The wrist has often been described as an irrelevant articulation that can be fused without causing much functional impairment to the upper extremity. Undoubtedly, patients with a fused wrist may recover enough grip strength so as to return to their previous occupation; their hands, however, will never be completely normal. A stiff wrist does not allow, for instance, washing one’s own back, dusting a low surface, turning a steering wheel, or beating an egg without overloading the adjacent upper limb articulations. If the wrist is fused, the hand can reach distant objects, but it cannot be properly oriented to grasp, push, or manipulate them effectively. Certainly this is one of the most important roles of the wrist: to place the hand in the position that ensures its maximal efficiency with minimal energy cost.
If properly identified and treated, wrist injuries tend to heal without sequelae, with a very low complication rate. When there is a complication, the carpus may remain aligned or it may collapse in a variety of ways. The articular cartilage may deteriorate or remain normal and asymptomatic. Identifying which injuries will evolve into significant dysfunctions is not always easy. How does one prevent an injured wrist from becoming unstable? How does one differentiate between carpal instability and carpal misalignment? There are no good answers to these questions; certainly, the more these subjects are analyzed, the more unknowns arise, leading to the realization that further research is needed.
Progress is being made, however, and the proof can be found in this chapter. Only 5 years have passed since the sixth edition of this book was published, yet most chapters have had to be extensively rewritten. The goals have been: (1) to incorporate new knowledge acquired during this period, (2) to propose changes to the classification of carpal disorders, (3) to question misleading terminology, and (4) to eliminate concepts that the test of time has proved obsolete. The book’s title itself has been modified to emphasize the fact that not all carpal misalignments are necessarily unstable, and that normal alignment does not guarantee stability.
Undeniably, there is a growing interest in the wrist. What follows is an earnest attempt to respond to that interest by offering an updated description of how to assess and treat the various disorders of the wrist, truly one of the most complex joints of the human body.
Terminology.
Most terms used to describe carpal misalignment and wrist instability were introduced in the 1970s, when these subjects were discussed for the first time. Some of them have passed the test of time and are still useful and widely utilized; others have been proven less effective, if not misleading or totally incorrect, and need to be revised. The following are some suggestions in this regard. The goal is to improve the clarity of what is taught and to avoid misinterpretation of the facts.
The term rotary (or rotatory) instability of the scaphoid , used to describe the advanced stage of carpal collapse where the scaphoid has lost its oblique alignment relative to the radius, is not correct: The scaphoid not only undergoes flexion and pronation but also translates dorsolaterally. There is never a pure rotation as implied by the adjective “rotary” or “rotatory.” Furthermore, such an advanced stage of misalignment involves not only a complex displacement of the scaphoid but also of the lunate, which should be mentioned as well.
The terms dorsal intercalated segment instability (DISI) and volar intercalated segment instability (VISI) seem quite appropriate for cases where the entire proximal carpal row adopts a position of abnormal extension or flexion in relation to the long axis of the radius. However, its use to define deformities secondary to dissociative carpal instabilities may create some confusion: only the lunate is in DISI not the scaphoid, which goes into flexion and pronation. Both are intercalated bones, and the deformity of each should be defined separately to prevent confusion; the scaphoid flexes and pronates, while the lunate extends.
While reading the literature, it is not uncommon to find confounding descriptions of wrist pathology that are a sign of a limited understanding of the basic principles of its mechanics. A typical example would be the use of redundant expressions such as “rotation into flexion” or “rotation into extension”—“flexion” or “extension” is enough. Another example is the use of “dorsiflexion” or “palmar-flexion.” The first is a contradiction and the second is another redundancy; “extension” and “flexion” need no embellishments.
Although radial and ulnar deviation are widely accepted expressions, following the recommendations of the Committee on Standardization of Nomenclature of the International Federation of Societies for Surgery of the Hand (IFSSH), they should be replaced by radial and ulnar inclination . An inclination is a mathematical term used to define the angle between two lines or planes. The term deviation is to be used when something departs from an acceptable standard or norm, and this is not what happens when the wrist is moved.
The inclination of the distal articular surface of the radius in relation to its longitudinal axis is frequently referred to as radial tilt , an expression that could be misinterpreted as an inclination toward the radial side. We suggest using the term ulnar tilt of the radius ; for the same reason, it is best to use the term volar tilt to refer to the anterior inclination of the radius.
By consensus, wrist joints are termed by juxtaposing the names of the bones that meet in that articulation in an orderly manner, from proximal to distal and from radial to ulnar. Ligaments are named in a similar way. Based on this, the central midcarpal articulation should not be called “capitolunate” but “lunocapitate,” and the ligaments connecting the triquetrum to the lunate should not be called “triquetrolunate” but “lunotriquetral.”
Wrist Anatomy
Carpal disorders cannot be properly diagnosed without a first-rate understanding of what is normal and what is abnormal in a symptomatic wrist. This requires a thorough knowledge of both the anatomy and mechanics of the normal wrist. This section reviews the former. The terminology used from here on is in accordance with the recommendations made by the IFSSH, as well as by the International Wrist Investigators’ Workshop (IWIW). A list of abbreviations used in this chapter is shown in Table 13.1 .
Acronym | Definition |
---|---|
AAOS | American Academy of Orthopedic Surgeons |
AIN | Anterior interosseous nerve |
APL | Abductor pollicis longus |
ASSH | American Society for Surgery of the Hand |
CIC | Carpal instability complex |
CID | Carpal instability dissociative |
CIND | Carpal instability nondissociative |
CMC | Carpometacarpal |
DASH | Disabilities of the arm, shoulder, and hand |
DISI | Dorsal intercalated segment instability |
DRUJ | Distal radioulnar joint |
DT | Dart thrower’s |
ECRB | Extensor carpi radialis brevis |
ECRL | Extensor carpi radialis longus |
ECU | Extensor carpi ulnaris |
EPL | Extensor pollicis longus |
FCR | Flexor carpi radialis |
FCU | Flexor carpi ulnaris |
FPB | Flexor pollicis brevis |
FPL | Flexor pollicis longus |
FR | Flexor retinaculum |
L | Lunate |
LC | Lunocapitate |
LTq | Lunotriquetral |
RASL | Reduction association scapholunate |
RL | Radiolunate |
RS | Radioscaphoid |
RSC | Radioscaphocapitate |
RSL | Radioscapholunate |
S | Scaphoid |
SC | Scaphocapitate |
SL | Scapholunate |
SLD | Scapholunate dissociation |
SLIL | Scapholunate interosseus ligament |
SNAC | Scaphoid nonunion advanced collapse |
STT | Scaphotrapeziotrapezoid |
TFCC | Triangular fibrocartilage complex |
Tq | Triquetrum |
TqC | Triquetrocapitate |
TqH | Triquetrohamate |
VAS | Visual analog scale |
VISI | Volar intercalated segment instability |
Osseous Anatomy
The wrist is a complex, composite joint formed by 20 interdependent articulations binding 15 bones: radius, ulna, 8 carpal bones, and the bases of 5 metacarpals. In the frontal (coronal) plane, the carpus is arranged in two rows (proximal and distal). The distal carpal row consists of 4 tightly bound bones (i.e., trapezium, trapezoid, capitate, hamate) with little mobility between them. The proximal carpal row, by contrast, exhibits substantial intercarpal mobility. It consists of three bones (i.e., scaphoid, lunate, triquetrum), interconnected by two intercarpal joints: scapholunate (SL) and lunotriquetral (LTq) ( Figure 13.1 ). The pisiform, usually considered a proximal row bone, is actually a sesamoid bone that increases the lever arm of the flexor carpi ulnaris (FCU) tendon.

The radiocarpal joint is an oval-shaped glenoid articulation that connects the proximal convexities of the scaphoid, lunate and triquetrum—the so-called “carpal condyle”—with the antebrachial glenoid formed by the shallow concavities of the distal radius and triangular fibrocartilage complex (TFCC). The distal radius is tilted in two planes: the sagittal plane, where there is an average 10 degrees inclination toward the palm, and the frontal plane, where there is an average inclination toward the ulna of 24 degrees. The proximal joint surface of the scaphoid is more curved than that of the lunate. To ensure articular congruency, the radius has two articular facets (i.e., scaphoid and lunate fossae), often separated by a cartilaginous sagittal ridge (i.e., interfacet prominence).
The midcarpal joint is a combination of three types of articulations. On the radial side, the convex distal surface of the scaphoid articulates with the concavity formed by the trapezium and the trapezoid—the scaphotrapeziotrapezoid (STT) joint. This is also known as the radial column. The central portion of the midcarpal joint is concave proximally and convex distally and has two sectors: the scaphocapitate (SC) and lunocapitate (LC) joints. The column formed by the lunate and capitate is also known as the central column. The lunate may have only one distal facet articulating with the capitate (lunate type I) or have two distal facets to articulate with the capitate and proximal pole of the hamate (lunate type II). On the ulnar side, the triquetrum articulates with the hamate through the helicoidally shaped triquetrohamate (TqH) joint. This is known as the ulnar column.
The bones of the central column are collinear in the sagittal plane in only one-third of cases. In another 30%, the lunate is slightly flexed relative to the longitudinal axis of the forearm, while in the remaining 40% is slightly extended. The scaphoid is placed obliquely in ~45 degrees of flexion in relation to the radius (range 30-60 degrees) to provide support to the thumb metacarpal, which is located anteriorly to the plane of the capitate.
In the axial (transverse) plane, the carpus forms the carpal tunnel, a palmar concavity, enclosed by the transverse carpal ligament (also known as the flexor retinaculum). The narrowest portion of the carpal tunnel is located at the level of the distal carpal row.
Ligamentous Anatomy
The bones of the wrist are interconnected by a complex arrangement of ligaments ( Figure 13.2, A–C ). Some ligaments are mechanically important structures, formed by tightly packed collagen fibers with a minimal amount of sensory corpuscles. Others are sensorially important structures with a rich population of Ruffini, Pacini, or Golgi corpuscles embedded within a less dense structure of collagen fibers. The former are static structures designed to hold the bones together. The latter provide the necessary proprioceptive information that the sensorimotor system uses to guarantee joint stability.

