Vitamin D physiology has gained more importance and publicity than any of its counterparts in the water- and fat-soluble vitamin groups combined. This is partly because vitamin D deficiency is still widely prevalent in the developed world and the beneficial effects are thought to extend beyond the regulation of calcium and phosphorus homeostasis alone. Vitamin D deficiency becomes even more important in the various stages of chronic kidney disease (CKD); CKD itself is also on the increase. How vitamin D physiology is altered in CKD and how the various treatment modalities can alter the morbidity and mortality associated with CKD is the topic of discussion for this article.
Vitamin D physiology has gained more importance and publicity than any of its counterparts in the water- and fat-soluble vitamin groups combined. This is partly because vitamin D deficiency is still widely prevalent in the developed world and the beneficial effects are thought to extend beyond the regulation of calcium and phosphorus homeostasis alone. Vitamin D deficiency becomes even more important in the various stages of chronic kidney disease (CKD); CKD itself is also on the increase. How vitamin D physiology is altered in CKD and how the various treatment modalities can alter the morbidity and mortality associated with CKD is the topic of discussion for this article. Chronic kidney disease, mineral and bone disorder (CKD-MBD) is the broad term used to describe the disease complex of hyperphosphatemia, secondary hyperparathyroidism, low levels of vitamin D, and their associated complications. The National Kidney Foundation, Kidney Disease Outcomes and Quality Initiative (KDOQI), and subsequently, Kidney Disease: Improving Global Outcomes (KDIGO), has endeavored to provide evidence-based clinical practice guidelines for various stages of CKD and has set certain target levels for the major factors involved in CKD-MBD. These factors include calcium, phosphorus, vitamin D, and parathyroid hormone (PTH). Whether achieving and maintaining these targets will provide any benefit for the overall survival of patients with kidney disease remains to be demonstrated.
Calcium
Calcium in present abundantly in the body. The skeleton acts as reservoir and buffer for calcium such that when a large oral load of calcium is ingested and absorbed, it gets buffered in the skeleton and when calcium is acutely needed, it is mobilized from the skeleton. Regulation of serum calcium is under complex control with short-term and long-term regulation. This regulatory control is governed by vitamin D metabolism, phosphorus metabolism, fibroblast growth factor 23 (FGF-23), and PTH. These regulators of calcium homeostasis are heavily dependent on each other, and there are continuous positive and negative feedback mechanisms. For various reasons that are discussed later, these regulatory mechanisms of calcium homeostasis become disrupted in CKD and result in the presentation of CKD-MBD.
CKD-MBD
Patients with kidney disease are at a many fold higher risk of bone fracture than their age-matched controls. Hyperphosphatemia and hyperparathyroidism are virtually universal in patients with advanced kidney disease. Vascular calcification can be debilitating and is strongly associated with the increased cardiovascular morbidity and mortality associated with CKD. Many patients in CKD stage 4 (estimated glomerular filtration rate [eGFR] 15–29) never seem to progress to CKD stage 5 (eGFR<15), and mortality from cardiovascular disease may be a contributing factor. CKD-MBD starts early, usually by CKD stage 3 (eGFR 30–59) and, for the most part, is a silent problem that only becomes manifest as CKD advances. Vitamin D deficiency is widely prevalent in the general population and even more so in patients with kidney disease.
CKD-MBD
Patients with kidney disease are at a many fold higher risk of bone fracture than their age-matched controls. Hyperphosphatemia and hyperparathyroidism are virtually universal in patients with advanced kidney disease. Vascular calcification can be debilitating and is strongly associated with the increased cardiovascular morbidity and mortality associated with CKD. Many patients in CKD stage 4 (estimated glomerular filtration rate [eGFR] 15–29) never seem to progress to CKD stage 5 (eGFR<15), and mortality from cardiovascular disease may be a contributing factor. CKD-MBD starts early, usually by CKD stage 3 (eGFR 30–59) and, for the most part, is a silent problem that only becomes manifest as CKD advances. Vitamin D deficiency is widely prevalent in the general population and even more so in patients with kidney disease.
Normal vitamin D physiology
Vitamin D is crucial for calcium and phosphorus homeostasis and the regulation of parathyroid function. In addition to obtaining vitamin D from diet, a significant amount is formed in the skin ( Fig. 1 ). Ultraviolet rays of the correct wavelength (UVB) in sunlight convert 7-dehydrocholesterol in the skin to previtamin D. This is then transported to the liver where it is hydroxylated at carbon 25 to form 25-hydroxyvitamin D [25(OH)D]. This is the main storage form of vitamin D in the human body and is the vitamin D metabolite that reflects the state of vitamin D nutrition. Almost all 25(OH)D is bound to circulating vitamin D–binding protein (DBP) and is then filtered by the kidney and taken up by the proximal convoluted tubule by an endocytic receptor, megalin. The 25(OH)D-DBP complex is degraded in proximal tubule lysosomes, releasing 25(OH)D, which then translocates to the mitochondria. In the mitochondria, 25(OH)D is converted to 1,25-dihydroxyvitamin D by the enzyme 1α-hydroxylase and returned to the circulation as the active form of vitamin D. New research has shown the presence of this enzyme in organs other than the kidney, such as pancreas, brain, lymph nodes, heart, gastrointestinal tract, adrenal glands, and prostate gland, such that 1,25-dihydroxyvitamin D may be made locally in these tissues. The biologic role of the extrarenal 1α-hydroxylase and the local effects of 1,25-dihydroxyvitamin D in extrarenal sites is the subject of ongoing studies.
