Chapter 6 Extraspinal Techniques
Role of the peripheral joints
From the beginnings of chiropractic, practitioners have treated the nonspinal joints. D.D. Palmer1 described his treatment of the feet in 1910. Applying manual procedures to the peripheral joints is a skill that is taught in all accredited chiropractic programs.
Impairments involving the muscles, ligaments, and joints of the extremities can significantly reduce the quality or the ease of performing many important activities related to personal care, livelihood, and recreation. The rationale for treating peripheral joints includes correction of biomechanical problems and reflex-triggered functional syndromes.2 Biomechanical problems are characterized primarily by joint pain, but swelling and paresthesia may be present. These problems may be the result of trauma (sprains, strains, athletic injuries, or work injuries) or repetitive activities and postures (carpal tunnel syndrome, plantar fasciitis, or foot pronation). Reflex-triggered functional syndromes occur through a series of distortions affecting the kinetic chain. They are a result of mechanical deficiencies (short leg) or muscular insufficiencies or deformities (valgus or varus). These problems present as clinical findings associated with specific areas of the body, but the major dysfunctions are located somewhere else. For example, a patient may have acute or recurrent low back pain that appears to be localized to the sacroiliac joints, but treatment to the sacroiliac joints is ineffective. On further examination, a dysfunctional metatarsal joint is found that disturbs normal proprioceptive function, leading to sacroiliac changes.
Temporomandibular joint
The weight of the head must be balanced and stabilized atop the spine. A biomechanical relationship exists between the forces developed in stabilization of the cervical spinal segments, tension in the deep cervical fascia, movements of the temporomandibular joints (TMJs), and activity of the hyoid bone muscles, as well as the structures of the shoulder girdle (Figure 6-1). More importantly, postural stresses, muscle tone, malocclusion of the teeth, and joint dysfunction have clinical relationships with neck pain, headache, orofacial pain, and abnormalities of chewing and swallowing. An association is formed between two of the body’s most complicated joint systems—the TMJ and the atlanto-occipital joint. Both of these joint systems should be evaluated in patients complaining of head and neck pain.
Functional anatomy
Osseous Structures
The mandible, the largest and strongest bone of the face, articulates with the temporal bones while accommodating the lower teeth (Figure 6-2). The body of the mandible runs horizontally and has two posterior rami. The rami are perpendicular to the body and form an inferior palpable angle. Each ramus has two processes: the coronoid process, serving as a point of attachment for muscles, and the condylar process, for articulation with the temporal bone via the intra-articular disc. Lines drawn through the axis of each condyle intersect just anterior to the foramen magnum, the significance of which is in visualizing a line of correction for manipulative procedures (Figure 6-3). The temporal bone has a concave mandibular fossa, with the convex articular eminence just anterior to it.
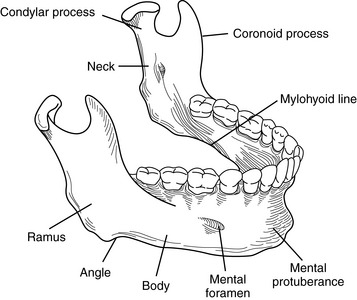
Figure 6-2 The osseous components of the mandible.
(Modified from Hertling D, Kessler RM: Management of common musculoskeletal disorders: Physical therapy principles and methods, ed 2, Philadelphia, 1990, JB Lippincott.)
Functionally, the mandibular fossa serves as a receptacle for the condyles when the joint is in a closed-packed position (teeth approximated). During opening, closing, protrusion, and retrusion, the convex surface of the condyle must move over the convex surface of the articular eminence (Figure 6-4). The existence of the intraarticular disc compensates functionally for the incongruity of the two opposing convex surfaces.3 The disc also separates the joint into an upper and lower portion or compartment, each with synovial linings. The outer edges of the disc are connected to the joint capsule.
Ligamentous Structures
Four ligaments serve as secondary stabilizers for the joint. They are the articular capsule, temporomandibular ligament, stylomandibular ligament, and sphenomandibular ligament (Figure 6-5). The primary function of the joint capsule is to enclose the joint, but because the disc is tethered to it, the joint capsule also causes the disc to move forward when the condyle moves forward. The temporomandibular ligament is the main suspensory ligament of the mandible during opening movements of the jaw. It also prevents excessive forward, backward, and lateral movements. The stylomandibular ligament prevents excessive anterior movement of the mandible and, as such, serves as a stop for the mandible in extreme opening. The sphenomandibular ligament functions as a suspensory ligament for the mandible during wide opening of the joint.
