Upper Limb Externally Powered Components
LeRoy H. Oddie CP, MBA, CLCP
Neither LeRoy H. Oddie nor any immediate family member has received anything of value from or has stock or stock options held in a commercial company or institution related directly or indirectly to the subject of this chapter.
This chapter is adapted from Beachler MD, Oddie LR: Upper limb externally powered components, in Krajbich JI, Pinzur MS, Potter BK, Stevens PM, eds: Atlas of Amputations and Limb Deficiencies: Surgical, Prosthetic, and Rehabilitation Principles, ed 4. American Academy of Orthopaedic Surgeons, 2016, pp 175-191.
ABSTRACT
Externally powered prosthetic components are a viable solution for individuals with upper limb amputation or paralysis. Because there are many possible component combinations, selection of an optimal combination can be challenging. Factors to consider in the selection of powered upper limb components include availability, weight, cost, cosmesis, power requirements, noise, durability, repairability, and compatibility, along with the patient’s limb length, gadget tolerance, hand dominance, and the anticipated environments where the prosthesis will be used. Upper limb-powered prehensors, wrists, elbows, and shoulders can be characterized by the mechanical joint type, degree(s) of freedom, joint impedance, and power requirements. By comparing these characteristics with the lost anatomic function and unique requirements of the user, an appropriate combination of externally powered and/or body-powered prosthetic and orthotic components may be prescribed.
Keywords:
externally powered prostheses; hybrid prostheses; myoelectric prostheses; myoelectric orthosis; powered orthosis
Introduction
There have been steady advancements in the field of externally powered upper limb orthotic and prosthetic components. In large part, the field of orthotics and prosthetics has benefited from technological developments of other industries in areas of electronics, software, communication standards, batteries, actuators, manufacturing methods, material science, and mobile communication devices. Consequently, current externally powered upper limb orthoses and prostheses offer increased hand dexterity, longer battery life, more intuitive control, increased function, and new methods of user interaction.1
Although the focus here is primarily on prosthetic components, nonmodular powered upper limb orthoses are commercially available for individuals with upper limb paralysis. Consequently, the terminology focuses on prosthetic components and amputees, yet is also applicable to individuals with upper limb paralysis. Discussion of powered upper limb orthoses is included at the end of the chapter.
The intact upper limb effortlessly performs both fine and gross motor tasks and even subtly contributes to communication.2,3 It should be remembered that the intact upper limb is marvelously capable of a minimum of 28 simultaneous degrees of freedom (DOF),4 with sightless proprioception (including position, heat, moisture, and pressure), with substantial strength for gross motor tasks and delicate dexterity for fine motor tasks, all with seemingly unlimited energy and unconscious control in a visually appealing, lightweight, waterproof package with self-healing properties.
In contrast, the upper limb prosthesis is primarily restricted to a supportive role, is often underactuated, and is generally limited to performing gross motor functions. All upper limb prosthetic components are engineering exercises in compromise, with limitations in power, size, aesthetics, strength, cost, and durability. As prosthetic functionality is increased, the cost, weight, and complexity of the components are also increased.5 In addition, media reports often highlight outcomes of new prosthetic technologies, with no inclusion of important limitations; therefore, older prosthetic technology, which may not only be appropriate but may represent the best solution, is often ignored.6 Thus, the expectations of individuals with upper limb amputations and their families may be greater than a prosthetist is capable of providing. The clinical team and the prosthetist must carefully inculcate realistic functional and aesthetic expectations as early in the rehabilitation process as possible.
Because upper limb prosthetic components are modular and highly compatible, a large number of component combinations are possible. In addition, these components are often complex in design and function, requiring
prosthetists to remain abreast of technological developments and consult with prosthetic manufacturers and other prosthetists who specialize in upper limb components. Prosthetists who are inexperienced in treating patients with complex upper limb amputations should consider referral to a prosthetist who specializes in upper limb care. Many manufacturers of upper limb prostheses employ prosthetists and/or occupational therapists with upper limb expertise who can serve as valuable resources.
prosthetists to remain abreast of technological developments and consult with prosthetic manufacturers and other prosthetists who specialize in upper limb components. Prosthetists who are inexperienced in treating patients with complex upper limb amputations should consider referral to a prosthetist who specializes in upper limb care. Many manufacturers of upper limb prostheses employ prosthetists and/or occupational therapists with upper limb expertise who can serve as valuable resources.
