Vitamin D has gone through a renaissance with the association of vitamin D deficiency with a wide array of common diseases including breast, colorectal and prostate cancers, cardio-vascular disease, autoimmune conditions and infections. Vitamin D analogs constitute a valuable group of compounds which can be used to regulate gene expression in functions as varied as calcium and phosphate homeostasis, as well as cell growth regulation and cell differentiation of a wide spectrum of cell types. This review will discuss the full range of vitamin D compounds currently available, some of their possible uses, and potential mechanisms of action.
Of late, vitamin D has gone through a renaissance with the association of vitamin D deficiency with a wide array of common diseases, including breast, colorectal, and prostate cancers; cardiovascular disease; autoimmune conditions; and infections. As a result, vitamin D and its metabolites and analogs constitute a valuable group of compounds that can be used to regulate gene expression in functions as varied as calcium and phosphate homeostasis, as well as cell growth regulation and cell differentiation of a variety of cell types, such as enterocytes, keratinocytes, and epithelial cells of vasculature. The discovery of the metabolites, 25-hydroxyvitamin D 3 (25-OH-D 3 ) (calcidiol) and 1 α ,25-dihydroxyvitamin D 3 (1 α ,25-[OH] 2 D 3 ) (calcitriol), in the early 1970s led to their chemical synthesis, and over the past 3 decades, the development of several generations of calcitriol analogs. The pharmaceutical industry has attempted to separate the calcemic properties of 1 α ,25-(OH) 2 D 3 from its cell-differentiating properties to develop vitamin D analogs with specialized calcemic or noncalcemic (cell-differentiating) uses. Several low-calcemic agents in the forms of calcipotriol, 22-oxacalcitriol (OCT), 19-nor-1 α ,25-(OH) 2 D 2 , and 1 α -OH-D 2 have resulted, with widespread use in the treatment of psoriasis and secondary hyperparathyroidism. Research has also focused on the synthesis of vitamin D receptor (VDR) antagonists and cytochrome P450 (CYP) 24 inhibitors, which block VDR-mediated action or the catabolism of 25-OH-D and 1 α ,25-(OH) 2 D to provide agents with possible use in metabolic bone diseases, osteoporosis, and cancer. Perception of the importance of vitamin D/25-OH-D repletion has been modified by the concept that some 1 α ,25-(OH) 2 D 3 is produced locally by target cells, making this molecule an endocrine and intracrine factor. This review discusses the full spectrum of vitamin D compounds currently available, some of their possible uses, and potential mechanisms of action.
Pharmacologically important vitamin D compounds
Vitamin D compounds can be subdivided into 4 major groups ( Figs. 1–4 ).




Vitamin D and Its Natural Metabolites
Fig. 1 shows the structures of vitamin D 3 and some of its important metabolites. Ironically, vitamin D 3 , the natural form of vitamin D, is not approved for use as a drug in the United States, whereas it is found increasingly as an over-the-counter natural food supplement and is used in both roles in virtually every other country in the world.
During the late 1960s and early 1970s, most of the principal vitamin D metabolites were first isolated and identified by gas chromatography–mass spectrometry and their exact stereochemical structure determined. This led to chemical synthesis of the naturally occurring isomer and its testing in various biologic assays in vitro and in vivo. All the major metabolites, namely, 25-OH-D 3 (Calderol), 1 α ,25-(OH) 2 D 3 (Rocaltrol), and 24,25-dihydroxyvitamin D 3 24( R ),25-(OH) 2 D 3 , Secalciferol) are currently or have been available for use as drugs globally.
Vitamin D Prodrugs
Fig. 2 lists some of the important prodrugs of vitamin D. All of these compounds require a step (or more) of activation in vivo before they are biologically active. Included here is vitamin D 2 (ergocalciferol), which is derived from the fungal sterol, ergosterol, by irradiation. When the nutritional basis of rickets and osteomalacia became apparent in the first half of the twentieth century, vitamin D (in particular vitamin D 2 because it was less expensive) became the treatment of choice for these diseases. In North America, low-dose prophylactic vitamin D (400 IU) in the form of dietary supplements or fortification of milk, margarine, bread, and other food products replaced much of the need for therapeutic vitamin D to abolish overt rickets and osteomalacia. Florid vitamin D deficiency rickets has become uncommon in North America because of adherence to public health guidelines and the fact that vitamin D fortification is required by law, in contrast to before fortification, and it is still more prevalent in the world where food fortification is not practiced. In the United States, vitamin D 2 is the form of vitamin D used exclusively in pharmaceutical preparations, whereas vitamin D 3 is increasingly incorporated in over-the-counter supplements. Vitamin D 2 differs only in that it possesses 2 specific modifications of the side chain (see Fig. 2 ) but these differences do not preclude the same series of activation steps as vitamin D 3 , these hydroxylations giving rise to the metabolites 25-OH-D 2 , 1 α ,25-(OH) 2 D 2 , and 24,25-(OH) 2 D 2 respectively. Recently, there has been controversy regarding the relative usefulness of dietary vitamin D 2 and vitamin D 3 to raise the circulating 25-OH-D level. Evidence suggests that oral pharmacologic doses of vitamin D 3 are significantly more effective than equivalent doses of vitamin D 2 for increasing the 25-OH-D level to the sufficient range (>40 ng/mL) whereas there is ample evidence vitamin D 2 compounds are less toxic than their vitamin D 3 counterparts. Alternatively, other studies using smaller, daily oral dosing of vitamins D 2 and D 3 suggest approximate bioequivalence.
25-OH-D 3 was developed and approved as the pharmaceutical preparation Calderol in the 1970s but was withdrawn recently and is currently available only in Europe. Two other prodrugs, 1 α -OH-D 3 and 1 α -OH-D 2 , were synthesized in the 1970s as alternative sources of 1 α ,25-(OH) 2 D 3 and 1 α ,25-(OH) 2 D 2 , respectively, that in the process circumvent the renal 1 α -hydroxylase enzyme, which was shown to be tightly regulated and prone to damage in renal disease. The prodrug, 1 α -OH-D 5 , has been in clinical trials for the treatment of breast cancer. The 1α-hydroxylated prodrugs have also been used in the treatment of osteoporosis. Although the etiology of this disease is complex and likely multifactorial, there have been consistent claims that serum levels of 1 α ,25-(OH) 2 D 3 are low in osteoporosis. In addition, evidence that certain VDR genotypes correlate with bone mineral density suggests some genetically inherited basis involving vitamin D exists leading to increased susceptibility to osteoporosis. As a consequence, it is not surprising that clinical trials of 1 α -OH-D 3 , 1 α -OH-D 2 , and 1 α ,25-(OH) 2 D 3 have led to reports of modest gains in bone mineral density and reductions in fracture rates in osteoporotic patients, a topic reviewed by Seeman and colleagues.