A mandibular-malleolar ligament connecting the neck and anterior process of the malleus to the medioposterior aspect of the joint capsule has been reported. 4,5 The clinical significance of this structure lies in making an anatomic connection between the TMJ and the middle ear. The mandibular malleolar ligament passes through the petrotympanic fissure to connect the malleolus to the meniscus and the capsular ligament of the TMJ. The anterior tympanic artery, which is responsible for supplying blood to the tissue around the tympanic membrane, and the chorda tympani nerve, which gives pain sensation to the tongue, also travel through this fissure. Irritation to these structures can cause symptoms such as ear pain, tinnitus, vertigo, subjective hearing loss, hyperacusis, tongue pain, and muscle pain.
Musculature
The primary movers of the mandible in elevation are the temporalis, masseter, and medial pterygoid muscles (Table 6-1 and Figure 6-6). The posterior fibers of the temporalis also retract the mandible while maintaining the condyles posteriorly. The superficial fibers of the masseter protrude the jaw, and the deep fibers act as a retractor. The deep fibers also attach to the lateral aspect of the joint capsule. The medial pterygoid can protrude the mandible, as well as deviate the jaw laterally (Figure 6-7).
TABLE 6-1 Actions of the Muscles of the Temporomandibular Joint
Action | Muscles |
---|---|
Mandibular elevation (closing) | Temporalis, masseter, and medial pterygoid |
Mandibular depression (opening) | Lateral pterygoid, suprahyoid, and infrahyoid |
Protrusion (anterior glide) | Superficial fibers of the masseter, medial pterygoid, and lateral pterygoid |
Retrusion (posterior glide) | Temporalis and deep fibers of the masseter |
Lateral glide | Medial pterygoid and lateral pterygoid |
Hyoid elevation | Stylohyoid, mylohyoid, and digastrics |
Hyoid depression | Infrahyoid |
The suprahyoid muscle group is composed of the digastric, stylohyoid, geniohyoid, and the mylohyoid muscles (Figure 6-8). The digastric muscle pulls the mandible downward and in a posterior direction. The stylohyoid muscle initiates and assists jaw opening, but also draws the hyoid bone upward and backward when the mandible is fixed. The geniohyoid muscle pulls the mandible downward and backward. The mylohyoid muscle elevates the floor of the mouth and assists in depressing the mandible when the hyoid is fixed or in elevating the hyoid when the mandible is fixed.
Biomechanics
Posture and Alignment
The TMJ undergoes the coupled motions of rotation and translation. Rotational movement of the condyle occurs about a transverse axis between the condyle and the intraarticular disc. This movement causes the first 12 to 15 mm of mandibular opening and closing. Translational movement of the condyle consists of a downward and forward gliding movement of the disc-condyle complex and depends on the synchronous movement of disc and condyle (Figure 6-9). Rotational movements occur mainly in the inferior (subdiscal) joint space, and translational movements occur mainly in the superior (supradiscal) joint space.
Mandibular movement is a dynamic combination of opening, closing, anterior glide (protrusion), posterior glide (retrusion), and lateral glide. Mandibular opening involves the contraction of the lateral pterygoids and digastrics, with assistance from the suprahyoid and infrahyoid muscles. Indirectly, the posterior cervical muscles must contract to prevent neck flexion and permit the mandible to drop away from the cranium. The first few degrees of opening are rotational movements about a transverse axis. Then the condyle and disc complex must translate forward over the articular eminence, allowing condylar rotation to continue. The closing sequence is the reverse. The condyle and disc complex translate posteriorly with condylar rotation, which brings the joint to its resting position, or intercuspal position. The masseter, medial pterygoid, and temporalis muscles are responsible for this action. Table 6-2 lists the osteokinematic and arthrokinematic movements of the TMJ.