Factors to Consider in Component Selection
Selecting the ideal combination of components is arguably the most difficult aspect in the provision of prostheses for upper limb amputees. Factors to consider in the selection of powered upper limb components include availability, weight, cost, cosmesis, noise, durability, repairability, and component compatibility, as well as patient-related factors such as limb length, gadget tolerance, and the environments where the prosthesis will be used.
Availability
Because the market for powered upper limb components is small, there are substantial product gaps such as the lack of powered wrists with multiple DOF. With knowledge of both what is and what is not commercially available, prosthetists and occupational therapists specializing in the care of individuals with upper limb amputation can serve as excellent resources for recommending appropriate prosthetic components.
Weight
Weight is an important factor in selecting an upper limb prosthesis. Multiple surveys have indicated that excessive weight is a cause for prosthesis abandonment.7,8 Powered upper limb components inherently have more mass than other upper limb components because the sources of actuation (motors) and power (batteries) are usually contained within the prosthesis. However, this increased weight often results in increased function, which may be a priority for some users. Because weight tolerance is dependent on the strength of the individual amputee, weight is more likely to affect smaller individuals. Decreases in component mass are seldom proportional to decreases in component size.
Importantly, not all component weight is equal. Static weight (component weight measured on a scale)9 does not equate to functional weight (the weight of the device perceived by the amputee during use). The more proximal the placement of the weight, the less the user perceives it. Depending on the length of the residual limb and component selection, the prosthetist can strategically decrease functional weight. For example, in a patient with a short transhumeral amputation, the prosthetist may place the battery proximal to the prosthetic elbow or on the posterior side of the socket, rather than within the forearm where the additional weight would decrease elbow capacity and increase perceived weight with humeral flexion. The overall functional and static weight must be considered when selecting a suspension method as heavier components may eliminate the preferred methods from consideration. Generally, less weight is preferable as less weight is often perceived as increased comfort.
Cost
The costs of powered prosthetic components are substantially greater than body-powered alternatives. Often, the end user does not pay for the prosthesis because the prosthetist is reimbursed by a third-party payer such as Medicare, private insurance, or workers’ compensation. However, third-party payers may provide only one prosthesis during the lifetime of an amputee or may not cover the cost of powered prosthetic components because such technologies are considered experimental or investigational.10 In addition, the amputee’s medical coverage may change and the future costs of replacements and/or repairs must be considered. Because cost is not always reflective of function, more expensive components should not be selected unless they are a superior solution for the user’s needs. Similarly, costs should not be a limiting factor unless available financial resources are exceeded. A long-term perspective should be used when considering costs because higher initial costs may provide justification for reduced future costs.11 Knowledge of local and state laws and insurance regulations and the amputee’s financial situation should be understood before a prosthesis is prescribed as some component costs may exceed available funding.11,12
Cosmesis
The importance of cosmesis (lifelike appearance) is highly personal and often culturally influenced.13 Some amputees desire prostheses with a lifelike appearance, preferring detailed custom silicone prostheses, even to the point of sacrificing essential function.13 Other amputees recognize that achieving a lifelike appearance with a prosthesis is challenging and thus prefer prosthetic designs which are far from lifelike. Other amputees adopt an intermediate position in which lifelike appearance is appreciated, but a prosthesis that does not draw the attention of a casual observer is also acceptable.14
Cosmesis can be further differentiated into static and dynamic cosmesis. Static cosmesis, which is the appearance of the prosthesis when not in motion, may be convincing to the casual observer. However, dynamic cosmesis considers the visual normalcy of both movement and appearance.15 Dynamic cosmesis is not limited to the appearance of prosthetic components, but also considers how naturally the residual anatomic movement appears, such as the lack or minimization of compensatory movements.16,17 Dynamic cosmesis often supersedes static cosmesis in importance because unnatural movement will alert the casual observer that the prosthesis is not a natural limb. Dynamic cosmesis may also refer to the motion of a powered prosthetic component, such as direct synchronous supination movement of a wrist rotator in contrast to the smooth motion of the anatomic forearm.18
Noise
For some individuals, noise can be just as important as cosmesis. Noise can draw undesirable attention to the prosthesis, diminishing realism.19 For such individuals, demonstration of powered components before prescription can identify if actuator noise levels are tolerable.
