no protection. Outside of a clinical trial, compliance both by nursing staff and patients is poor [138–140]. Although mechanical thromboprophylaxis cannot be recommended as routine, single-modality thromboprophylaxis in patients with pelvic trauma, these devices are potentially useful in the following situations:
Early after injury and perioperatively in patients for whom evidence of primary hemostasis has not yet been demonstrated while waiting to initiate anticoagulant thromboprophylaxis
For trauma patients who also have intracranial bleeding or ongoing, active bleeding at other sites until the high-bleeding risk resolves [141]
Combined with anticoagulant thromboprophylaxis in an attempt to provide more effective protection in patients at very high risk [142, 143]
There is no direct evidence that SCDs are more effective than the much less expensive GCSs, although these modalities have never been compared in trauma patients [144].
The VFP is potentially advantageous in orthopedic trauma patients because it requires access to the feet only. However, the VFP is unlikely to be as efficacious in patients who sustain major trauma as in some other patient groups [145–147]. In a prospective study [147] of 135 patients with major trauma who received mechanical prophylaxis with the VFP, the DVT rate by venography was 57% and 11% of patients had proximal DVT. A randomized trial demonstrated significantly greater protection with SCDs than with the VFP in trauma patients who did not have lower extremity fractures [146]. Many patients do not tolerate these devices; one study of 221 trauma patients demonstrated that VFPs were used properly in only 59% of observations [148]. After hip or knee arthroplasty, VFPs were used correctly in only 40% of 391 observations [149].
When mechanical thromboprophylaxis is used, clinical personnel must carefully select the correct size of the device, apply the devices bilaterally (not just to the uninjured leg or to an arm and a leg) [135], and ensure optimal compliance (ie, continuous use with removal only for a short time each day for bathing or walking).
5.2 Anticoagulant methods of thromboprophylaxis
These drugs act at various steps in the coagulation process to inhibit the formation and extension of thrombi. Given the heterogeneity of trauma patients, a parenteral modality of thromboprophylaxis is required, at least initially, for most patients. LMWHs have evolved to become the modality of choice for thromboprophylaxis for most patients who sustain major trauma. Clinical practice guidelines produced by the Eastern Association for the Surgery of Trauma (EAST), the American College of Chest Physicians, and the UK National Institute of Clinical Excellence recommend LWMH for trauma patients [122, 125, 150, 151].
The author conducted a double-blind, randomized trial comparing heparin 5,000 U SC twice daily with enoxaparin 30 mg SC twice daily, both initiated within 36 hours of injury, in 344 patients who sustained serious trauma without frank intracranial bleeding [68]. Using bilateral contrast venography between day 10 and 14 as the primary outcome, the study showed that enoxaparin was significantly more efficacious than low-dose heparin (LDH) with a relative risk reduction for DVT of 30%. More importantly, there was a 58% risk reduction for proximal DVT favoring enoxaparin (15% versus 6%; P = .01). Major bleeding was uncommon and did not differ significantly between groups. This trial included 47 patients with pelvic fractures. The relative risk reduction with LMWH compared with LDH in these patients was 47% for DVT and 77% for proximal DVT. As a result of this trial and evidence from other high-risk groups, LMWH appears more efficacious than LDH in patients who sustain major trauma [122]. In another clinical trial [135], 442 trauma patients randomized to calf-length SCDs or enoxaparin 30 mg SC twice daily were screened with weekly DUS. The proximal DVT rate was three times higher in patients wearing SCDs than in those who received enoxaparin with no difference in bleeding. Specific results in the 79 patients with pelvic fracture were not reported.
The importance of early initiation of LMWH prophylaxis in patients with pelvic trauma has been shown. In patients given LMWH within 24 hours after injury, the rate of proximal DVT was seven times lower than in patients for whom prophylaxis was started later (3% versus 22%, P <.01) [64]. Neither group experienced major bleeding. At least three additional studies [55, 152, 153] demonstrate that early initiation of LMWH in trauma patients is associated with decreased thromboembolic events without an increase in bleeding. In a study [154] of 175,665 critically ill patients, thromboprophylaxis that was delayed more than 24 hours resulted in a 17% increase in hospital mortality compared with patients with prophylaxis that was started earlier. In this study, the odds ratio of hospital death in trauma patients was 1.7 (95% confidence interval, 1.2–2.3; P = .001) if thromboprophylaxis was not initiated within 24 hours of admission to the intensive care unit.