TABLE 6-2 Arthrokinematic and Osteokinematic Movements of the Temporomandibular Joint
Osteokinematic Movements | Arthrokinematic Movements | |
---|---|---|
Mandibular elevation and depression | 40 to 60 mm of incisor separation | Combination of spin and glide occurring in the upper and lower joint compartments |
Mandibular retraction and protrusion | 5 to 10 mm of incisor separation | Glide movement occurring in the upper joint compartment |
Mandibular lateral deviation | 5 to 10 mm of incisor separation | Glide, spin, and angulation occurring mostly in the upper compartment but also in the lower joint space |
Lateral glide of the mandible involves a pivoting rotation of the condyle on the side to which the mandible is moving and a translation of the other condyle. For lateral glide to the right, the left lateral pterygoid and the anterior bellies of both the digastrics contract, causing the left condyle to move downward, forward, and medially. Meanwhile, contraction of the right temporalis and the right lateral pterygoid rotates the right condyle in the fossa and displaces the mandible to the right. Box 6-1 identifies the close-packed and loose-packed positions for the TMJ.
Evaluation
Problems affecting the TMJ can be broadly classified into developmental abnormalities, intracapsular diseases, and dysfunctional conditions (Box 6-2). Developmental abnormalities include hypoplasia, hyperplasia, impingements of the coronoid process, chondromas, and ossification of ligaments, such as the stylohyoid ligament (Eagle syndrome). The intracapsular diseases include degenerative arthritis, osteochondritis, rheumatoid arthritis, psoriatic arthritis, synovial chondromatosis, infections, steroid necrosis, gout, and metastatic tumors.6 Developmental abnormalities and intracapsular diseases must be ruled out by using appropriate evaluative procedures, including diagnostic imaging and clinical laboratory studies. The focus here is on the dysfunctional conditions affecting the TMJ, which are categorized as extracapsular (myofascial pain syndromes and muscular imbalance); capsular (sprain, hypomobility and hypermobility, and synovial folds); and intracapsular (disc displacement and disc adhesions).
BOX 6-2 Classification of Problems Affecting the Temporomandibular Joint
Inspect the face and jaw, looking for bony or soft tissue asymmetry, misalignment of the teeth, and evidence of swelling (Figure 6-10). Observe the jaw during opening and closing to identify excursional deviations and the presence of clicking7 8 9 (Figure 6-11). Ask the patient if the movements are painful. Normal opening should accommodate three of the patient’s fingers inserted between the incisors. If not, hypomobility resulting from joint dysfunction or a closed lock as a result of disc displacement should be suspected. If opening beyond three fingers occurs, hypermobility from capsular attenuation is likely (Figure 6-12).
Palpate the joint by placing the fifth digit into the patient’s external auditory meatus with the palmar surface forward (Figure 6-13). Before placing the finger into the ear, push on the tragus to determine if the external ear canal is painful. The position of the condyles and intra-articular clicking can be felt from within the external canal. The lateral aspect of the joint and capsule can be palpated externally for position, pain, and movement disorders (Figure 6-14). Palpate the muscles to determine changes in tone, texture, and tenderness. The lateral pterygoid can be palpated intraorally by following the buccal mucosa to the medial aspect of the TMJ just proximal to the tonsils (Figures 6-15 and 6-16). According to investigations, the lateral pterygoid muscle is practically inaccessible for intraoral palpation because of topographic and anatomic reasons.10 11 12 13 Palpation of the lateral pterygoid muscle is also characterized by poor interexaminer agreement.10 In contrast to these reports, Stelzenmüller and associates14 reliably confirmed the palpation of the lateral pterygoid muscle, which was controlled by two imaging procedures and electromyography. All three of the procedures confirmed palpation. The difficulty in reliably identifying the muscle seems to be due to the fact that the medial pterygoid muscle must be passed before palpating the lateral pterygoid muscle.14 Note also the position and movement of the hyoid bone, as well as the state of the associated soft tissues.
Perform accessory motions of the TMJ by placing the gloved thumbs intraorally over the lower teeth and wrapping the fingers around the mandible externally (Figure 6-17). Apply passive stress in long-axis distraction, lateral glide, and anterior-to-posterior (A-P) glide (Box 6-3). Normally, a springing end feel is perceived (Figure 6-18).