The ligaments are extracapsular or intracapsular. Only three are extracapsular: the transverse carpal ligament, and the two ligaments that connect the pisiform to the hook of the hamate and to the base of the fifth metacarpal. All other ligaments are contained within the depth of the capsule, surrounded by sheaths of loose connective tissue.
Two categories of intracapsular ligaments exist: extrinsic and intrinsic. Extrinsic ligaments connect the forearm with the carpus, whereas intrinsic ligaments originate and insert within the carpus ( Figure 13.3, A, B ). Histological and biomechanical differences exist between the two types. Extrinsic ligaments are mostly inserted onto bone, whereas intrinsic ligaments insert mostly onto cartilage. The extrinsic ligaments are more elastic and less resistant to traction (i.e., lower yield strength) than most intrinsic ligaments. Not surprisingly, the extrinsic ligaments tend to sustain midsubstance ruptures, whereas the intrinsic ligaments are more frequently avulsed than ruptured. The best way to assess the intracapsular ligaments is to use an arthroscope. Inside the joint, most extrinsic ligaments can be identified under a thin synovial sheath.

Extrinsic Ligaments
There are four palmar radiocarpal ligaments: radioscaphoid (RS), radioscaphocapitate (RSC), long radiolunate, and short radiolunate ligaments (long RL and short RL). The first three originate from the anterolateral margin of the distal radius and take an oblique course to insert onto the proximal edge of the scaphoid tuberosity (RS ligament), the palmar aspect of the capitate (RSC ligament), and the palmar surface of the lunate (long RL ligament). The short RL ligament originates from the anteromedial margin of the distal radius and has a vertical direction until merging with fibers of the long RL ligament. The RSC ligament courses around the palmar concavity of the scaphoid, forming a sling over which the scaphoid rotates. Between the two diverging RSC and long RL ligaments is the so-called “interligamentous sulcus” (i.e., space of Poirier), which represents a weak zone through which perilunate dislocations frequently occur.
There are three palmar ulnocarpal ligaments: one superficial (ulnocapitate) and two deep (ulnotriquetral and ulnolunate). The ulnocapitate ligament arises from a rough surface at the base of the ulnar styloid (i.e., the basistyloid fovea) and courses distally to attach onto the neck of the capitate. The ulnotriquetral and ulnolunate ligaments are deep fascicles that emerge from the palmar edge of the TFCC and run toward their distal insertions onto the anterior aspects of the lunate and triquetrum. The distal insertions of the ulnocapitate ligament blend with those of the triquetrocapitate ligament before inserting onto the capitate. That combined ligament, together with the RSC ligament, form the “distal V” ligament, also known as arcuate ligament.
There is only one dorsal extrinsic ligament binding the radius to the carpus—the dorsal radiotriquetral ligament, also known as the dorsal radiocarpal ligament. It is a wide ligament that emerges from the dorsal edge of the distal radius and courses obliquely toward its distal insertion onto the dorsal ridge of the triquetrum. This ligament may have fibers inserted onto the lunate, but rarely onto the scaphoid. There are no dorsal ligaments between the ulna and the carpus.
Because the wrist is not a hinge joint, there are no longitudinal ligaments between the radial and ulnar styloid processes and the medial and lateral corners of the carpal condyle. The absence of collateral ligaments is substituted for by the dynamic actions of the extensor carpi ulnaris (ECU) medially and the abductor pollicis longus (APL) laterally. Although long considered an extrinsic palmar ligament, the radioscapholunate (RSL) ligament of Testut-Kuentz is not a true ligament, but a bundle of loose connective tissue containing vessels that supply the palmar corner of the proximal pole of the scaphoid.
Intrinsic Ligaments
There are two types of intrinsic carpal ligaments: transverse intercarpal and midcarpal. The former interconnect bones from the same carpal row, while the latter link bones across the midcarpal joint.
The SL joint is stabilized by two distinct transverse intercarpal ligaments (palmar and dorsal) and the proximal fibrocartilaginous membrane uniting both of them. The latter follows the arc of the proximal edges of the two bones from dorsal to palmar, separating the radiocarpal and midcarpal joint spaces. The dorsal SL ligament is located in the depth of the dorsal capsule and connects the dorsal–distal corners of the scaphoid and lunate bones. It is formed by a thick collection of fibers, slightly obliquely oriented, and plays a key role in scaphoid stability. Its anterior counterpart, the palmar SL ligament, has longer, more obliquely oriented fibers, which allow substantial flexion and extension of the scaphoid relative to the lunate. The dorsal SL ligament has the greatest yield strength, 260 newtons (N) on average, followed by the palmar SL ligament (118 N) and the proximal membrane (63 N). The proximal membrane is formed by fibrocartilaginous tissue that is often perforated in older individuals ( Figure 13.4 ).

The LTq joint also has two transverse intercarpal ligaments (palmar and dorsal) connecting the palmar and dorsal aspects of the two bones, and a fibrocartilaginous membrane closing the joint proximally. In contrast to the SL ligaments, the palmar LTq ligament is thicker and stronger than its dorsal counterpart (average yield strengths: 301 N and 121 N) with the proximal portion being the weakest (64 N). Unless perforated by age or injury, this proximal membrane prevents communication between the radiocarpal and midcarpal joint spaces. The fibers of the two LTq ligaments are under greater tension through all ranges of motion than the fibers of the SL ligaments. The most distal fibers of palmar and dorsal LTq ligaments are often connected to the distal fibers of the SL ligaments, forming the palmar and dorsal scaphotriquetral ligaments. These structures, as well as the interdigitating fibers of the dorsal intercarpal ligament, contribute to the stability of the LC joint by increasing the depth of the midcarpal fossa.
The distal carpal row bones are strongly bound to each other by stout transverse intercarpal ligaments (i.e., dorsal, palmar, intraarticular). They are essential to ensure the rigidity of the transverse carpal arch and to protect the carpal tunnel contents.
The midcarpal joint is crossed by three palmar ligaments (i.e., triquetrohamate [TqH], triquetrocapitate [TqC], and scaphocapitate), one dorsolateral STT ligament, and one dorsal intercarpal ligament. The TqH and TqC ligaments are thick structures, variable in size and shape, that play an important role in the stabilization of the midcarpal joint. Their anatomy varies according to the type of lunate (I or II). The TqC ligament is also known as the ulnar arm of the arcuate ligament. Laterally, the scaphoid tuberosity is linked to the distal row by the anteromedial SC ligament and the dorsolateral STT ligament. These two ligaments behave as collateral ligaments for the STT joint.
The only ligament crossing the dorsum of the midcarpal joint is the dorsal intercarpal ligament. On the ulnar side, it arises from the dorsal ridge of the triquetrum and courses transversely along the dorsal edges of the proximal row bones, distal to the scaphotriquetral ligament. At the level of the scaphoid, this ligament fans out to insert onto the dorsal rim of the scaphoid, the trapezium, and the trapezoid. There are no ligaments, palmar or dorsal, between the lunate and capitate.
Wrist Biomechanics
The wrist is a highly mobile, composite articulation that links the two forearm bones and the hand, characterized by its ability to sustain substantial amounts of load in all positions. To achieve this, there must be appropriate interaction between wrist motor tendons, joint surfaces, and soft tissue constraints. What follows is a brief description of how the wrist moves (i.e., kinematics) and how it withstands loads without yielding (i.e., kinetics).
Carpal Kinematics
The wrist can be moved passively by an external force, or actively by contracting muscles with a tendon crossing the joint. None of these tendons inserts onto the proximal carpal row; all of them insert distal to the midcarpal joint. Consequently, when these muscles contract, the distal carpal row is the first to move. The bones of the proximal row do not start rotating until the midcarpal ligaments become taut and pull them into motion. In other words, around the neutral position, the only joint that moves is the midcarpal articulation.
Flexion–Extension
In the sagittal plane, the scaphoid rotates more than the lunate, owing to differences in the shape of their proximal and distal articulating surfaces and surrounding ligamentous anatomy ( Figure 13.5 ). From neutral-to-maximal wrist flexion, the scaphoid and lunate contribute 70% and 46%, respectively, to the total range. Similarly, during maximal wrist extension, the scaphoid and lunate contribute 72% and 42%, respectively. The shape of the two bones also deserves a comment: the scaphoid is obliquely oriented relative to the longitudinal axis of the forearm; because of that, it is easier for it to flex than to extend.