The authors of one economic analysis [155] concluded that the institutional cost per thromboembolic event prevented was only USD 279 with routine use of LMWH compared with no prophylaxis. In another cost-effectiveness study [156] of patients with lower extremity trauma, the authors concluded that use of LMWH instead of LDH resulted in a societal cost of only USD 751 for each DVT or PE prevented with LMWH and a cost per life year saved of only USD 1,017. Authors of a third economic analysis reported that use of LMWH was actually less expensive than LDH in patients with serious trauma [157].
Because patients with pelvic fracture have a high prevalence of concomitant intraabdominal and pelvic organ injuries and because retroperitoneal hematoma occurs frequently after serious pelvic fractures, concerns about anticoagulant prophylaxis-related bleeding has sometimes been a deterrent to initiation of effective thromboprophylaxis in these patients. Management of active bleeding clearly takes precedence over preventing thrombosis in major trauma. However, in most patients with pelvic trauma, the risk of further bleeding decreases rapidly after stabilization of the pelvic and other fractures, whereas the risk of clinically important VTE usually is delayed at least several days after injury. This delay provides a window of opportunity to initiate anticoagulant prophylaxis once active bleeding has been controlled and there is evidence that primary hemostasis has occurred based on results of clinical examination and hemoglobin values, supplemented, if needed, by imaging studies. A brief delay in the start of anticoagulant prophylaxis will usually still prevent thrombus propagation, embolization, and fatal PE even if venous thrombosis has already started. In the author′s experience, the incidence of delayed, significant bleeding with anticoagulant prophylaxis is extremely low once primary hemostasis has been demonstrated.
The optimal dose of LMWH has not been established in pelvic trauma. However, because of the high thromboembolic risk in these patients, the author frequently starts thromboprophylaxis with usual doses of LMWH (eg, dalteparin 5,000 U once daily or enoxaparin 30 mg twice daily), and then often doubles the dose after the patient′s condition has been clinically stable for a few days. The author has never encountered bleeding attributable to the thromboprophylaxis using this strategy. For trauma patients who weigh 100–130 kg, the LMWH dose is also doubled and, for those heavier than 130 kg, weight-appropriate thromboprophylaxis is given [158, 159]. An area of potential concern is the reduced bioavailability of standard doses of LMWH in critically ill trauma patients with generalized edema or who are being given vasopressors [160, 161]. For example, half of trauma or surgical patients given enoxaparin 30 mg SC twice daily had peak anti-Factor Xa levels less than 0.2 U/mL, which were associated with a DVT rate that was more than three times higher (37% versus 11%) than that in patients who had higher peak levels [161]. More studies are required to determine optimal dosing of LMWH in such critically ill trauma patients.
Low-dose heparin has largely been replaced by LMWH as thromboprophylaxis in patients with major trauma [68, 122, 134]. Although LDH has been shown to be safe and effective in preventing DVT, PE, and reducing all-cause mortality in low-to-moderate risk general surgical patients [122, 125], it is less effective in high-risk patients, including those undergoing hip and knee surgery and spinal cord injury and is not recommended for any of these groups [122, 134]. Studies [24, 57, 137, 162] in trauma generally report that LDH provides little or no protection against DVT compared with no prophylaxis. A metaanalysis [134] documented that PE was three times more likely to occur in a trauma patient who received LDH than LMWH. Other reasons LDH has been replaced by LMWH include a 30-fold lower risk of heparin-induced thrombocytopenia [163, 164], once- or twice-daily dosing (compared with three daily doses for LDH), and similar or lower cost.
Although oral anticoagulants are not appropriate in the acute phase after major trauma, they may be useful in preventing thromboembolic complications in high-risk patients who require prolonged hospitalization including rehabilitation [123]. The author considers oral anticoagulation with rivaroxaban [65] or full-dose warfarin (INR 2.0–3.0) in patients who have sustained major trauma and meet the following criteria: a continued high risk for thrombosis (eg, multiple lower extremity fractures or spinal cord injury); evidence that hemostasis has been achieved; no plans for immediate further invasive or surgical procedures; and the expectation that hospitalization (including rehabilitation) is likely to continue for at least several weeks. Among 197 patients with acetabular fractures who received perioperative mechanical prophylaxis followed by 3 weeks of oral anticoagulation, the incidence of symptomatic DVT or PE was only 4% with no fatal emboli [40].