Adjustive procedures
The manipulative techniques used to treat TMJ disorders aim to restore normal joint mechanics, which will then ideally allow full pain-free functioning of the mandible. Two basic types of adjustive procedures are used for the different forms of TMJ dysfunction: distraction and translation techniques (Box 6-4). See Box 5-3 for abbreviations used in illustrating techniques.
Distraction Techniques
Translation Techniques
Shoulder
The primary role of the shoulder is to place the hand in a functional position. To achieve this function, a great deal of joint mobility is necessary, which requires complex anatomy and biomechanics. The shoulder is not one joint, but rather is a relationship of anatomic and physiologic joints, forming a four-joint complex. The glenohumeral joint is a true anatomic joint and forms the shoulder proper. The sternoclavicular and acromioclavicular joints are also true anatomic joints formed between the manubrium of the sternum, the clavicle, and the acromial process of the scapula, respectively. The scapulocostal joint lacks a joint capsule and is therefore considered a physiologic joint, necessary to allow the smooth gliding of the scapula over the ribs (Figure 6-26).
Functional anatomy
Osseous Structures
The convex articular surface of the proximal humerus is directed slightly posteriorly, medially, and superiorly, and is met by the articular surface of the glenoid fossa of the scapula (Figure 6-27). A 45-degree angle is formed between the articular surface and the shaft of the humerus. The glenoid fossa is not a deep impression into the bone of the scapula, and by itself it is incongruous with the humeral head. The glenoid labrum is a fibrocartilaginous rim that encircles the fossa and provides a greater surface area of contact for the humerus, which helps provide some stability (Figure 6-28).
The S-shaped clavicle provides for more movement during elevation of the arm. The distal third of the clavicle is concave anteriorly, which directs the distal articular surface anteriorly and somewhat superiorly. The proximal end of the clavicle articulates with the upper and lateral edge of the manubrium and the superior surface of the first rib costocartilage (Figure 6-29). An intra-articular disc lies between the clavicle and manubrium joint surfaces and is important in preventing medial dislocations of the clavicle.
The scapula lies at a 30-degree angle away from the coronal plane and forms a 60-degree angle with the clavicle15 (Figure 6-30). It has the coracoid process for muscular attachment, projecting anteriorly and lying just medial to the glenoid fossa. The spine of the scapula arises from the medial border at about the T3 level and courses laterally and superiorly, ending as the acromion process.
Ligamentous Structures
Many ligaments are associated with the shoulder, connecting one bone to another and providing a secondary source of joint stability (Figure 6-31). With the varied movements the shoulder can perform, these ligaments will become either slack or taut. Remember that a ligament is painlessly palpated unless it is injured or stretched.
The acromioclavicular ligament strengthens the superior aspect of the joint capsule. It is intrinsically weak, however, and gives way when a force is applied to the acromion process or glenohumeral joint from above (Figure 6-32). The major stabilizing ligaments of the acromioclavicular joint are the coracoclavicular ligaments, which include the conoid and trapezoid ligaments. The conoid ligament twists on itself as it connects between the coracoid process and clavicle. It prevents excessive superior movement of the clavicle on the acromion, as well as retraction of the scapula, by not allowing the scapuloclavicular angle to widen. Furthermore, the conoid ligament tightens on humeral abduction, causing axial rotation of the clavicle that is necessary for full elevation of the arm. The trapezoid ligament also connects between the coracoid process and the clavicle, but lies distal to the conoid ligament. Its role is to check lateral movement of the clavicle, thereby preventing overriding of the clavicle on the acromion process. The trapezoid ligament also prevents excessive scapular protraction by not allowing the scapuloclavicular angle to narrow.
The sternoclavicular joint capsule is reinforced anteriorly and posteriorly by the anterior and posterior sternoclavicular ligaments, respectively (see Figure 6-32). The interclavicular ligaments reinforce the capsule superiorly. Lying just lateral to the joint, the costoclavicular ligament attaches between the clavicle and first rib and serves to check elevation of the clavicle. Its posterior fibers prevent medial movement, and the anterior fibers prevent lateral movement of the clavicle.