The lunate, by contrast, has an inherent tendency to extend as it is narrower dorsally than palmarly. With this in mind, it is easy to understand why the contribution of the radiocarpal and midcarpal joints to the total range of wrist flexion–extension is different in the central column (55% of the total range takes place in the midcarpal joint) than in the radial column (70% of motion happens at the RS joint).
Radioulnar Inclination
When the wrist moves along the frontal (i.e., coronal) plane, both the proximal and distal rows go into radial and ulnar inclination, but the proximal carpal row also flexes and extends. In radial inclination, the trapezium and trapezoid approximate to the radius and therefore the scaphoid has to flex because the space is reduced, and its longitudinal axis becomes almost perpendicular to that of the radius. The lunate and triquetrum also flex, although to a lesser degree.
When the wrist goes into ulnar inclination the scaphoid extends, pulled by the trapezium and trapezoid, with its longitudinal axis becoming practically collinear to that of the radius. The lunate and triquetrum also extend during ulnar inclination, although to a lesser degree. For this reason, if the wrist is ulnarly inclined when a plain radiograph or a sagittal tomogram is obtained, one may erroneously think that the extended position of the lunate is secondary to misalignment.
Dart-Thrower’s Motion
The orthogonal (i.e., frontal and sagittal) planes of motion are seldom utilized in activities of daily living. The most commonly used plane of motion goes from extension and radial inclination to flexion and ulnar inclination. Fisk described this plane as “when someone is casting a fly or throwing a dart”; thus, hand surgeons use the term “dart-thrower’s” (DT) motion.
When the wrist is in radial inclination, the scaphoid and lunate are flexed. If the wrist is then brought into extension, the lunate recovers its initial alignment, as when the wrist was in neutral. When the wrist goes into ulnar inclination, the scaphoid and lunate extend, but they regain that neutral position when the wrist is flexed while in ulnar inclination. In other words, when the wrist moves along an oblique plane going from extension and radial inclination to neutral, and finally into flexion and ulnar inclination, the scaphoid and lunate remain in neutral position. Indeed, during DT motion, most wrist mobility takes place at the midcarpal joint.
The obliquity of the DT plane of motion is unique to each individual, ranging from 37 to 59 degrees from the sagittal plane, according to various authors. This oblique plane of wrist motion, generated by the pull of two wrist extensors—extensor carpi radialis longus (ECRL) and extensor carpi radialis brevis (ECRB)—and one wrist flexor, the FCU, is one of the most frequently used in activities of daily living.
Reversed Dart-Thrower’s Motion
The opposite rotation, from extension–ulnar inclination to flexion–radial inclination has been referred to as the “reversed dart-thrower’s” motion. It is a rotation mediated by the FCR on the volar side and the ECU on the dorsal side of the wrist. As opposed to what happens during DT rotation, the contribution of the midcarpal joint to the reversed dart-thrower’s type of rotation is minimal: only the radiocarpal joint is mobilized. Based on this, the wrist can be compared to a universal (i.e., cardan) type of articulation with two axes of rotation: one for the midcarpal joint and another for the radiocarpal joint. The midcarpal dart-throwing axis and the radiocarpal reversed DT axis do not coincide in space: the first would be located at about the center of the head of the capitate, while the second would be more proximally located at about the LC joint.
Needless to say, this way of describing wrist motion is an oversimplification. In reality, each bone has its own center of rotation that changes in location and orientation as the wrist moves. There are substantial synergies between the bones of each row that make this simplified model useful for the clinician, however. In fact, this model is the first to provide a convincing explanation of how the wrist is able to transmit pronosupination torques, from distal-to-proximal or vice versa, in all wrist positions, without losing stability ( Figure 13.6 ).

Intracarpal Pronosupination
Most axial rotation of the hand takes place in the forearm, with the radius rotating around a slightly oblique longitudinal axis running from the center of the head of the radius to the center of the head of the ulna. However, some pronation and supination also take place in the wrist, mainly at the midcarpal joint. Passive intracarpal pronation and supination are greater than active rotation.
The mechanism of active intracarpal pronosupination has recently been elucidated and can be explained as follows. Distal to the extensor retinaculum, most wrist motor tendons change direction, taking an oblique path to their distal insertion. The ECU tendon, for instance, from a dorsal position at the level of the ulnar head, courses obliquely toward its distal insertion onto the volar–ulnar corner of the fifth metacarpal. The ECRL and the APL also have an oblique course but in the opposite direction, toward the lateral border of the wrist.
All of them are dorsal at the radiocarpal level but diverge distally toward their ulnar or radial insertions. If the wrist is in a neutral position, isolated isometric contraction of any of these muscles will generate a pronation or supination moment to the distal row: ECU muscle contraction will cause intracarpal pronation, whereas the ECRL and APL muscles will induce a supination moment to the distal row.
Carpal Kinetics
Hand function generates a considerable amount of force that is transmitted across the carpometacarpal (CMC) joints into the carpus. At the distal row, loads are distributed among the midcarpal joints with the SC and LC joints transmitting ~50%, the STT joint ~30%, and the TqH joint ~20%. The fact that most synovial cysts emerge from the SL interval proves this to be a particularly overloaded area; indeed, ganglions tend to appear on the surface of chronically strained ligaments.
Once in the proximal row, 50% of the total load is transmitted across the RS joint (scaphoid fossa), 35% across the RL joint (lunate fossa), and 15% across the TFCC into the ulna. The average pressure values within the radiocarpal joint may be as high as 5.6 MPa, which is approximately 0.5 N/mm 2 or 810 lb/in 2 . These figures vary substantially with wrist position. The lunate fossa is increasingly loaded with ulnar inclination, the scaphoid fossa with radial inclination. When the wrist is in the “position of function” (i.e., slightly extended and radially inclined), the lunate tends to carry more load than the scaphoid.
When axially loaded, the carpal bones become displaced following specific patterns. The magnitude and direction of displacement depend on the shape of the articular surfaces, the orientation and point of application of the incoming loads, and the status of the various soft tissue constraints (i.e., capsule, ligaments, muscles).
A wrist is stable when it is able to sustain physiological loads in all positions without experiencing abnormal carpal displacement. When a stable wrist is loaded, the carpal bones do not displace beyond normal limits. For this to happen, the articular surfaces must be normally oriented and congruous, the ligaments must be all present and active; and the forces generated by the muscles acting on the carpal bones must be balanced.
The Role of Ligaments in Carpal Stabilization
The proximal carpal row, truly an intercalated segment between the radius and distal carpal row, is inherently unstable. If not for the capsule, ligaments, and muscles, the three bones would collapse when compressed by the distal carpal row against the radius. The obliquely oriented scaphoid would rotate into flexion and pronation, while the wedge-shaped (thinner dorsally than palmarly) lunate and triquetrum would collapse into extension and supination ( Figure 13.7 ).

In theory, if the SL ligaments were all intact, the flexion tendency of the scaphoid would be counteracted by the opposite tendency of the loaded lunate and triquetrum to rotate into extension, and stability would be achieved. In practice, these ligaments cannot do that alone. The tensile forces involved in most hand activities are far too high for these ligaments to be the only wrist stabilizers. To achieve stability, ligaments need to be protected by muscles.
To ensure a timely and protective muscle response, the carpal ligaments contain mechanoreceptors providing the proprioceptive information required by the sensorimotor system to activate that response. In other words, the ligaments provide the first line of defense against instability, but the muscles are the ultimate stabilizers.
The Role of Muscles in Carpal Stabilization
Until recently, muscles were considered to have a negative influence on carpal stability. Instability was assumed to be a ligament insufficiency problem made worse by muscle contraction. Now we know that the assumption is wrong: muscles play an important stabilizing role ( Figure 13.8 ).