Durability
The importance of durability varies by individual needs. Individuals with upper limb amputations who work in occupations involving manual labor require durable prosthetic components. Unlike the anatomic arm, all prosthetic components lack self-healing properties and have a limited useful life that necessitates eventual replacement. In general, powered upper limb components are less durable than body-powered components. In some instances, it may be challenging to pay for or to obtain reimbursement by third-party payers for the repair and replacement of powered components. The amputee should be encouraged to take personal responsibility in caring for their prosthesis to reduce both the cost and inconvenience of repairs.
Repairability, Warranty, and Service
In some instances, powered upper limb prosthetic components may require repair by the manufacturer. In such cases, consideration must be given to how the amputee will perform essential activities of daily living (ADLs) and instrumental activities of daily living (iADLs) while repairs are made. Solutions may include loaner components from the manufacturer for the duration of the repair, or the provision of a secondary spare prosthesis.
Component Compatibility
Because upper limb prostheses are assembled from various components, the patient’s outcome will only be as successful as the compatibility of such components. Because there is only a limited number of manufacturers making upper limb prosthetic components,20 the available selection of components is limited, and compatibility between components from different manufacturers cannot be assumed. Some manufacturers only ensure compatibility between their own prosthetic components, whereas other manufacturers develop products with the specific purpose of overcoming the incompatibility problem. If there is incompatibility between components, third parties may offer solutions, but the effect on warranty periods should be considered.
Component compatibility can manifest itself in several forms. The most obvious is mechanical compatibility. The quick-disconnect-type wrists made by several manufacturers allow interchangeability with most externally powered terminal devices, although a formal industry standard has not been established. Although most upper limb components have mechanical compatibility, they may not be electrically compatible. Components may operate at different voltages or the connecting cables may not be compatible. Some manufacturers offer adapter cables to enable electrical compatibility. Given the complexity of upper limb components and potential compatibility issues, a prosthetist with upper limb expertise is valuable in understanding appropriate component combinations, whether mechanical or electrical.
Limb Length
The length of the residual limb can have a substantial effect on the selection of appropriate components and, subsequently, available functions. In general, the restored prosthetic limb segments should be matched as closely as possible in function and cosmesis to the contralateral limb in those with unilateral upper limb loss.21 In patients with bilateral upper limb amputation, anthropomorphic ratios serve as a guide, and left/right limb segment symmetry should be restored if possible.21 In elective amputations or revision surgical procedures, the clinical team should consider the length of potential components when planning the amputation length.
For transradial amputations, there is a trade-off between a longer limb that preserves wrist supination and pronation and a shorter limb that allows space for wrist components with flexion features or powered pronation and supination. For transhumeral procedures, a length 12 cm (4.72 inches) shorter than an elbow disarticulation is ideal to permit selection of any elbow components. Although powered elbows can accommodate amputations as long as 5 cm (1.97 inches) short of elbow disarticulation, longer lengths are less desirable because the humeral segment may be longer than ideal.22 Elbow disarticulations and transhumeral amputations that are less than 5 cm shorter than elbow disarticulations will require a body-powered elbow or outside hinges.