The final compound in Fig. 2 , dihydrotachysterol (DHT) has a complex history as a prodrug. Originally it was believed to be active when converted to 25-OH-DHT by virtue of an A ring rotated 180° such that the 3 β -hydroxyl function assumes a pseudo-1 α -hydroxyl position. The mechanism of action of DHT has become less clear with the description of the extrarenal metabolism of 25-OH-DHT to 1 α ,25-(OH) 2 -DHT and 1 β ,25-(OH) 2 -DHT, 2 further metabolites that have greater biologic activity than the two 25-OH-DHT metabolites or DHT themselves.
Calcitriol Analogs
Fig. 3 lists some of the most promising vitamin D analogs of 1 α ,25-(OH) 2 D 3 approved by governmental agencies; currently under development by various industrial or university research groups; or abandoned at various stages of the development process. Because the number of vitamin D analogs synthesized now lists in the thousands, the table is provided mainly to give a flavor of the structures experimented with thus far, the worldwide scope of the companies involved, and the broad spectrum of target diseases and uses.
The first generation of calcitriol analogs included molecules with fluorine atoms placed at metabolically vulnerable positions in the side chain and resulted in highly stable and potent calcemic agents, such as 26,27-F 6 -1 α ,25-(OH) 2 D 3 . A second generation of analogs focused on features that make the molecule more susceptible to clearance, such as in calcipotriol (MC903), where a C22-C23 double bond, a 24-hydroxyl function, and a cyclopropane ring have been introduced into the side chain or in OCT, where the 22-carbon has been replaced with an oxygen atom. Both modifications have given rise to highly successful analogs, calcipotriol and maxacalcitol, marketed in Europe and Japan, respectively.
The C24 position is the chemists’ favorite site for modification and many analogs contain 24-hydroxyl groups (eg, 1 α ,24[ S ]-[OH] 2 D 2 and 1 α ,24[ R ]-[OH] 2 D 3 ). Other analogs contain multiple changes in the side chain in combination, including unsaturation; 20-epimerization; 22-oxa replacement; and homologation in the side-chain or terminal methyl groups. The resultant molecules, such as EB1089 and KH1060, attracted the strong attention of researchers because of their increased potency in vitro and were pursued as possible anticancer and immunomodulatory compounds, respectively, but their development seems to have been stalled.
Attempts have been made to modify the nucleus of calcitriol. The Roche compound, 1 α ,25-(OH) 2 -16-ene-23-yne-D 3 , touted as an antitumor compound in vivo, possesses a D-ring double bond. Declercq and Bouillon have made a novel 14-epi,19-nor-23-yne derivative with the same 23-yne feature, which also holds promise in cancer therapy (see Fig. 3 ), and the same researchers have introduced a series of biologically active analogs without 1 of the other of the C/D rings but with a rigid backbone to maintain the spatial arrangement of the A-ring hydroxyl groups and the side chain (see Fig. 4 ). The A-ring–substituted 2-hydroxypropoxy-derivative, ED71, which by virtue of an A-ring substituent at C2 and tighter binding affinity to DBP has a longer half-life in the plasma, has been tested as an antiosteoporosis drug. It has been claimed that ED71 has performed well at restoring bone mass without causing hypercalcemia in long-term studies involving ovariectomized rats and in phase I and II clinical trials. Other bulky modifications at the C2 position of the A ring are accommodated well by the VDR, as indicated by modeling and biologic activity studies. Another C-substituted bone-specific analog, 2MD, is currently at an early stage of pharmaceutical development for the treatment of osteoporosis.
19-nor-1 α ,25-(OH) 2 D 2 , lacks a 19-methylene group and is similar to the in vivo active metabolite, 1 α ,25-(OH) 2 DHT 2 , formed from dihydrotachysterol, because it lacks a functional group at the pseudo C19 position. Many other compounds have been developed with rigid or altered cis -triene structures or modifications of the 1 α -, 3 β -, or 25-hydroxyl functions for use as drugs but allow establishing minimal requirements for biologic activity in structure/activity studies. BXL628 combines 1-flourination; 16-ene and 23-ene unsaturations; 26,27-homologation; and 20-epimerization, all found in earlier generations of analogs to make a antiproliferative agent currently in clinical trials for the treatment of prostate cancer and prostatitis.
Miscellaneous Vitamin D Analogs and Associated Drugs
One series of compounds (depicted in Fig. 4 ) is the substituted biphenyls originally developed by Ligand, representing nonsteroidal scaffolds selected by high-throughput screening, which show weak VDR binding but good transactivation through vitamin D responsive element (VDRE)–driven, vitamin D–dependent genes and produce hypercalcemia in vivo. This family has recently been extended by the synthesis of some highly potent, tissue-selective nonsecosteroidal VDR modulators with nanomolar affinity (eg, LY2109866). This is the first class of vitamin D mimics that lack the conventional cis -triene secosteroid structure while maintaining the spatial separation of the A-ring and side-chain hydroxyl functions needed to bind to certain key residues of the ligand-binding pocket of the VDR. Although these nonsecosteroidal compounds are purported to exhibit an improvement of the therapeutic index over calcitriol in animal models, they are still to be tested clinically. In addition, Fig. 4 shows the structures of 2 different classes of VDR/cacitriol antagonists. The former compounds, including TEI-9647, are dehydration products of the metabolite, 1 α ,25-(OH) 2 D 3 -26,23-lactone (see Fig. 1 ), and are used in the treatment of Paget disease.