The mechanisms of muscle stabilization of the wrist are based on the previously mentioned ability of some muscles to pronate or supinate the distal carpal row. When the intracarpal supinators (ECRL or APL) contract, the trapezium displaces dorsally, thus tightening the STT ligament. If the STT ligament is taut, the scaphoid cannot collapse into flexion and pronation. The intracarpal supinators, therefore, protect against excessive flexion–pronation of the scaphoid (see ). In contrast, when the ECU contracts, the distal row pronates, tightening the TqH ligament and preventing the proximal row from collapsing into flexion. Thus, the supinators are useful in the treatment of scaphoid instabilities, and the pronators in the treatment of most ulnarside carpal instabilities. Again, muscles are the ultimate carpal stabilizers.
Wrist Disorders
Wrist function may be altered in a variety of ways. A change in the shape of the articulating bones, a rupture or insufficiency of specific ligaments, and/or a loss of the stabilizing effect of specific muscles can explain most carpal disorders. The resulting malfunction may be present at birth (i.e., congenital disorders); may appear slowly as a result of abnormal growth (i.e., developmental disorders); or be the consequence of violent trauma, repetitive stress, or a joint damaging disease (i.e., acquired disorder). This chapter discusses the assessment and treatment of most acquired disorders of the wrist.
With time, most wrist disorders evolve into carpal misalignment. In theory, the most misaligned wrists should all be unstable. In practice, this is not always true. Instability is a multifactorial phenomenon that may or may not be associated with an alteration of the carpal alignment. The misalignment of the so-called “adaptive wrists”, for instance, is not a sign of ligament-related instability, but an adaptation of a normal carpus to compensate for an extracarpal bone deformity. Another example is the constitutional hyperlax wrist: It may appear misaligned, yet it frequently remains asymptomatic, able to handle most activities of daily living, and seldom requires treatment. Chronic unreduced perilunate dislocations are also examples of malaligned, but not unstable wrists. Certainly, if a dislocation is left unreduced, the joint will be unstable for a while until it undergoes a process of arthrofibrosis and becomes stiff, and stiffness is the opposite of being unstable. Indeed, a normally aligned carpus may behave as an unstable articulation; and vice versa, a grossly misaligned wrist may behave as if it was a completely stable structure.
With these considerations in mind, this chapter differentiates the unstable carpus secondary to intracarpal pathology from the misaligned, yet normal carpus secondary to extracarpal pathology, and from the misaligned but stiff wrist secondary to posttraumatic arthrofibrosis. What follows is a review of some aspects that are common for these three clinical conditions.
Pathomechanics
Carpal derangements may result from a direct or an indirect mechanism of injury. In direct mechanisms, the destabilizing force is applied directly to the dislocating bone, whereas indirect mechanisms involve a force applied at a distance from the injured joint. A typical direct mechanism occurs when the wrist is crushed by a power press or a wringer-type machine. If the dislocating force is applied over a large surface area of the hand, a carpal derangement may follow. In contrast, if the dislocating force is exerted over a small surface area, a localized fracture–dislocation may occur.
Wrist extension is the primary component of injuries causing distal radial fractures, scaphoid fractures, or perilunate dislocations. Differences in bone stock, the direction and magnitude of the deforming forces, and/or the position of the wrist at the time of incident may explain which type of injury occurs.
Perilunate dislocations are complex carpal derangements, usually the result of a combination of loaded hyperextension and intracarpal axial rotation. A typical case would be a head-on motorbike collision, where the hand gets pinned to the handlebar while the body is violently projected forward. The wrist, in such instances, becomes the pivot point about which the body rotates. When this happens, carpal destabilization undergoes a quite consistent four-stage pattern called “progressive perilunar instability,” described by Mayfield et al. The stages shown in Figure 13.9 are described in the following subsections.

Stage I: SL Dissociation or Scaphoid Fracture
When the wrist is violently twisted into extension and intracarpal supination, if the STT and SC ligaments are intact, the scaphoid extends and supinates, dragged by the trapezium. The lunate, in contrast, stays behind, constrained by the two long and short RL ligaments. With this, an increasing SL torque is created. When this torque reaches a certain level, progressive tearing of the SL intercarpal membrane and ligaments tends to occur. Usually, this happens from palmar-to-dorsal.
The end stage would be a complete SL dissociation. If the same process happens when the wrist is radially inclined (i.e., when the proximal pole of the scaphoid is powerfully constrained by the RSC ligament), instead of SL dissociation, an scaphoid fracture is likely to occur. More rarely, the lunate may suffer a coronal fracture, the volar portion of it remaining attached to the radius while the dorsal fragment follows the scaphoid and distal row in their dorsal displacement.
Stage II: Perilunate Dislocation
Once the SL joint is disrupted, if the destabilizing force continues, the capitate may leave the lunate concavity, leading to a dorsal perilunate dislocation. If instead of SL dissociation there is a scaphoid fracture, the wrist will suffer a transscaphoperilunate dislocation. Less commonly, the capitate may undergo a fracture. In such cases, only the body of the capitate dislocates dorsally with the rest of the distal row. The head of the capitate stays behind, sometimes completely rotated. In most instances, the displacement of the distal row is associated with a mid-substance stretching injury of the RSC ligament.
Stage III: LTq Disruption or Triquetrum Fracture
As the distal row dislocates dorsally, the TqH and TqC ligaments become extremely taut, generating an extension/dorsal translation vector to the triquetrum. This may result either in the separation of the triquetrum from the lunate, owing to tearing of the LTq ligaments, or a sagittal fracture of the triquetrum.
Stage IV: Lunate Dislocation
When all perilunate ligaments are torn, only the dorsal capsule and palmar RL ligaments can hold the lunate in place. In such circumstances, the dorsally displaced capitate, propelled by the forearm muscles, may enter the radiocarpal joint and push the lunate palmarly, out of the lunate fossa. This results in an anterior dislocation of the lunate. Such a dislocation is often associated with a variable degree of lunate flexion into the carpal tunnel.
Depending on the amount of lunate rotation, lunate dislocation has been subdivided into three categories: type I, in which the lunate exhibits greater than 90 degrees of flexion deformity; type II, in which the lunate is flexed >90 degrees around an intact, undisrupted, palmar RL capsule and ligament; and type III, which is a complete palmar enucleation of the lunate with rupture of the palmar capsule.
There are cases where the carpal derangement starts at the ulnar side and proceeds radially around the lunate. Usually, this happens as a result of a backward fall on the outstretched hand with the upper limb externally rotated. In such circumstances, the disrupting load is applied to the hypothenar area, the triquetrum being forced by the pisiform to displace dorsally relative to the lunate. Since the lunate cannot displace in the same direction because it is constrained by the radius, an increasing shear stress develops between the two bones. In such cases, complete rupture of the LTq ligaments would be stage I of a reversed perilunate destabilization pattern of the wrist, ulnocarpal ligament rupture plus LC dislocation would be stage II, and SL dissociation would be stage III. This alternative mechanism has recently been confirmed in the laboratory.
Diagnosis of Wrist Disorders
Wrist disorders present in a great variety of ways. From the patient who has suffered a violent trauma such as a fall from a height or a crush injury to the wrist, presenting with a major fracture or dislocation of the upper limb, to the patient with a symptomatic malfunctioning wrist without a clear recollection of what caused the problem. In the former, the diagnosis of a major carpal derangement may be obvious; however, in the latter, identification of the true nature of the problem may not be easy. This section discusses clinical and radiographic assessments of both acute and chronic dysfunctions. Supplemental information on the particular features of each type can be found in Rhee et al and Sauvé et al.
Clinical Examination
Physical examination always needs to be preceded by a thorough investigation of the patient’s medical history, with special emphasis on the mechanism of injury. The patient should be asked details about the location, duration, and characteristics of any pain including aggravating and relieving factors, and previous treatments, if any. With chronic problems, it is also important to inquire about the patient’s jobs and hobbies, and whether there has been exposure to repetitive stress, vibrating tools, or potentially dangerous instruments.
The next step is inspection. Except in cases with an open dislocation, the external appearance of most wrist dislocations may not be dramatic. Swelling is generally moderate, and bone displacements may be evident only if the patient is seen immediately after experiencing the trauma. If there has been a delay since the accident, swelling may have increased significantly, making visualization of the displaced bones more difficult. When present, skin abrasions, contusions, or ecchymosed areas may be helpful in determining the mechanism of injury and the potential areas of damage.
Palpation for areas of maximal tenderness is one of the most useful tools in the diagnosis of wrist pathology, especially in patients with chronic dysfunctions. In acute dislocations, because of extensive soft tissue damage, tenderness is seldom elicited at specific points, but rather in a diffuse manner. Nonetheless, palpation should always be performed in a methodical way.
Acutely, range of motion is usually limited by pain, whereas it may be reduced or normal in more chronic cases. In the latter, passive mobilization of the joints is valuable not only in determining the presence of abnormal motion or crepitus but also in reproducing the patient’s pain. Grip and pinch strength also need to be measured for chronic problems to uncover underlying pathology. Strength may be reduced due to muscle atrophy or by pain inhibition. A local injection of anesthetic within the affected joint may normalize the dynamometer readings in the latter.
A careful assessment of neurovascular status is imperative, with particular attention being paid to the median and ulnar nerves, which may be injured by direct contusion at the moment of impact, by compression from displaced bones, or by swelling in the carpal canal.
Radiological Examination
Routine Radiograph Views.
The initial radiographic examination of a patient with a suspected carpal injury should include four images of the wrist. If some of these are omitted, or of inadequate quality, the likelihood of missing important information increases.
Posteroanterior (Palm Down) Projection.
The posteroanterior (PA) view should be obtained with the hand placed flat over the radiograph film, the shoulder abducted 90 degrees, the elbow flexed 90 degrees, and the forearm in neutral rotation. One can determine whether it is a true PA radiograph by checking that the ECU groove is projected radial to the axis of the ulnar styloid. In the PA view of a normal wrist, the proximal and distal outlines of the proximal row, as well as the proximal outline of the distal row (i.e., the so-called “Gilula’s lines”) are smooth, without breaks in their continuity ( Figure 13.10 ). Any step-off of these lines indicates abnormality at the site where the arc is broken.