Musculature
Because of the high degree of mobility and the numerous muscles necessary to provide stability to the joint, eight or nine bursae are found about the shoulder joint to reduce friction between the moving parts. Irritation to the bursae, leading to an inflammatory response, is a common clinical occurrence. Of specific clinical significance are the subscapular bursae and the subacromial or subdeltoid bursae (see Figure 6-31). The subscapular bursa lies beneath the subscapularis muscle and overlies as well as communicates with the anterior joint capsule. Distension of this bursa occurs with articular effusion. The subacromial or subdeltoid bursa extends over the supraspinatus tendon and under the acromion process and deltoid muscle (Figure 6-33). It is susceptible to impingement beneath the acromial arch, and inflammation often follows supraspinatus tendinitis.
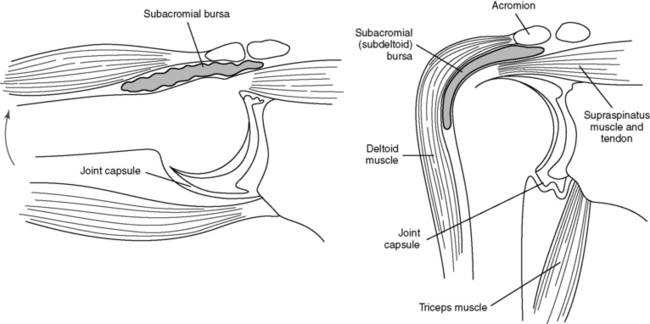
Figure 6-33 Subacromial bursa. A, With humerus pendulous. B, With humerus abducted.
(Modified from Hertling D, Kessler RM: Management of common musculoskeletal disorders: Physical therapy principles and methods, ed 2, Philadelphia, 1990, JB Lippincott.)
A review of the location and function of the numerous muscles that stabilize and supply the force for performing the varied movements of the shoulder is necessary to understand the dysfunctional conditions that affect the shoulder joint complex (Table 6-3). Although muscle tendons tend to lend stability to the joint, they do not prevent downward dislocation. The rotator cuff is composed of the supraspinatus, infraspinatus, teres minor, and subscapularis muscles. It is most notably the horizontal running fibers that prevent dislocation as they check the lateral excursion of the glenoid cavity, which in turn allows downward movement of the humerus. The factors preventing downward dislocation include the slope of the glenoid fossa, which forces the humerus laterally as it is pulled down; tightening of the upper part of the capsule and of the coracohumeral ligament; and the activity of the supraspinatus muscle working with the posterior fibers of the deltoid.
TABLE 6-3 Actions of the Muscles of the Shoulder Joint Complex
Action | Muscles |
---|---|
Flexion | Anterior deltoid, coracobrachialis, and pectoralis major (clavicular) |
Extension | Latissimus dorsi, teres major, and posterior deltoid |
Abduction | Middle deltoid, supraspinatus, and serratus anterior (scapular stability) |
Adduction | Pectoralis major and latissimus dorsi |
External rotation | Infraspinatus, teres minor, and posterior deltoid |
Internal rotation | Subscapularis, pectoralis major, latissimus dorsi, teres major, and anterior deltoid |
Scapular stabilization | Trapezius, serratus anterior, and rhomboids |
Scapular retraction (medial glide) | Rhomboid major and minor |
Scapular elevation | Trapezius and levator scapulae |
Biomechanics
Scapulohumeral Rhythm
For the arm to be abducted from the side to overhead, motion of the scapula must be simultaneous and synchronous. During the first 30 degrees of abduction, the scapula seeks a stable position on the rib cage through contraction of the trapezius, serratus anterior, and rhomboid muscles (Figure 6-34). Beyond 30 degrees, 2 degrees of glenohumeral movement occur for every 1 degree of scapulocostal movement. Thus, 15 degrees of arm abduction results from 10 degrees of glenohumeral joint movement and 5 degrees of scapulocostal joint movement. Rotation of the scapula during abduction also enhances mechanical stability by bringing the glenoid fossa directly under the humeral head.