Another group of compounds that has an impact on the vitamin D field that is under development are the CYP24A1 inhibitors. By blocking CYP24A1, the main catabolic pathway within the vitamin D target cell, these agents extend the life of the natural agonist, calcitriol, giving rise to a longer-lasting biologic effect. Sandoz/Novartis developed a group of molecules that have greater specificity toward CYP24A1 and CYP27B1 from the general CYP inhibitor, ketoconazole, which showed usefulness in blocking cell proliferation in vitro but these compounds were discontinued after early clinical trials. Cytochroma has developed a library of CYP24A1 inhibitors synthesized by Posner and colleagues based on vitamin D templates; some of these are pure CYP24A1 inhibitors whereas others are mixed VDR agonist/CYP24A1 inhibitors (see Fig. 4 ). Some of these drugs have currently reached phase IIB human clinical trials for the treatment of psoriasis and are being tested systemically in the treatment of secondary hyperparathyroidism. Their promise stems from their ability to block the attenuating action of CYP24 on calcitriol-mediated pre-pro-parathyroid hormone (PTH) gene suppression.
Clinical applications of vitamin D compounds
The clinical potential of vitamin D analogs has been discussed comprehensively in published reviews. This article focuses on diseases currently treated with vitamin D analogs.
Secondary Hyperparathyroidism
Chronic kidney disease (CKD) is accompanied by a gradual fall in serum 1 α ,25-(OH) 2 D levels over the 5-stage natural history of the disease, stages being defined by the decline in glomerular filtration rate (GFR). CKD culminates in the need for dialysis (stage 5). For 3 decades, this reduction in serum 1 α ,25-(OH) 2 D was assumed the result of a decline in 1α-hydroxylase (CYP27B1) activity, due in turn to loss of the protein itself, but the recent elucidation of the FGF23-regulated phosphate homeostatic pathway has opened up an alternative explanation. Because FGF23 triggers down-regulation of 1α-hydroxylase (CYP27B1) and up-regulation of the catabolic 24-hydroxylase (CYP24A1), this hormone may also contribute to the progressive reduction in circulating 1 α ,25-(OH) 2 D levels. Furthermore, FGF23 rises as early as CKD stage 1, preceding the decline in the 1α-hydroxylase activity that occurs in CKD stage 2 and well before the hypocalcemia and secondary hyperparathyroidism, which characterize the later stages of this disease. Unchecked, these biochemical events, together with the other sequelae of renal failure, such as phosphate retention, can result in renal osteodystrophy. Active vitamin D analogs, such as 1 α -OH-D 3 and 1 α ,25-(OH) 2 D 3 , raise plasma Ca 2+ concentrations and, in addition, lower PTH levels by direct suppression of PTH gene transcription at the level of the PTH gene promoter. Slatopolsky and colleagues showed that intravenous infusion of active vitamin D preparations results in a more effective suppression of plasma PTH levels without such a profound increase in plasma (Ca 2+ ) in end-stage renal disease (ESRD). Subsequent work has used low-calcemic vitamin D analogs, such as 1 α -OH-D 2 (doxercalciferol), OCT, or 19-nor-1 α ,25-(OH) 2 D 2 (paricalcitol), as substitutes for the more calcemic natural hormone. The Food and Drug Administration has approved oral and intravenous versions of these drugs for the treatment of secondary hyperparathyroidism at stages 3 and 4 of the disease as well as hemodialysis and peritoneal dialysis patients.
In 2003, a body of nephrologists released guidelines recommending more aggressive use of vitamin D preparations and active vitamin D analogs in the treatment of secondary hyperparathyroidism in CKD. Kidney Disease Outcomes Quality Initiative (K/DOQI) guidelines suggested that treatment as early as stage 3 (GFR <60) might benefit patients by limiting the extreme rises in plasma PTH levels and preventing the parathyroid gland resistance to vitamin D treatment often observed in ESRD. K/DOQI guidelines also recognized the high frequency of vitamin D deficiency (25-OH-D <10 ng/mL) and vitamin D insufficiency (25-OH-D 10–30 ng/mL) in the CKD and ESRD populations and made the opinion-based recommendation to make an initial attempt at vitamin D repletion with escalating doses of vitamin D 2 before administration of active vitamin D analog replacement therapy. This initial intervention to boost 25-OH-D levels has proved successful in stage 3 CKD patients in that it increases 1 α ,25-(OH) 2 D and mildly suppresses PTH, but the strategy failed to produce the desired effects in stage 4 CKD patients due to reduced renal 1α-hydroxylase activity. Currently, oral and intravenous formulations of various active vitamin D analogs are available for use in stage 3–5 patients to take over when vitamin D repletion fails to regulate PTH levels.
The emergence of the potential importance of the extrarenal 1α-hydroxylase in normal human physiology has led to a re-evaluation of the vitamin D repletion and active hormone replacement arms of the CKD therapy. The value of the vitamin D repletion is now seen as providing the substrate 25-OH-D for the renal 1α-hydroxylase, which is the main determinant of circulating 1 α ,25-(OH) 2 D 3 , and the extrarenal 1α-hydroxylase, which is postulated to augment 1 α ,25-(OH) 2 D 3 synthesis for local or intracrine actions around the body. Although the decline of the renal enzyme during CKD is well established, the fate of the extrarenal 1α-hydroxylase in the face of uremia is largely a matter of conjecture. Evidence from anephric patients treated with large doses of 25-OH-D 3 suggests that the extrarenal enzyme survives in CKD patients, arguing that provision of a source of 25-OH-D to vitamin D–deficient and insufficient patients throughout all stages of CKD is warranted. The data from anephrics also argues for the more judicious use of active vitamin D analogs as hormone replacement therapy in addition to conventional vitamin D repletion therapy. Early attempts at this type of combined vitamin D/active vitamin D analog approach in a pediatric population have resulted in more efficient PTH control without many of the usual problems of soft tissue calcification observed in patients treated only with active vitamin D analogs. The 3-fold higher susceptibility of CKD patients for cardiovascular disease may also point to the deleterious effects of untreated vitamin D deficiency on the vasculature and highlight the beneficial effects of renally and locally produced 1 α ,25-(OH) 2 D for maintaining normal blood pressure, for antiproliferative effects on myocardial cell hypertrophy, and for direct suppressive effects on vascular epithelial cell osteoblastic gene expression (eg, Runx2 and osterix).