Normally articulating bones have parallel opposing surfaces separated by 2 mm or less. Any overlap between well-profiled carpal bones definitely suggests an intercarpal abnormality. Joint diastasis should not be interpreted as a sign of joint disruption unless it is asymmetric. Contralateral wrist radiographs are mandatory for a ligament injury workup.
In the PA view of a wrist in neutral, the normal lunate has a trapezoidal configuration. It is possible to differentiate a flexed from an extended lunate based on the shape of its contour ( Figure 13.11 ). To do so, it is important to remember that, in the transverse plane, the lunate is wider palmarly than dorsally. Therefore, when abnormally extended, the lunate has an ovoid configuration due to the distal displacement of the wider anterior horn. Conversely, when abnormally flexed the lunate is triangular shaped, often with a half moon-like configuration, with its concavity facing toward the scaphoid.

Lateral Projection.
The lateral view must be taken with the arm adducted to the patient’s side, and with both the forearm and the wrist in neutral rotation. For a lateral projection to be correct, the axis of the third metacarpal needs to be parallel to that of the radius, and the palmar outline of the pisiform needs to be located between (and equidistant to) the palmar surfaces of the scaphoid tuberosity and the capitate head.
Scaphoid Projection.
The best projection to confirm a scaphoid fracture is a PA view centered on it, with the wrist in ulnar inclination and the fingers fully flexed.
Semipronated (Oblique) Projection.
A 45-degree semipronated oblique view profiling the anterolateral and posteromedial corners of the carpus is useful to investigate the presence of fractures of the dorsal ridge of the triquetrum and of the scaphoid tuberosity.
Additional Radiograph Views.
When routine radiographs do not confirm the suspected diagnosis, obtaining additional views is recommended. The following are the most commonly used projections for the wrist.
- •
Anteroposterior (palm up) view with a clenched fist: Alternatively, get radiographs with a longitudinal compression force applied to the wrist by an assistant, which may accentuate the gap that often appears in SL dissociation ( Figure 13.12 ). It is preferable to obtain this view without wrist extension or flexion. Correct positioning can be objectively evaluated by looking at the third CMC joint. When the wrist is in neutral, the joint’s surfaces are parallel.
FIGURE 13.12
Compression of the carpus by having the patient make a fist ( large arrow ) may accentuate the gap in complete scapholunate dissociation ( small arrows ).
- •
PA (palm down) view with 10 degrees of tube angulation from ulna toward radius: This view is ideal to assess the SL interval. Measurement of its separation (SL gap) is made at the midportion of the joint where its anatomy is more consistent. The spacing should be compared with the opposite wrist and with the surrounding carpal articulations.
- •
Clenched-pencil view: This is one of the best ways to demonstrate SL dissociation because it optimally profiles the joint while it is being separated with some grip force. It consists of a PA view of both wrists while the two hands are gripping one pencil tightly, with the index fingers apposed and the thumb metacarpals lying flat on the radiographic cassette. The technique provides comparison of both wrists at the same time.
- •
Oblique view at 20 degrees of pronation from the lateral position: This view is used to visualize the dorsum of the triquetrum, where avulsion fractures frequently occur, and to evaluate the distal tuberosity and waist of the scaphoid. It is ideal for fracture–subluxations of the fifth CMC joint.
- •
Oblique view at 30 degrees of supination from the lateral position: The pisotriquetral relationship is best seen with this view.
- •
Lateral view with the wrist radially inclined: The palmar outline of the hook of the hamate can be seen on a lateral view with the wrist radially inclined and the thumb in maximal anteposition. It places the hook of the hamate between the bases of the first and second metacarpals.
- •
Carpal tunnel view: By profiling the carpal concavity of the wrist, a clearer view of the hook of the hamate, the pisiform, and the palmar ridge of the triquetrum can be obtained. In patients with acute injuries, however, pain produced by extending the wrist may not allow the radiograph to be taken.
- •
Static motion views: For patients suspected of having carpal instability, a routine “motion” series may be needed. It should include PA and anteroposterior views in radial and ulnar inclination, in addition to lateral views in extension and flexion.
Carpal Bone Alignment Measurement.
Carpal misalignment has traditionally been determined by measuring specific distances and angles on PA or lateral radiographs. When interpreting these data, however, one must be aware that the normal ranges for these parameters are quite diverse. Also, reproducibility is low and small errors in rotational positioning of the hand at the time of radiograph exposure may result in substantial variation in angle determination. The following are the most frequently used measurements to define carpal bone alignment ( Figure 13.13 ).
- •
LC angle: This is helpful to quantify midcarpal misalignment. The axis of the lunate is a line perpendicular to a line connecting the palmar and dorsal tips of it. The capitate axis is identified by connecting a point in the center of the convexity of its head to a point at the center of the third CMC joint. A normal LC angle should be 0 ± 15 degrees with the wrist in neutral.
- •
SL angle: This angle, formed by a line tangential to the proximal and distal convexities of the scaphoid’s palmar aspect and that of the lunate, has been quoted extensively in the literature as one of the major determinants of SL dissociation. Normal values range from 30 to 60 degrees (average, 47 degrees). Although angles greater than 80 degrees indicate SL ligament disruption, lower readings do not rule out this pathology. Values less than 30 degrees are not unusual for patients with STT joint osteoarthritis.
- •
RL angle: This gives objective evidence of the dorsal or palmar tilt of the lunate. The normal RL angle should be 0 ± 15 degrees.
- •
Ulnar variance: The relative lengths of the radius and ulna, or “ulnar variance,” and the possible effects of this parameter on various carpal disorders have long been investigated. Ulnar variance is usually measured on standard PA radiographs, although lateral radiographic projections also offer very accurate readings. The usual way to assess ulnar variance is to measure the distance between two lines perpendicular to the longitudinal axis of the radius. One is tangential to the most distal point of the ulnar dome, and the other is tangential to the distal articular surface of the lunate fossa. When the ulna is shorter than the radius, the ulnar variance is negative, and when longer, it is positive.
- •
Carpal height ratio: This is another parameter in the evaluation of carpal collapse. The term carpal height refers to the distance between the base of the third metacarpal and the distal articular surface of the radius measured along the proximal projection of the axis of the third metacarpal. The ratio (carpal height ÷ length of third metacarpal) was found to be 0.54 ± 0.03 in normal wrists. Because wrist radiographs often do not include the entire third metacarpal, some authors have proposed using the length of the capitate instead (i.e., carpal height ÷ capitate length), with a normal range of 1.57 ± 0.05 ( Figure 13.14 ).
FIGURE 13.14
Carpal height ratio is calculated by dividing the carpal height ( L2 ) by the length of the third metacarpal ( L1 ). The normal ratio is 0.54 ± 0.03.117.
- •
Ulnar translocation ratio: In some instability conditions, there is an ulnar shift of the carpal bones. The amount of translocation can be quantified using a variety of techniques. The more commonly used one measures the perpendicular distance from the center of the head of the capitate to a line from the radial styloid, which extends distally and parallel to the longitudinal axis of the radius. The carpal translocation ratio (calculated as the ratio of this distance to the length of the third metacarpal) in normal wrists is 0.28 ± 0.03. Other techniques use the axis of the ulna or the axis of the radius as a reference ( Figure 13.15 ). However, they may not be as accurate as the ulnar translocation ratio.
FIGURE 13.15
Ulnar translocation of the carpus can be quantified in different ways according to various authors. McMurtry and colleagues suggested using the axis of the ulna as a reference to determine whether there is an ulnar shift of the center of the capitate head. In normally positioned wrists, the distance b divided by the length of the third metacarpal ( L1 ) should equal 0.3 ± 0.003. According to Chamay and coworkers, a vertical line extending distally from the radial styloid offers a more reliable reference to measure the ulnar shift of the capitate. Normal values for the distance c divided by L1 are 0.28 ± 0.03. A similar method was described by Di Benedetto and colleagues by using the longitudinal axis of the radius as a reference. The distance divided by L1 should be 0.015 ± 0.024. The so-called “lunate (L)-uncovering index” has been suggested by Linn and colleagues as another method to determine the relative position of the lunate with respect to the radius. According to Schuind and associates, the ratio between the length of uncovered lunate ( f ) and the maximal transverse width of this bone ( f / g ) should equal 32.6 ± 11. To measure lunate translocation, Bouman and associates found it more reproducible to use the ratio e / d , which in normal wrists equals 0.87 ± 0.04. The last two methods are more likely to detect ulnar translocations of the lunate than the first three methods, at the expense of being strongly dependent on the wrist being precisely positioned in neutral. Even minor degrees of radial or ulnar inclination may significantly alter the results.