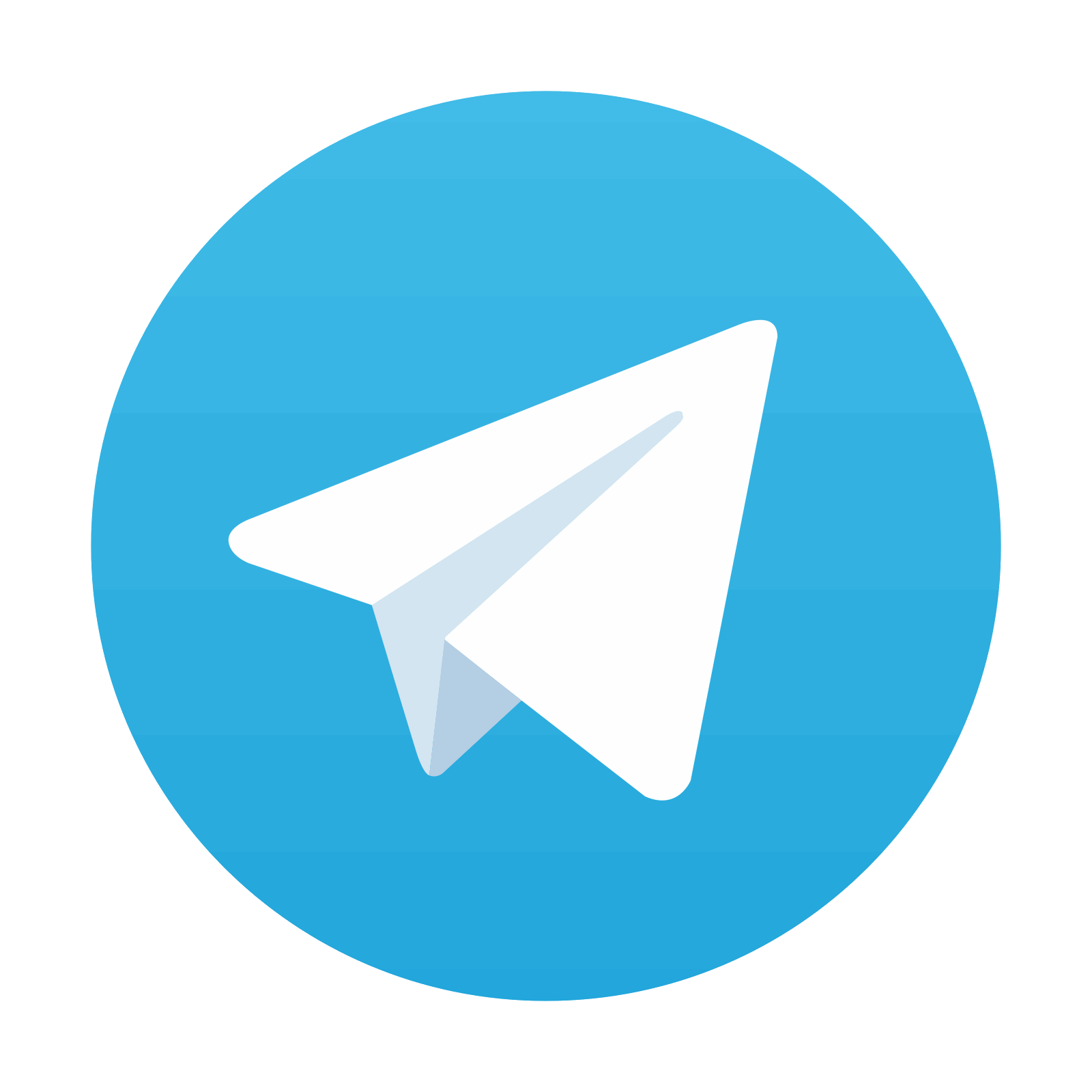
Stay updated, free articles. Join our Telegram channel

Full access? Get Clinical Tree
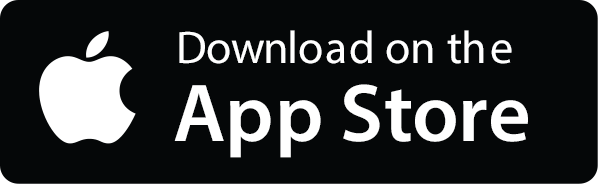
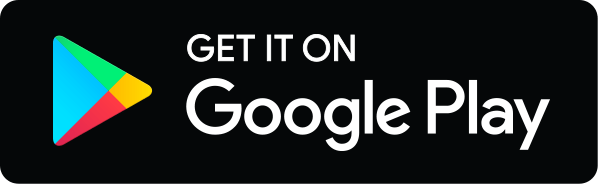