Hyperproliferative Conditions: Psoriasis and Cancer
The demonstration that 1 α ,25-(OH) 2 D 3 is an antiproliferative, prodifferentiating agent for certain cell types in vivo and many cell lines in vitro suggested that vitamin D analogs might offer some relief in hyperproliferative disorders, such as psoriasis and cancer. Early psoriasis trials with systemic 1 α ,25-(OH) 2 D 3 were moderately successful but plagued with hypercalcemic side effects. Modifications to the protocol included (1) administration of calcitriol overnight when intestinal concentrations of [Ca 2+ ] were low and (2) substitution of low-calcemic analogs for the calcitriol.
Although oral calcitriol can be an effective treatment for psoriasis when administered using an overnight protocol, the most popular treatment for psoriasis is the topical administration of the low-calcemic analog, calcipotriol, formulated as an ointment, which results in an improvement in more than 75% of patients. 1 α ,25-(OH) 2 D 3 and calcipotriol are effective in psoriasis because they block hyperproliferation of keratinocytes, increase differentiation of keratinocytes, and help suppress local inflammatory factors through their immunomodulatory properties. Calcipotriol has been marketed worldwide for use in psoriasis for more than 15 years.
Several thousands of vitamin D analogs have been tested in vitro and in vivo with some degree of success in controlling the growth of tumor cells, thus offering potential for use as anticancer drug therapies. Many vitamin D compounds are effective antiproliferative or prodifferentiation agents in vitro acting through a variety of mechanisms involving alterations of cell cycle genes and proapototic genes to produce their effects. Preclinical studies in laboratory animals have also resulted in promising data. With the analog EB1089, the promising antiproliferative effects observed in vitro and in the N-nitroso-N-methylurea (NMU)-induced mammary tumor and in LNCaP prostate cancer xenograft models were also extended into the clinic. Early trials in limited numbers of breast cancer patients have been followed-up with more extensive ongoing phase II and phase III clinical trials in several different cancers. Several other anlogs have entered clinical trials for the treatment of a variety of hyperproliferative diseases, usually involving VDR-positive tumors.
Despite the promise of vitamin D analogs as anticancer agents, there is yet to result in an approved vitamin D analog for use in any type of cancer. The principal problem in anticancer studies involving orally administered vitamin D compounds is hypercalcemia. Although the newer analogs seem less calcemic than calcitriol itself, they retain some ability to raise serum calcium; they are not noncalcemic as is sometimes claimed. Another problem emerging from experience with clinical trials is that effective doses needed to retard cell growth (approximately 1 nM or higher) cannot be attained in vivo due to low bioavailability. One of the determinants of tumor cell vitamin D analog levels is the catabolic enzyme, CYP24A1, which is up-regulated in vitamin D target cells and limits the effective drug concentration reached. Thus, another approach to effective vitamin D therapy in cancer patients is the potential use of a CYP24 inhibitor (see Fig. 4 ) with or without calcitriol/calcitriol analog. Nevertheless, it remains uncertain if a vitamin D compound can be developed that is sufficiently devoid of calcemic activity while retaining significant antiproliferative activity to be effective against tumors and also surviving the catabolic processes that operate in vivo.
Clinical applications of vitamin D compounds
The clinical potential of vitamin D analogs has been discussed comprehensively in published reviews. This article focuses on diseases currently treated with vitamin D analogs.
Secondary Hyperparathyroidism
Chronic kidney disease (CKD) is accompanied by a gradual fall in serum 1 α ,25-(OH) 2 D levels over the 5-stage natural history of the disease, stages being defined by the decline in glomerular filtration rate (GFR). CKD culminates in the need for dialysis (stage 5). For 3 decades, this reduction in serum 1 α ,25-(OH) 2 D was assumed the result of a decline in 1α-hydroxylase (CYP27B1) activity, due in turn to loss of the protein itself, but the recent elucidation of the FGF23-regulated phosphate homeostatic pathway has opened up an alternative explanation. Because FGF23 triggers down-regulation of 1α-hydroxylase (CYP27B1) and up-regulation of the catabolic 24-hydroxylase (CYP24A1), this hormone may also contribute to the progressive reduction in circulating 1 α ,25-(OH) 2 D levels. Furthermore, FGF23 rises as early as CKD stage 1, preceding the decline in the 1α-hydroxylase activity that occurs in CKD stage 2 and well before the hypocalcemia and secondary hyperparathyroidism, which characterize the later stages of this disease. Unchecked, these biochemical events, together with the other sequelae of renal failure, such as phosphate retention, can result in renal osteodystrophy. Active vitamin D analogs, such as 1 α -OH-D 3 and 1 α ,25-(OH) 2 D 3 , raise plasma Ca 2+ concentrations and, in addition, lower PTH levels by direct suppression of PTH gene transcription at the level of the PTH gene promoter. Slatopolsky and colleagues showed that intravenous infusion of active vitamin D preparations results in a more effective suppression of plasma PTH levels without such a profound increase in plasma (Ca 2+ ) in end-stage renal disease (ESRD). Subsequent work has used low-calcemic vitamin D analogs, such as 1 α -OH-D 2 (doxercalciferol), OCT, or 19-nor-1 α ,25-(OH) 2 D 2 (paricalcitol), as substitutes for the more calcemic natural hormone. The Food and Drug Administration has approved oral and intravenous versions of these drugs for the treatment of secondary hyperparathyroidism at stages 3 and 4 of the disease as well as hemodialysis and peritoneal dialysis patients.
In 2003, a body of nephrologists released guidelines recommending more aggressive use of vitamin D preparations and active vitamin D analogs in the treatment of secondary hyperparathyroidism in CKD. Kidney Disease Outcomes Quality Initiative (K/DOQI) guidelines suggested that treatment as early as stage 3 (GFR <60) might benefit patients by limiting the extreme rises in plasma PTH levels and preventing the parathyroid gland resistance to vitamin D treatment often observed in ESRD. K/DOQI guidelines also recognized the high frequency of vitamin D deficiency (25-OH-D <10 ng/mL) and vitamin D insufficiency (25-OH-D 10–30 ng/mL) in the CKD and ESRD populations and made the opinion-based recommendation to make an initial attempt at vitamin D repletion with escalating doses of vitamin D 2 before administration of active vitamin D analog replacement therapy. This initial intervention to boost 25-OH-D levels has proved successful in stage 3 CKD patients in that it increases 1 α ,25-(OH) 2 D and mildly suppresses PTH, but the strategy failed to produce the desired effects in stage 4 CKD patients due to reduced renal 1α-hydroxylase activity. Currently, oral and intravenous formulations of various active vitamin D analogs are available for use in stage 3–5 patients to take over when vitamin D repletion fails to regulate PTH levels.