Other Diagnostic Tests
Computed Tomography.
Computed tomography (CT) scans are usually taken at 1- to 2-mm intervals along the axial, sagittal, and coronal planes, or any plane in which the structure of interest can be best visualized. CT scan images following the longitudinal axis of the scaphoid, at approximately 45 degrees to the long axis of the radius, are best at showing the amount of collapse in a “humpback” scaphoid deformity. CT is also useful in evaluating the union of fractures or arthrodeses, although in many instances the image can be altered by the presence of retained hardware.
CT has the added advantage of allowing computer manipulation to obtain three-dimensional (3D) images of the carpal bones, which help visualize the structure to be analyzed. When surgery is planned on a malunited scaphoid or a complex carpal dislocation, a 3D reconstruction provides excellent visual information about the amount and direction of the displacement ( Figure 13.16 ). However, it is important to note that all the information provided by such a reconstruction is already present in the original CT image.

The recent introduction of ultrafast CT scans has substantially reduced image-acquisition time to a fraction of a second. By observing a sequence of 3D representations of the wrist, one can get the impression of seeing it in motion (see ). This is called “dynamic 3D,” also known as “four dimensions–computed tomography” (4D-CT scan). Although promising, this technology needs to be used with caution owing to the higher dose of radiation involved.
Distraction Views.
In patients with acute fracture–dislocations, the four routine views described earlier may be difficult to interpret because of the overlapping of displaced carpal bones. In such cases, obtaining anteroposterior and lateral radiographs with the hand suspended in finger traps is recommended. Distraction views may reveal intraarticular fracture fragments or joint dissociations in the form of a step-off that cannot be seen on routine films.
Stress Views.
In some instances, obtaining radiographs while stressing the joints in various directions may help visualize the abnormality ( Figure 13.17 ). A commonly used technique consists of taking PA projections of the wrist while forcing it into maximal radial or ulnar inclination. Lateral views while applying a dorsal or palmar force to the distal carpal row (“drawer test”) are also helpful to identify midcarpal instabilities.

Lateral radiographs, while extending a fully flexed wrist against resistance (“resisted extension test”), may reveal dynamic dorsal subluxation of the proximal scaphoid. In this case, if the SL ligaments are disrupted, the lunate will remain in a neutral or extended posture, substantially increasing the previously measured SL angle.
Cineradiography.
Dynamic cineradiographic examination of the wrist provides considerable information for the evaluation of patients with altered kinematics (“clunking” wrists). Cineradiography routinely includes observation of active motion from a radial-to-ulnar inclination in PA views; flexion and extension in lateral views, and radial and ulnar inclination in lateral views. If the patient has a painful clunk, the true nature of the subluxation can be identified by observing the moving wrist under an image intensifier. Provocative stress maneuvers may be of help to document the location of maximal dysfunction. In a recent study, cineradiography has been found to have a sensitivity of 90%, a specificity of 97%, and a diagnostic accuracy of 93% in detecting SL dissociation.
Arthrography.
Although long considered the gold standard in the assessment of intercarpal derangements, wrist arthrography is now rarely performed as an isolated procedure. The technique was originally introduced based on the assumption that any flow of dye from the radiocarpal to the midcarpal space or vice versa was pathologic. Since then, it has been proven that asymptomatic degenerative tears of the proximal SL or LTq membranes are not unusual, especially in older adults.
Nonetheless, arthrography still has some potential, particularly in association with high-resolution tomography (i.e., arthroscan), to assess cartilage defects and ligament injuries. When injecting the joint, it is important to watch the pattern of dye flow because this facilitates detection and estimation of the size of communicating defects.
Magnetic Resonance Imaging.
Compared with arthroscopy, traditional MRI without dedicated wrist coils shows reduced sensitivity and specificity (63% and 86%, respectively) in the diagnosis of SL ligament injury. When intravenous contrast medium is injected, these parameters may slightly improve, and this technique is increasingly being used. Technical improvements and advanced wrist imaging algorithms enable accurate measurement of the articular cartilage thickness, the length and integrity of specific ligaments, as well as injuries of the TFCC.
Because of its superior soft tissue contrast, direct multiplanar acquisition, and lack of ionizing radiation, MRI is an effective means to evaluate the ligaments of the wrist. High-resolution noncontrast techniques have proven efficacious in the evaluation of the TFCC and intrinsic ligaments, but require a dedicated wrist coil and slice thickness of no more than 1 mm.
Ultrasonography.
This is gaining a well-deserved reputation in the assessment of both intrinsic and extrinsic wrist ligaments. High-frequency linear transducers have been shown to have great potential. Ultrasonography is considerably less expensive than MRI, is real time (permitting dynamic evaluation of kinematic instabilities), and does not require intraarticular injection of a contrast medium or the use of ionizing radiation. No doubt, in the near future, ultrasonography will be incorporated into clinical practice as an inexpensive and safe method to get most of the information required to make accurate treatment decisions.
Arthroscopy.
Wrist arthroscopy provides the technical capabilities to examine and treat intraarticular abnormalities without an arthrotomy. As well as allowing direct visualization of the articular surfaces, synovial tissues, and intercarpal ligaments, arthroscopy has proved to be a useful adjunct in the management of various acute and chronic wrist lesions. Arthroscopy is an important wrist surgery tool; indications for its use are mentioned throughout this chapter and reviewed in detail in Chapter 17 .
Carpal Instability
Definition
Injuries to the wrist that induce carpal misalignment have long been recognized in the medical literature. In 1968, Fisk was the first to use the term “carpal instability” to describe a zigzag pattern of carpal misalignment present in some fractured scaphoids. In 1972, a landmark article by Linscheid and coworkers helped define specific patterns of carpal malalignment secondary to carpal ligament injury. Unfortunately, an oversimplified interpretation of those publications propagated the incorrect view that all carpal misalignments are unstable. As emphasized earlier, the term instability cannot be used as a synonym for misalignment , and an alteration of carpal alignment is not always pathologically unstable.
From a mechanical point of view, stability can be defined as the ability of a joint to avoid subluxations or dislocations under physiologic loads in all wrist positions. Stability not only implies the capacity to transfer loads without yielding (i.e., kinetic stability) but also the ability to maintain smooth carpal rotations without sudden, unexpected changes in bone position and/or alignment (i.e., kinematic stability). However, it is important to emphasize from a mechanical point of view, that the presence of symptoms and carpal instability are not synonymous. In fact, asymptomatic instabilities are frequent and many do not require treatment.
Analysis of Carpal Instability
Carpal instability is a multifactorial phenomenon that is difficult to diagnose and treat unless there is a thorough understanding of all the factors contributing to each individual case. What follows is an analytical scheme developed by Larsen et al to help the clinician in the assessment and interpretation of patients with carpal instability ( Table 13.2 ). According to this scheme, any carpal instability can be characterized by investigating the following five features.
- •
Chronicity: Traditionally, ligament injuries have been classified depending on the time elapsed from injury to diagnosis into three categories: acute, subacute, and chronic. In acute injuries, diagnosed within a week, the ligament-healing potential is likely to be optimal. In subacute injuries, diagnosed between 1 and 6 weeks, the deformity is still easily reducible but the ligaments may have reduced healing potential because of retraction and/or necrosis. After 6 weeks, or in chronic cases, the possibility of achieving an acceptable reduction and primary ligament healing, although possible, is unlikely. The exception would be some ligament avulsions where the ligament is detached but not resorbed; it remains capable of being repaired with good healing potential beyond the time limit quoted for mid-substance ligament ruptures.
- •
Severity: Any carpal instability can be analyzed according to the severity of the resulting subluxation. If carpal misalignment appears only under high stress in specific wrist positions, the case is less severe than if it were permanently present. Based on this idea, three groups of instabilities exist: occult (partial ligament tears with no misalignment under stress), dynamic (complete ruptures exhibiting carpal misalignment only under certain loading conditions), and static (complete ruptures with permanent alteration of carpal alignment).
- •
Etiology: Although most instability problems are caused by trauma, certain diseases (e.g., inflammatory arthritis) may also be responsible for a similar constellation of radiographic findings. In traumatic cases, especially if diagnosed early, effective repair of the ruptured ligaments is possible. If ligament rupture results from rheumatoid arthritis, strong remaining ligament tissue is unlikely.
- •
Location: It is important to investigate the location of the most important dysfunctions (e.g., subluxation or joint gapping), which may or may not coincide with the location of the primary lesion. This is especially important in the nondissociative instabilities in which treatment depends on which joint (i.e., radiocarpal or midcarpal) is more unstable under stress. It is also important to consider whether the pathology affects only one or several joints.
- •
Direction: When present, the direction of the carpal misalignment is an important factor to consider; several patterns have been recognized ( Figure 13.18 ). The most common are: (1) DISI, when the lunate is regarded as an intercalated segment and appears abnormally extended relative to the radius and capitate; (2) VISI, when the lunate appears abnormally flexed; (3) ulnar translocation, when a portion of, or the entire proximal row, is (or can be) displaced ulnarly beyond normal limits; (4) radial translocation, when the proximal row can be passively displaced radially beyond normal, usually in the context of a radially malunited distal radius fracture; (5) dorsal translocation, when the carpal condyle, often as a result of a dorsally malunited fracture of the radius, is (or can be) displaced in a dorsal direction.
FIGURE 13.18
Views of the two major patterns of sagittal malalignment as described by Linscheid and coworkers. In dorsal intercalated segment instability (DISI) and volar intercalated segment instability (VISI; also known as PISI, for palmar) the lunate (L) and triquetrum (Tq) rotate abnormally in a dorsal or volar direction, respectively ( red arrows ).
Category I: Chronicity | Category II: Constancy | Category III: Etiology | Category IV: Location | Category V: Direction |
---|---|---|---|---|
Acute, >1 week (maximum primary healing potential) Subacute, 1–6 weeks (some healing potential) Chronic, >6 weeks (little healing potential) | Occult Dynamic Static reducible Static irreducible | Congenital Traumatic Inflammatory Neoplastic Iatrogenic Miscellaneous | Radiocarpal Proximal intercarpal Midcarpal Distal intercarpal CMC Specific bones | VISI rotation DISI rotation Ulnar translation Dorsal translation Other |
Classification of Carpal Instability
Classifying the great variety of conditions that can cause carpal instability depends in large part on the findings from the preceding analysis. Many classification systems exist based on the location of the predominant disorder, the direction of the abnormal alignment, and the severity of the misalignment itself. However, none of these systems is exhaustive enough to allow categorization of all types of carpal instability or simple enough to be easily remembered and used clinically. Despite its limitations, the Mayo Clinic classification is the most commonly used by the majority of wrist specialists and therefore, it is recommended.
Having clarified that neither adaptive misalignments nor all types of fracture–dislocations are necessarily unstable, there are three major instability patterns of the wrist. The first group includes the so-called “dissociative carpal instabilities,” also known as carpal instability dissociative (CID), in which the predominant dysfunction (i.e., ligament rupture or insufficiency) occurs between bones of the same carpal row. Most CIDs occur between the scaphoid and lunate, and less often between the lunate and triquetrum. Dissociative instabilities between the bones of the distal carpal row are rare, usually the result of a violent dorsopalmar crushing mechanism.
The second group includes the nondissociative carpal instabilities, also known as carpal instability nondissociative (CIND). This group includes radiocarpal, midcarpal, and combined radiocarpal–midcarpal instabilities. If the entire carpus is malaligned (translocated) with respect to the radius in any direction, this is radiocarpal instability. If the proximal carpal row as a unit collapses and becomes unstable, either in flexion or extension, this is a combined radiocarpal–midcarpal instability, a condition that is also known as nondissociative instability of the proximal carpal row. Less frequently, there are cases where there is only a dysfunction at the midcarpal level, the radiocarpal joint being normal. Only these cases can be called true midcarpal instabilities.
The third group of instabilities is formed by the so-called “carpal instability complex” (CIC), where there are features of both CID and CIND types. Carpal dislocations, if treated inadequately, may generate complex (i.e., CIC) patterns of instability where the dysfunction affects both the radiocarpal and the proximal intercarpal joint. With time, however, the untreated or suboptimally reduced carpal derangement may evolve into a stiff misaligned joint. When this happens, the wrist may no longer be considered unstable, but stiff, and stiffness is the opposite of instability. This is why, in this edition, carpal dislocations are discussed in a section different from that of true carpal instabilities.
Dissociative Carpal Instability
In this group of instabilities, the misalignment occurs between two adjacent bones of the proximal carpal row, such as between the scaphoid and lunate or between the lunate and triquetrum. Dissociative instabilities seldom occur between the bones of the distal carpal row, as there is little mobility between them.
Scapholunate Dissociation.
Although this condition was recognized in the early 20th century, it was not until 1972 that the clinical features of SL dissociation were described by Linscheid and associates. SL dissociation is the most frequent carpal instability and can appear either as an isolated injury or in association with other local injuries (e.g., distal radial fractures, perilunate injuries, or displaced scaphoid fractures).
Pathomechanics of SL Dissociation.
A fall on the outstretched hand with the wrist in extension and ulnar inclination and associated with midcarpal supination may cause a wide spectrum of injuries, from minor SL sprains to complete perilunar dislocations. Although apparently different, these clinical conditions are stages of the same progressive perilunar destabilization process described earlier.
Different authors have investigated the kinematic and kinetic consequences of having a complete SL dissociation. In the cadaver, if only the palmar SL ligament and the proximal membrane are sectioned, minor kinematic alterations may appear. The SL joint, however, does not show an acutely detectable gap, not even under stress (occult instability). Scapholunate kinematics is altered, however, and heavy mechanical activity may cause symptoms. When painful, this may be due to the presence of joint synovitis and may improve with rest and antiinflammatory agents.
Complete sectioning of the SL ligaments results in substantial alterations of wrist kinematics and force transmission parameters, but acutely does not result in carpal misalignment. Immediate carpal misalignment only occurs if there is concomitant rupture of the secondary scaphoid stabilizers; that is, the palmar RSC, the palmar SC, and the anterolateral STT ligament. These ligaments may gradually attenuate with time and lead to secondary malrotation and carpal collapse. In these cases, the dissociated lunate and triquetrum rotate into extension under load. The scaphoid and the lunate separate in the coronal plane and adopt opposite deformities. The scaphoid flexes around the RSC ligament, while pronating and translocating toward the radial side. As a result of the scaphoid collapsing into flexion and pronation and the lunate and triquetrum into extension, the distal row is forced to rotate into pronation ( Figure 13.19 ).