The actions of 1,25-dihydroxyvitamin D are mediated by binding to the vitamin D receptor (VDR) and result in the alteration of the transcription of many genes in the various target organs.
Vitamin D metabolism in kidney disease
Kidney disease seems to be a risk factor for vitamin D deficiency and as many as 70% to 85% of patients with CKD are found to have low levels of 25(OH)D. Many factors may contribute, including lack of sunlight, loss of 25(OH)D-DBP with heavy proteinuria, diabetes, chronic illness, decreased production of previtamin D in the skin, and other unknown factors. Thus, the concentration of substrate for conversion to 1,25-dihydroxyvitamin D is decreased. This is further complicated by the fact that with decreases in glomerular filtration rate (GFR) and decreased renal mass, there is decreased delivery of substrate to the renal 1α-hydroxylase, which limits the ability of the diseased kidney to produce the active 1,25-dihydroxyvitamin D. In addition, as CKD develops, phosphate retention occurs decreasing 1α-hydroxylase activity, directly and leading to increases in the levels of FGF-23, which in turn, can directly decrease the activity of 1α-hydroxylase. FGF-23 is a recently discovered phosphaturic hormone that is regulated by dietary phosphate, serum phosphate, and 1,25-dihydroxyvitamin D. FGF-23 levels increase early in CKD, presumably in response to phosphate retention, in an effort to increase phosphate excretion in conjunction with increases in PTH. Although PTH stimulates the activity of 1α-hydroxylase, the suppressive effect of FGF-23 on 1α-hydroxylase activity seems to dominate in this clinical situation. In addition, it has been suggested that accumulation of N-terminally truncated PTH peptides of C-terminal PTH fragments may decrease 1α-hydroxylase. Thus, because of these abnormalities ( Fig. 2 ), it is not surprising that the levels of 1,25-dihydroxyvitamin D are reduced in CKD and progressively decline with advancing stages of CKD. In addition to decreased production of 1,25-dihydroxyvitamin D, there is also evidence for resistance to the actions of vitamin D as kidney disease progresses in that there may be decreased concentrations of the VDR, impaired binding of the 1,25-dihydroxyvitamin D binding to the VDR, and possibly impaired binding of the VDR complex to the vitamin D response elements in the nuclei. This altered vitamin D physiology in patients with renal disease contributes to subclinical or less commonly overt hypocalcemia and leads to the need for increased levels of PTH, which mobilizes minerals from the skeleton by stimulation of osteoclastic-mediated bone resorption. This process leads to the loss of lamellar bone and replacement with the woven and structurally weaker bone, increased propensity for fractures, and overall decreased quality of bone.
Phosphorus
Abnormal phosphorus metabolism is a major factor in CKD-MBD. Phosphate retention, as a consequence of decreased GFR, is thought to be a major factor in the pathogenesis of CKD-MBD. Phosphorus retention can lead to increased PTH directly and indirectly by increasing the levels of FGF-23, which, in turn, decreases the activity of 1α-hydroxylase. High levels of phosphorus have been shown to promote vascular calcification in animal models and are associated with vascular calcification in experiments in vitro.
Role of vitamin D treatment in CKD-MBD
Because of the major role of abnormal vitamin D metabolism in the disturbances of calcium and phosphorus homeostasis and in the pathogenesis of secondary hyperparathyroidism in the setting of CKD, use of vitamin D sterols is an important aspect of the therapy of patients with CKD. Current practice guidelines suggest evaluation for the presence of hyperparathyroidism early in the course of CKD, and if PTH values are elevated, vitamin D deficiency should be evaluated by measurement of 25(OH)D levels. If 25(OH)D levels are less than 30 ng/mL then vitamin D supplementation should be initiated. This is most often accomplished by the administration of ergocalciferol or cholecalciferol. Although the KDOQI guidelines suggest a dosage regimen for ergocalciferol, this is not always effective in achieving correction of the low levels of 25(OH)D in this patient group. The reasons for this are currently unclear. However, if the levels of 25(OH)D are increased then PTH values are seen to decrease in CKD stage 3. The decrease in PTH in patients with CKD stage 4 seems to be less, but again, there is marked heterogeneity in response to ergocalciferol. Accordingly, the use of active vitamin D sterols can be considered. The preparations available in North America are: calcitriol (1,25-dihydroxyvitamin D 3 ), doxercalciferol (1α-25-hydroxyvitamin D 2 ), and paricalcitol (19-nor-1,25-dihydroxyvitamin D 3 ). All are effective in reducing the secondary hyperparathyroidism associated with CKD, consistent with the finding that activation of the VDR in parathyroid glands results in decreased PTH gene transcription. Dosing should be monitored carefully to avoid toxicity, which is mainly manifested by hypercalcemia. In studies in animals, the analogue, paricalcitol, has been shown to be less calcemic and less phosphatemic than the native hormone. There are limited data on head-to-head studies of the active vitamin D sterols in patients, but paricalcitol seems to have the widest therapeutic window. It is likely that early recognition of secondary hyperparathyroidism and initiation of therapy early in the course of CKD may lead to effective control of hyperparathyroidism and prevent parathyroid growth.