The emergence of the potential importance of the extrarenal 1α-hydroxylase in normal human physiology has led to a re-evaluation of the vitamin D repletion and active hormone replacement arms of the CKD therapy. The value of the vitamin D repletion is now seen as providing the substrate 25-OH-D for the renal 1α-hydroxylase, which is the main determinant of circulating 1 α ,25-(OH) 2 D 3 , and the extrarenal 1α-hydroxylase, which is postulated to augment 1 α ,25-(OH) 2 D 3 synthesis for local or intracrine actions around the body. Although the decline of the renal enzyme during CKD is well established, the fate of the extrarenal 1α-hydroxylase in the face of uremia is largely a matter of conjecture. Evidence from anephric patients treated with large doses of 25-OH-D 3 suggests that the extrarenal enzyme survives in CKD patients, arguing that provision of a source of 25-OH-D to vitamin D–deficient and insufficient patients throughout all stages of CKD is warranted. The data from anephrics also argues for the more judicious use of active vitamin D analogs as hormone replacement therapy in addition to conventional vitamin D repletion therapy. Early attempts at this type of combined vitamin D/active vitamin D analog approach in a pediatric population have resulted in more efficient PTH control without many of the usual problems of soft tissue calcification observed in patients treated only with active vitamin D analogs. The 3-fold higher susceptibility of CKD patients for cardiovascular disease may also point to the deleterious effects of untreated vitamin D deficiency on the vasculature and highlight the beneficial effects of renally and locally produced 1 α ,25-(OH) 2 D for maintaining normal blood pressure, for antiproliferative effects on myocardial cell hypertrophy, and for direct suppressive effects on vascular epithelial cell osteoblastic gene expression (eg, Runx2 and osterix).
Hyperproliferative Conditions: Psoriasis and Cancer
The demonstration that 1 α ,25-(OH) 2 D 3 is an antiproliferative, prodifferentiating agent for certain cell types in vivo and many cell lines in vitro suggested that vitamin D analogs might offer some relief in hyperproliferative disorders, such as psoriasis and cancer. Early psoriasis trials with systemic 1 α ,25-(OH) 2 D 3 were moderately successful but plagued with hypercalcemic side effects. Modifications to the protocol included (1) administration of calcitriol overnight when intestinal concentrations of [Ca 2+ ] were low and (2) substitution of low-calcemic analogs for the calcitriol.
Although oral calcitriol can be an effective treatment for psoriasis when administered using an overnight protocol, the most popular treatment for psoriasis is the topical administration of the low-calcemic analog, calcipotriol, formulated as an ointment, which results in an improvement in more than 75% of patients. 1 α ,25-(OH) 2 D 3 and calcipotriol are effective in psoriasis because they block hyperproliferation of keratinocytes, increase differentiation of keratinocytes, and help suppress local inflammatory factors through their immunomodulatory properties. Calcipotriol has been marketed worldwide for use in psoriasis for more than 15 years.
Several thousands of vitamin D analogs have been tested in vitro and in vivo with some degree of success in controlling the growth of tumor cells, thus offering potential for use as anticancer drug therapies. Many vitamin D compounds are effective antiproliferative or prodifferentiation agents in vitro acting through a variety of mechanisms involving alterations of cell cycle genes and proapototic genes to produce their effects. Preclinical studies in laboratory animals have also resulted in promising data. With the analog EB1089, the promising antiproliferative effects observed in vitro and in the N-nitroso-N-methylurea (NMU)-induced mammary tumor and in LNCaP prostate cancer xenograft models were also extended into the clinic. Early trials in limited numbers of breast cancer patients have been followed-up with more extensive ongoing phase II and phase III clinical trials in several different cancers. Several other anlogs have entered clinical trials for the treatment of a variety of hyperproliferative diseases, usually involving VDR-positive tumors.
Despite the promise of vitamin D analogs as anticancer agents, there is yet to result in an approved vitamin D analog for use in any type of cancer. The principal problem in anticancer studies involving orally administered vitamin D compounds is hypercalcemia. Although the newer analogs seem less calcemic than calcitriol itself, they retain some ability to raise serum calcium; they are not noncalcemic as is sometimes claimed. Another problem emerging from experience with clinical trials is that effective doses needed to retard cell growth (approximately 1 nM or higher) cannot be attained in vivo due to low bioavailability. One of the determinants of tumor cell vitamin D analog levels is the catabolic enzyme, CYP24A1, which is up-regulated in vitamin D target cells and limits the effective drug concentration reached. Thus, another approach to effective vitamin D therapy in cancer patients is the potential use of a CYP24 inhibitor (see Fig. 4 ) with or without calcitriol/calcitriol analog. Nevertheless, it remains uncertain if a vitamin D compound can be developed that is sufficiently devoid of calcemic activity while retaining significant antiproliferative activity to be effective against tumors and also surviving the catabolic processes that operate in vivo.
Criteria that influence pharmacologic effects of vitamin D compounds
Three decades of work on vitamin D analogs have shown that several factors play a role in dictating the success of synthetic compounds to mimic some or all of the actions of calcitriol. These factors are discussed briefly.
Activating Enzymes
In vitro models show that some vitamin D compounds lacking 1 α -hydroxylation (25-OH-D 3 and 24[ R ],25-[OH] 2 D 3 ) are capable of interacting with VDR and transactivating reporter genes but this occurs only at high (μM) concentrations of ligand. It seems unlikely that these concentrations will be reached in vivo except in hypervitaminosis D. Consequently, most of the compounds described in Figs. 1 and 2 lack vitamin D biologic activity unless they are activated in vivo. This is the case particularly for the parent vitamin D 3 itself; for its main circulating form, 25-OH-D 3 ; or for any of the prodrugs listed in Fig. 2 . Vitamins D 2 and D 3 depend on the liver 25-hydroxylase and kidney 1 α -hydroxylase enzyme systems to be activated, whereas most prodrugs require only a single step of activation. In particular, the 1 α -OH-D drugs were designed to overcome the tightly regulated 1 α -hydroxylase step, which is defective in chronic renal failure. In essence, prodrugs depend on the weakly regulated 25-hydroxylase step in the liver for activation. CYP27A1, the CYP originally thought responsible for 25-hydroxylation of vitamin D 3 , has been shown to be a bifunctional enzyme that can execute activation of vitamin D 3 and the 27-hydroxylation of cholesterol during bile acid biosynthesis. CYP27A1, however, has a low affinity for vitamin D, does not 25-hydroxylate vitamin D 2 , and when mutated results in cerebrotendinous xanthomatosis, not rickets. Consequently, a more physiologically relevant 25-hydroxylase within the group of candidate orphan P450s may be CYP2R1, because this is a high-affinity microsomal enzyme with a known human mutation causing rickets and has been shown to 25-hydroxylate a vitamin D 2 prodrug, 1α-OH-D 2 . Recently, CYP2R1 was crystallized with several vitamin D substrates in the active site making it likely that it is the physiologically relevant isoform.