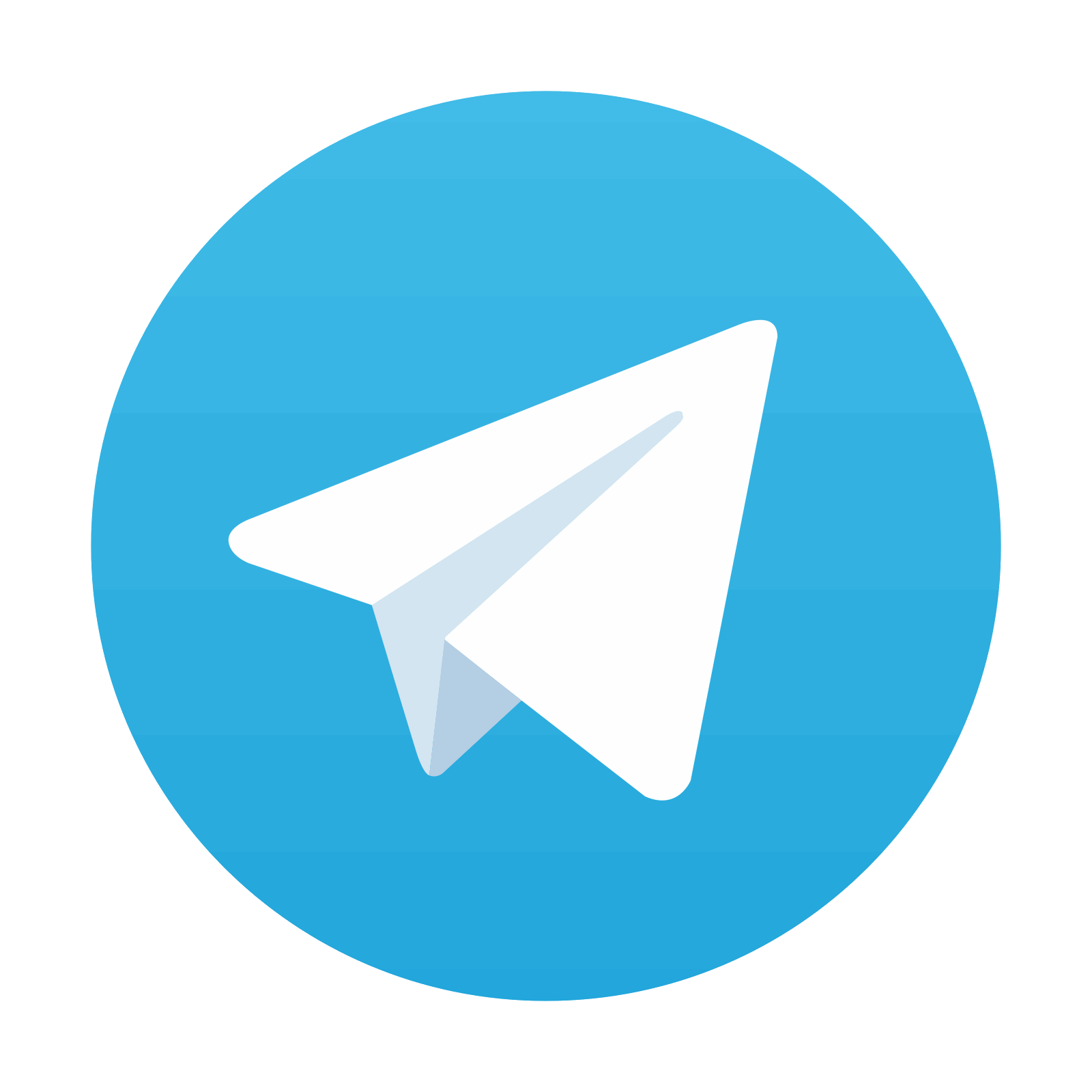
Stay updated, free articles. Join our Telegram channel

Full access? Get Clinical Tree
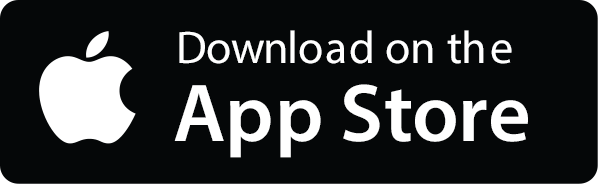
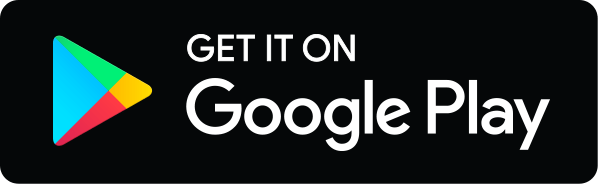