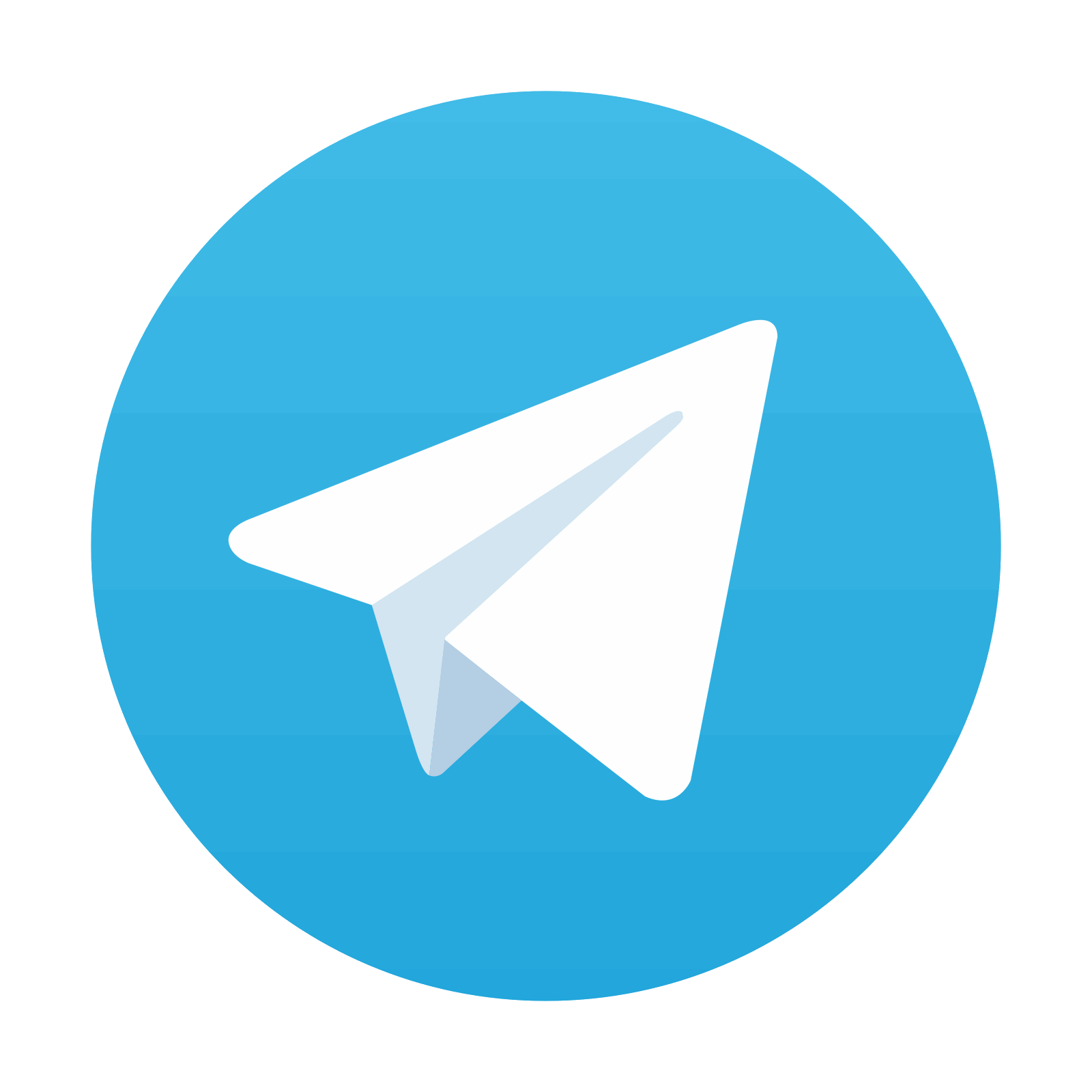
Stay updated, free articles. Join our Telegram channel

Full access? Get Clinical Tree
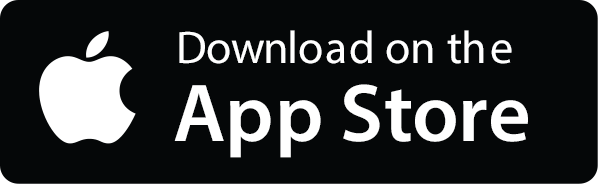
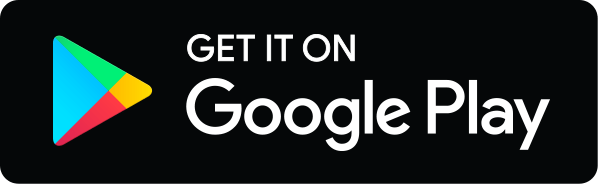