The role of extrarenal tissues to 1 α -hydroxylate various 25-hydroxylated metabolites and analogs in normal physiology has been controversial. It was widely accepted, however, that extrarenal 1 α -hydroxylase activity is pathologically relevant in granulomatous conditions (eg, sarcoidosis). In sarcoid patients, 25-OH-D can be converted to 1 α ,25-(OH) 2 D in monocytes/macrophages, a step that, unlike in renal cases, is not subject to tight regulation, thus potentially more likely to result in hypercalcemia. Exposure of such patients to sunlight or administration of 25-OH-D can result in excessive plasma levels of 1 α ,25-(OH) 2 D. After the cloning of the CYP representing the 1 α -hydroxylase (officially named CYP27B1), it was quickly shown that CYP27B1 can also be expressed extrarenally in skin, colon, and lung cancer cells. Knowledge has been extended over the past decade with studies of CYP27B1 messenger RNA levels using real-time PCR and specific anti-CYP27B1 antibodies to show a widespread distribution of this enzyme in many normal tissues as well as pathologic situations. As alluded to previously, the concept of the extrarenal 1α-hydroxylase suggests that this enzyme plays an important physiologic as well as pathologic role and this has raised the level of importance given to ensuring maintenance of adequate 25-OH-D levels by vitamin D or direct 25-OH-D 3 supplementation.
Most of the calcitriol analogs listed in Fig. 3 are thought to be active, not requiring any step of activation before their action on the transcriptional machinery or in nongenomic pathways.
Vitamin D Binding Protein
The vitamin D binding protein (DBP) provides transport for all lipid-soluble vitamin D compounds, from vitamin D to 1 α ,25-(OH) 2 D 3 , so it is not surprising that DBP also carries vitamin D analogs. Most of the analogs of calcitriol, designed to date, contain modifications to the side chain and this is usually detrimental to binding to DBP. Several analogs (eg, calcipotriol, OCT, and 19-nor-1α,25-[OH] 2 D 2 ) have weak affinities for DBP, reduced by as much as 2 to 3 orders of magnitude relative to 1 α ,25-(OH) 2 D 3 . This property has important implications for metabolic clearance rates, delivery to target cells, and tissue distribution. Detailed studies with one analog, OCT, have shown it to bind primarily to β -lipoprotein and exhibit an abnormal tissue distribution in vivo, with disproportionally high concentrations (ng/g tissue) in the parathyroid gland. It was thus proposed that this unusual distribution may make OCT a useful systemically administered drug with a selective advantage in the treatment of hyperparathyroidism. Another vitamin D analog with a modified side chain is 20-epi-1 α ,25-(OH) 2 D 3 , where the 20- S configuration of the side chain is opposite to the normal 20- R configuration. The DBP binding affinity of this analog is virtually unmeasurable as it does not displace [ 3 H]25-OH-D 3 from the plasma binding protein. Reporter gene transactivation assays show that 20-epi-1 α ,25-(OH) 2 D 3 transactivates equally well in COS cells incubated in the presence and absence of fetal calf serum (as a source of DBP), whereas 1 α ,25-(OH) 2 D 3 –induced reporter gene expression is sensitive to DBP in the external growth medium, requiring 2-fold less hormone in the absence of DBP as in its presence. It therefore seems that analogs that bind DBP less well than 1 α ,25-(OH) 2 D 3 derive a target cell advantage over the natural hormone, if they are able to find alternative plasma carrier proteins to transport them to their target cells. These same alternative plasma carriers, however, presumably result in changes in the tissue distribution and hepatic clearance of analogs over the natural metabolites of vitamin D. The recent development of a DBP knockout mouse suggests that 25-OH-D 3 clearance is more rapid in the absence of DBP.
VDR/RXR/VDRE Interactions
Three decades of work have established that 1 α ,25-(OH) 2 D 3 is able to work through a VDR-mediated genomic mechanism to stimulate transcriptional activity at vitamin D–dependent genes. Cloning of the VDR and elucidation of the 3-D structure of its ligand-binding domain have provided a huge boost to delineating the precise conformational changes that take place when the natural ligand binds to the VDR and the nature of the postligand binding transcriptional events that occur thereafter, in particular the nature of the coactivator proteins involved. Basic knowledge of the mechanism of action of vitamin D has also been aided by the opportunity to observe the lack of effects of calcitriol and its analogs in the VDR knockout mouse. These studies have largely refuted claims of alternative non–VDR-mediated mechanisms to produce physiologically relevant effects that might complicate understanding of the pharmacologic effects of vitamin D analogs.
Much evidence exists to support the viewpoint that vitamin D analogs mimic 1 α ,25-(OH) 2 D 3 and use primarily a genomic mechanism. In early work, Stern showed that there exists a strong correlation between chick intestinal VDR binding of an analog and its potency in a [ 45 Ca] rat bone resorption assay, suggesting that a vitamin D analog is only as good as its affinity for the VDR. More recent work has suggested that this a highly simplified viewpoint and that VDR binding affinity may not even be the major factor, transactivation activity arising from a series of additional parameters including conformation of the ligand/VDR complex, binding of the retinoid X receptor (RXR) partner, stability of the VDR/RXR/ligand complex, or even the nature of the coactivator proteins recruited to the complex. Recent data from the superagonist analogs suggest that 20-epi compounds, including KH1060, are consistently only 1 to 2 orders of magnitude more potent than 1 α ,25-(OH) 2 D 3 in gene transactivation assays or differentiation assays. Thus it seems that the quantitative advantages claimed for some of the calcitriol analogs are modest. Part of this advantage can be explained by other factors, such as differences in DBP binding or analog clearance.