It seems evident that axial loads will increase the normal flexed stance of the scaphoid. However, there is controversy regarding the mechanisms causing the lunate to go into extension. According to Kauer, the unconstrained lunate has a natural tendency toward displacing into extension owing to its configuration (with the dorsal horn being narrower than its palmar counterpart), but also to the fact that it is set onto a radial surface that is volarly inclined. Other authors believe that the lunate, when free from the constraints of the scaphoid, tends to follow the triquetrum toward extension under the influence of the helicoid TqH joint surfaces.
When the SL joint is completely dissociated, with the proximal pole of the scaphoid subluxed dorsoradially, the forces crossing the wrist cannot be distributed normally. An increased compressive and shear stress appears on the dorsal and lateral aspect of the radioscaphoid fossa, a situation that Watson and associates compared to two spoons sitting on top of each other with the handles out of alignment. Such peripheral contact may explain the frequent development of degenerative changes at the dorsolateral corner of the RS fossa. The lunate extends but remains stable with the radius because both opposing articular surfaces have the same radius of curvature. This explains why the RL joint is seldom affected by the degenerative process.
The term scaphoid–lunate advanced collapse (SLAC) has been proposed by Watson and associates to define the progression of degenerative changes secondary to SL dissociation. Although only three stages were initially proposed, a recent investigation by Lluch identified five stages: stage I, radioscaphoid; stage II, scaphocapitate; stage III, lunocapitate; stage IV, triquetrohamate; and stage V, radiolunate. In stage I, the earliest osteoarthritic changes will differ among SLAC and scaphoid nonunion advanced collapse (SNAC) wrists. In SLAC wrists, osteoarthritis includes the proximal scaphoid and the dorsal rim of the radius. In SNAC wrists, osteoarthritis develops between the distal scaphoid and the radial styloid, while the proximal fragment of the scaphoid remains free of pathology.
Diagnosis of SL Dissociation.
A history of a fall on an outstretched hand should warn the clinician about the possibility of an SL injury, sometimes masked by fractures of the scaphoid or the distal radius. SL dissociation is frequently missed at presentation, especially in isolated partial lesions, or when it is masked by other, more obvious injuries. When SL dissociation is static or results from a perilunate dislocation, the diagnosis is more obvious. According to Geissler and colleagues, up to 30% of distal radial fractures are associated with variable degrees of carpal ligament disruption. Aside from wrist trauma, iatrogenic SL injury can occur with excessive capsular excision when removing dorsal ganglions, or after ligament attenuation from rheumatoid, metabolic, or septic arthritis. Although SL dissociation is rare in children, diagnosis is more problematic because it is not easy to clearly identify bone axes in an immature skeleton and performing a careful clinical examination is difficult.
In both children and adults, a high index of suspicion is needed to avoid missing this injury. The symptoms of SL dissociation vary markedly depending on the magnitude of the ligament rupture, the extent of the associated injuries, and the time elapsed since the accident. Poor grip strength, limited mobility, dorsoradial swelling, and point tenderness over the dorsal aspect of the SL interval are the most common findings. Pain is common and is usually aggravated by intense use of the hand. The patient may report a clunking sensation during wrist movement due to dislocation or reduction of a dorsally subluxed proximal scaphoid.
Physical examination.
On inspection, there may be minimal or no changes in the appearance of the wrist with SL instability. Even in the acute phase, swelling may be moderate. Palpation at the areas of maximal tenderness is useful in the diagnosis of wrist pathology, especially for patients with chronic SL instability. By flexing the wrist and palpating the dorsum of the capsule distal to the Lister tubercle, one can obtain important information about the SL joint. If sharp pain is elicited by pressing this area, the probability of either a recent injury or a chronic localized synovitis is high. Most of these patients also have tenderness in the anatomical snuffbox and over the palmar scaphoid tuberosity. In acute cases, range of motion is usually limited by pain, whereas it may be normal in chronic cases.
Scaphoid shift test.
Passive mobilization of an unstable SL joint is valuable not only in determining the presence of abnormal scaphoid subluxation but also in reproducing the patient’s pain. A positive scaphoid shift test, as described by Watson and colleagues, is said to be diagnostic of SL dissociation. The examiner places four fingers behind the radius, and the thumb on the scaphoid tuberosity (distal pole). The other hand is used to move the wrist passively from ulnar-to-radial inclination. In ulnar inclination, the scaphoid is extended and assumes a position more in line with the forearm. In radial inclination, the scaphoid is flexed. Pressure on the tuberosity while the wrist is moved from ulnar-to-radial inclination prevents the scaphoid from flexing. If the SL ligaments are completely ruptured or elongated, the proximal pole subluxes dorsally out of the radius, inducing pain in the dorsoradial aspect of the wrist ( Figure 13.20 ). When pressure is released, a typical clunking may occur, indicating self-reduction of the scaphoid over the dorsal rim of the radius.