Gadget Tolerance
An amputee may have a physical or cognitive limitation (referred to as gadget tolerance) that prevents the use of a prosthesis, or possibly a particular prosthetic component. Each amputee’s ability to adjust emotionally to wearing and using a prosthesis is unique and dependent on their personality and psychological stage of loss.23 As prosthetic components become more complex, a higher gadget tolerance is needed. Modern upper limb-powered prosthetic components have many features, which makes them highly adjustable; however, such components may require interaction with mobile phones, computers, or other devices to access all of the possible features. For example, a prosthetic hand may offer several dozen possible grip patterns, but may require the user to interact with an external device such as a mobile phone to access a certain grip pattern. In addition, all powered prosthetic components currently require battery power, often requiring daily or intraday charging with frequent use. Some amputees may not have the cognitive or physical ability to access the desired features and/or may not be willing or responsible enough to charge batteries daily. For such amputees, simpler externally powered and/or body-powered components may be more appropriate.
Environmental Factors
Powered components have environmental limitations preventing their use in certain situations. Batteries and electronic components can be damaged or compromised by temperature extremes and water exposure. Although some components may be water resistant, most components are not waterproof, and wet environments should be avoided. Sand, dirt, or particle exposure also may compromise function or increase wear rates.24 If an amputee frequently encounters such adverse environments, the manufacturer’s specifications for the selected component should be consulted to ensure component warranties will not be voided with normal use.
Ancillary Equipment
Unlike body-powered prostheses, which require only the power of the amputee, externally powered prostheses require additional equipment for setup, maintenance, and use. Prosthetists and occupational therapists need testing equipment to determine if viable EMG signals are detectable, to refine EMG sensor placement within a prosthetic socket, and to provide muscle training. A computer, tablet, or mobile phone with appropriate software may be necessary for programming the features of microprocessor components. Demonstration tools are available for actuating prehensor devices to demonstrate component function to the amputee before component prescription (Figure 1). Amputees may also need a smartphone or other mobile device to actuate additional programmable modes. Although the patient’s access to software typically does not incur additional costs, hardware costs should be considered when providing powered components.
Preparatory Fitting
Because of many factors involved in component selection and the possibility of prosthesis abandonment, a preparatory fitting can be a highly effective tool to ensure the amputee is capable of using the selected components and that their functions are suited to their needs. A diagnostic socket (test or check socket) not only ensures appropriate socket fit, but also allows component alignment, lengths, and electrode placement to be adjusted.25 After powered components have been prescribed, component manufacturers should be consulted to determine if demonstration components or no-obligation trial periods are available to permit flexibility in selecting the final components.
Hybrid Configurations
Upper limb prostheses can be classified into the three broad categories of oppositional cosmetic restoration, body-powered, or externally powered; however, hybrid designs, with body-powered, passive, and/or externally powered components, are commonly prescribed for patients. Hybrid prostheses combine the advantages of both body-powered and externally powered designs, particularly for individuals with high-level and/or bilateral upper limb amputations.26 Hybrid prostheses may reduce weight, provide simultaneous control of multiple DOF, reduce costs, increase grasping force and lifting capacities, and/or permit finer motor control.26 However, hybrid prostheses also may compromise working envelopes and increase harnessing requirements.
In higher level upper limb amputations, the critical deciding factor in hybrid prosthesis design is often the choice of whether to power the elbow or the prehensor. Although both configurations are feasible, powering the prehensor seems to be the most advantageous choice.27 As the number of possible hybrid configurations is vast, an in-depth discussion is beyond the scope of this chapter. However, some hybrid upper limb components are discussed as appropriate.