Perhaps more important is whether or not analogs can be qualitatively different from 1 α ,25-(OH) 2 D 3 in their actions and work selectively in calcium and phosphate homeostasis or cell differentiation. Freedman and coworkers reported that the ability of a various analogs to transactivate vitamin D–dependent genes or to stimulate differentiation of cells is best correlated with their ability to recruit the coactivator, DRIP205, one of the many components of the DRIP complex isolated by Freedman and coworkers. Among the other coactivators/transcription factors implicated in vitamin D analog action is GRIP1/TIF2, which has been purported to have a particular propensity to interact with the analog OCT. In another study, by Issa and colleagues, a broad panel of vitamin D analogs showed that GRIP1 was more consistently recruited at levels closer to that of 1 α ,25-(OH) 2 D 3 than was another coactivator, AIB1. Work by Peleg and colleagues offers an insight into the purported bone tissue selectivity of the Roche analog, Ro 26-9228 (see Fig. 3 ), renamed BXL628, by showing recruitment of GRIP1 in osteoblasts but not in Caco-2 colon cancer cells, although paradoxically BXL628 is being pursued clinically in prostatic disease rather than osteoporosis. Nevertheless, it seems that there is a fairly strong basis for the hypothesis that differences in the biopotency advantage of certain vitamin D analogs over 1 α ,25-(OH) 2 D 3 are due in part to changes in the recruitment of the RXR dimerization partner or coactivators (eg, see Eelen and colleagues ), but there is no consensus on which of these coactivator proteins is the important one or if these different coactivators can explain tissue/cell selectivity. Work using chromatin immunoprecipitation (CHIP) assays, which shows temporal changes in coactivator recruitment at vitamin D–dependent gene promoters, may aid in understanding this complex transcriptional story.
Target Cell Catabolic Enzymes
Much evidence has accumulated to show that 1 α ,25-(OH) 2 D 3 is subject to target cell catabolism and side-chain cleavage to calcitroic acid via a 24-oxidation pathway catalysed by the CYP, CYP24A1. CYP24A1 is vitamin D inducible because its gene promoter contains a double VDRE; it performs multiple steps in side-chain modification process; it is present in most (if not all) vitamin D target cells, and its role seems to desensitize the target cell to continuing hormonal stimulation by 1 α ,25-(OH) 2 D 3 . The CYP24A1 knockout mouse shows 50% lethality at weaning; death results from hypercalcemia and nephrocalcinosis, and surviving mice show an inability to rapidly clear a bolus dose of 1 α ,25-(OH) 2 D 3 from the bloodstream and tissues. CYP24A1 knockout mice also exhibit a metabolic bone disease reminiscent of the excessive osteoid bone pathology observed in rodents given excessive amounts of 1 α ,25-(OH) 2 D 3 . Moreover, recent work crossing the CYP24 knockout mouse with the VDR knockout mouse rescues this bone defect, suggesting excessive VDR-mediated signaling is the cause, although the bone lesion can also be relieved by administration of 24,25-(OH) 2 D 3 .
The demonstrated importance of CYP24 to 1 α ,25-(OH) 2 D 3 clearance leads the question of whether or not vitamin D analogs might be subject to the same catabolic processes that determine their pharmacokinetics. There are vitamin D analogs, such as calcipotriol, OCT, EB1089, and KH1060, that are metabolized by vitamin D target cells to clearly defined and unique metabolites, which resemble products of the 24-oxidation pathway for 1 α ,25-(OH) 2 D 3 . Furthermore, some of these metabolites are products only of vitamin D target cells and are calcitriol inducible, suggesting that CYP24 is involved in their formation, this having been confirmed with some analogs, such as calcipotriol. Even in the case of several analogs blocked at C24 and subject to metabolism elsewhere on the side chain, the involvement of CYP24 is strongly implicated or proved, including 23-hydroxylation of 26,27-hexafluro-1 α ,25-(OH) 2 D 3 ; 26-hydroxylation of 24-difluro-1 α ,25-(OH) 2 D 3 ; 26-hydroxylation of 1 α ,25-(OH) 2 -16-ene-23-yne-D 3 ; and 26- and 28-hydroxylation of 1 α ,25-(OH) 2 D 2 . Because many of these same products are observed in vitro and in vivo and because pharmacokinetic parameters often parallel target cell metabolic parameters, a conclusion is that target cell metabolism of vitamin D analogs must contribute to the pharmacokinetics and biologic activity observed in vitro and in vivo. Even studies, such as that of Eelen and colleagues, which claimed differences in VDR-mediated gene expression at the coactivator level, also show that a CYP24A1 inhibitor, VID400 (see Fig. 4 ), narrowed potency differences between 23-yne analogs and 1 α ,25-(OH) 2 D 3 by blocking catabolism of the latter and reveal that analogs derive advantages at metabolic and transcriptional levels. Unfortunately, this metabolic blockade approach has not always been used in analog screening and there is little doubt that poor performance during in vivo testing is the result of poor metabolic stability of the studied analog.
Some of the calcitriol analogs with modifications in the vicinity of C23, namely, 20-epi-1 α ,25-(OH) 2 D 3 , 1 α ,25-(OH) 2 -16ene-D 3 , and 20-methyl-1 α ,25-(OH) 2 D 3, undergo 24-oxidation pathway metabolism that stalls at the level of the 24-oxo metabolite, seemingly because the enzyme CYP24A1 cannot efficiently carry out the usual 23-hydroxylation step and complete the catabolic sequence to the inactive cleaved product. The consequence, at least in vitro, is that the 24-oxo metabolite accumulates and there are claims that this metabolite retains significant biologic activity. Recently, this hypothesis has received a boost with the work of Zella and colleagues, who have found that the superagonist 20-epi-1 α ,25-(OH) 2 D 3 exhibits a prolonged duration of action on intestinal calcium regulating genes selectively and these researchers have proposed that this advantage over 1 α ,25-(OH) 2 D 3 stems from a reduction in its catabolic rate.