When performing the scaphoid shift test, one should be aware of its low specificity. If the SL ligaments are intact, but there are other local problems inducing local synovitis (e.g., occult ganglion or dorsal RS impingement), this test may also provoke sharp pain, making it difficult to discern whether there is an abnormally subluxable proximal scaphoid. Alternatively, patients with generalized laxity may exhibit painless clunks during this maneuver, which most likely emanate from the midcarpal joint. Comparison of the two sides is important, although sometimes the opposite “asymptomatic” wrist has a painful scaphoid shift test as well. Experience with this test is necessary before it can be interpreted with confidence.
Resisted finger extension test.
The ability of the proximal pole of the scaphoid to carry a load without producing pain can be explored by asking the patient to extend the index and middle fingers fully against resistance with the wrist partially flexed. In the presence of an injury or insufficiency of the dorsal SL ligament, a sharp pain is elicited in the SL area, probably due to the presence of synovitis at the RS joint. This maneuver is very sensitive but not specific for this pathology.
SL Ballottement Test.
The lunate is firmly stabilized with the thumb and index finger of one hand, while the scaphoid, held with the other hand (i.e., thumb on the palmar tuberosity and index on the dorsal proximal pole) is displaced dorsally and palmarly. The test is positive when there is pain, crepitus, and excessive mobility of the scaphoid.
Radiological examination.
On standard radiographs, SL dissociation is suspected in the presence of one or more of the features described in the following subsections. Dynamic instabilities require special projections or loading conditions for these features to be observed.
Increased SL joint space.
The “Terry Thomas sign,” named by Frankel after the English film comedian’s dental diastema, is considered positive when the space between the scaphoid and lunate appears abnormally wide compared with the contralateral side ( Figure 13.21 ). The SL gap should be measured in the middle of the flat ulnar facet of the scaphoid. A unilateral gap greater than 5 mm is diagnostic of SL dissociation. If there is no history of a specific traumatic episode, and yet there is an obvious SL diastasis, one must consider either a constitutionally increased SL gap, usually bilateral, with or without hyperlax ligaments. Other less common causes include rheumatoid arthritis, gout, and calcium pyrophosphate deposition disease. To rule out the presence of an occult asymptomatic SL ligament injury, a comparative radiographic examination is critical ( Figure 13.22 ).

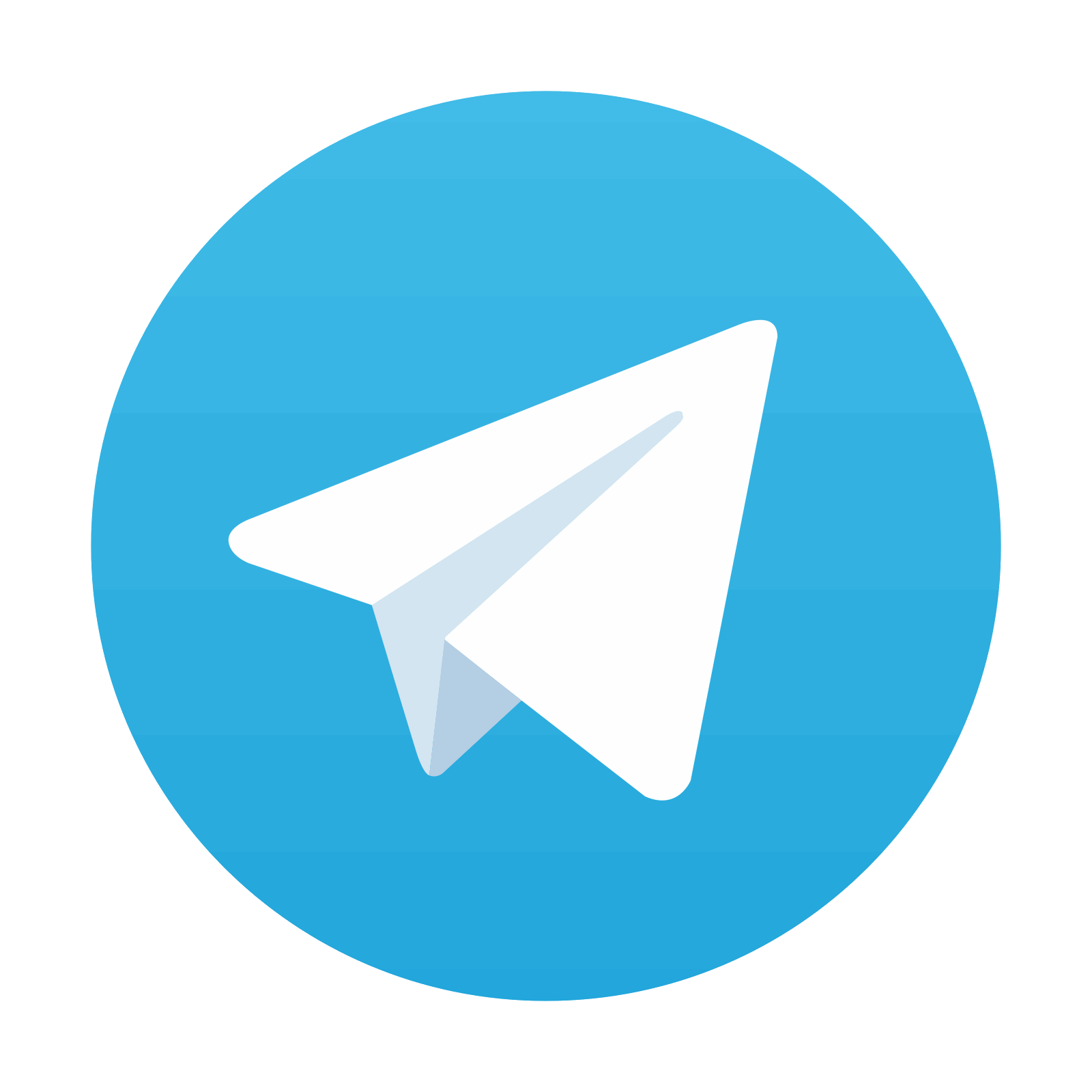
Stay updated, free articles. Join our Telegram channel

Full access? Get Clinical Tree
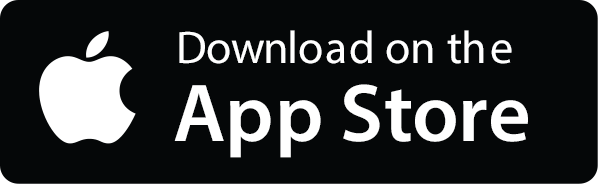
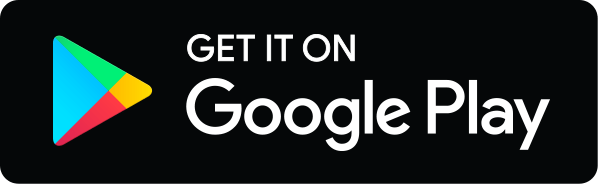