Hepatic Clearance or Nonspecific Metabolism
The poor DBP binding properties of many side-chain–modified calcitriol analogs opens up the possibility of alternative plasma carriers and accelerated degradation. The liver plays a major role in such metabolic clearance and a few detailed studies performed to date have included in vitro incubation with liver preparations. Calcipotriol, OCT, EB1089, and KH1060 are all subject to metabolism by liver enzymes. One such liver enzyme capable of 23- and 24-hydroxylation of 1 α ,25-(OH) 2 D 3 , and possibly some of its analogs, is the abundant cytochrome P450, CYP3A4. This enzyme is up-regulated by 1 α ,25-(OH) 2 D 3 in duodenum, suggesting a physiologically relevant loop exists. Because over the years, there have been frequent reports of drug-induced osteomalacia associated with coincidental use of anticonvulsants (eg, diphenylhydantoin) or barbiturates and vitamin D preparations, the direct association between CYP3A4 and 1 α ,25-(OH) 2 D 3 is potentially important to explain the putative accelerated clearance of some vitamin D metabolites. One such phenomenon that might be explained by intestinal CYP3A4 action is the purported lower toxicity of vitamin D 2 compounds as compared with their vitamin D 3 counterparts (discussed previously). Work using microsomes from an intestinal cell line and supersomes enriched in recombinant human CYP3A4 catabolize 1 α ,25-(OH) 2 D 2 at a significantly faster rate than 1 α ,25-(OH) 2 D 3 . The implication of this finding is that 1 α ,25-(OH) 2 D 2 and possibly other synthetic analogs, such as the mixed VDR agonist/CYP24A1 inhibitor, CTA018, are selectively broken down in intestine, potentially reducing their gene expression effects on intestinal calcium and phosphate absorption but not on other tissues.
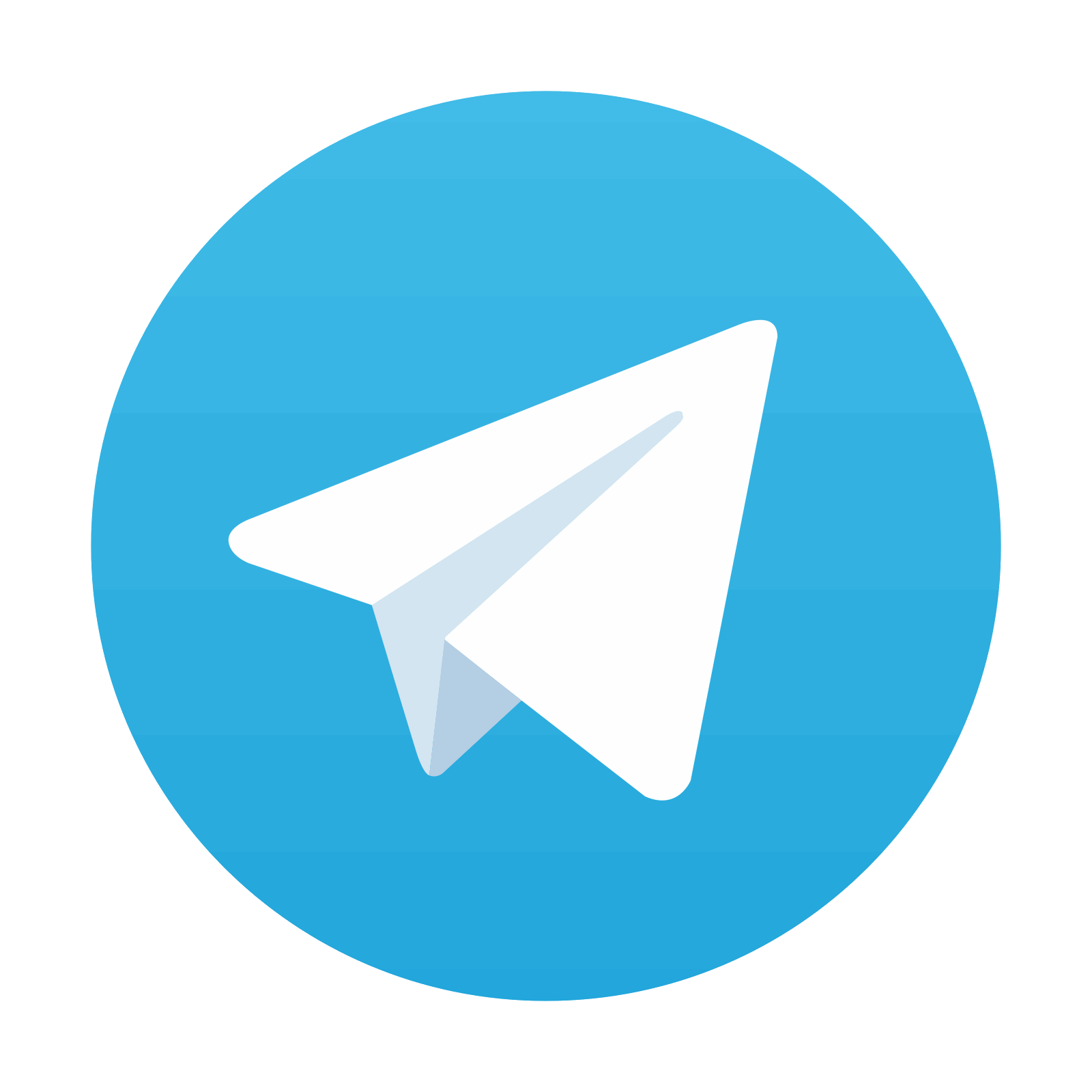
Stay updated, free articles. Join our Telegram channel

Full access? Get Clinical Tree
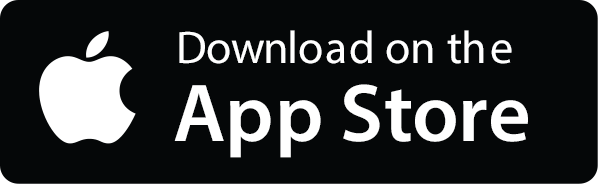
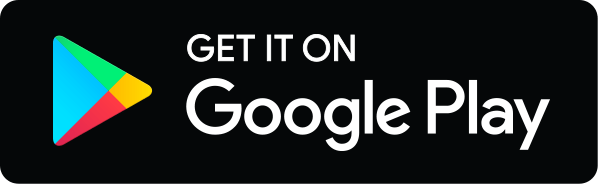