Characteristics of Externally Powered Components
Joint Types
Joints are essential for functional movement. To understand how prosthetic components mimic the anatomic upper limb, it is helpful to describe the function of the anatomic joints from the perspective of simple mechanical joints (Figure 2). Anatomic upper limb joints may be revolute with one DOF, universal with two DOF, or spherical with three DOF.28 In addition, various joints can be combined in series or in parallel to increase the available DOF.29
Joint Impedance (Compliance)
In the anatomic limb, joint impedance (resistance to external force) varies depending on the task. Although variable impedance is feasible for powered prosthetic components, it is very inefficient. Consider the elbow holding a book while reading. The impedance of the arm equals the force of the gravity acting on the book and forearm. In a mechanical system with continuously variable impedance, continual energy would be required to maintain elbow joint position. To conserve energy resources, prosthetic components typically adopt a dual-state impedance control with low impedance (free motion) to move the joint to a desired position
and high impedance to lock a joint into position.30 Other prosthetic components, such as the multiaxial wrist, have a spring-loaded impedance, returning the joint to a neutral position after an external force is removed. To prevent actuator damage, some components have clutch mechanisms to allow low impedance (break-away) after a predetermined force is exceeded.
and high impedance to lock a joint into position.30 Other prosthetic components, such as the multiaxial wrist, have a spring-loaded impedance, returning the joint to a neutral position after an external force is removed. To prevent actuator damage, some components have clutch mechanisms to allow low impedance (break-away) after a predetermined force is exceeded.
Movement Quality
Movement quality can refer to the extent to which the movement of powered actuators replicates lifelike motion. Many upper limb component actuators have crude singular velocity movements, which fall substantially short of the natural rapid accelerations and decelerations of normal human movement. In addition, it is common for amputees to overshoot the desired joint position, which increases task time and cognitive load and may lead to user frustration. As technology advances, the quality of movement of prosthetic components may receive more attention, improving functional outcomes and behavioral appearance.
External Power Source
Although unusual power sources, including compressed gas, rocket fuel, methanol, spinal fluid, and implantable glucose fuel cells, have been considered, all current available powered components rely on battery power.31,32 A user may tolerate daily battery charging but may find intraday battery replacement inconvenient, particularly if the second battery must be carried in-person. Typical daily battery use expectancy should be considered in component prescription as the charging frequency may influence prosthesis acceptance.
Battery performance is dependent on several variables. Battery technology determines the relative power density, with lithium-ion batteries being the current standard. High power density is preferred because longer performance can be achieved with a similar weight. From a user’s perspective, it is desirable to have a battery last an entire day before requiring recharging. The capacity of various batteries can be evaluated by comparing the milliamp-hour (mA h) rating, with a higher number representing increased time performance.26
Polymer batteries are packaged in a soft pouch, which decreases weight and rigidity. These batteries are advantageous for prosthetic applications because multiple thin flexible cells may be strategically located, such as proximally placed cells that are contoured to the socket to decrease perceived functional weight (Figure 3). Polymer batteries are not the same as lithium-polymer liquid electrolyte batteries, although both lithium-ion and lithium-polymer chemistries are available in flexible polymer packaging.33
Powered prosthetic systems may operate at differing voltages. For prostheses with multiple powered components, the voltage of one component may vary from another to maximize actuator performance. For example, the elbow requires much higher torque than the thumb or fingers because of the substantially longer forearm lever. To accommodate such complexity, dual-voltage batteries are available, or (less preferably) two batteries may be used.
User convenience is affected by battery type and placement. Some batteries are internal and must be recharged using a charging port before the prosthesis is useable. Other batteries are external (removable) to permit a charged replacement battery to be used while the depleted battery is being recharged. Batteries should be placed as proximally as possible to diminish perceived functional weight and maximize actuator capacity. Proprietary batteries may offer increased performance, but come at the expense of limited local availability. Battery charging times may become a factor if the amputee is not willing to carry a second battery when the capacity is insufficient for a full day of use.
![]() FIGURE 3 Photograph of the FlexCell Mini, a flexible lithium-ion polymer battery for use in upper limb prostheses. (Reproduced with permission from Infinite Biomedical Technologies.) |
![]() FIGURE 4 Depiction of the Myo Kinisi, an anthropomorphic single-degree-of-freedom prehensor. (Reproduced with permission from Steeper, Leeds, UK.) |
Prehensors
The compactness of the hand and its many functions make it difficult to replicate in a powered prosthetic prehensor. To adequately replicate the core grasps of the human hand, a prosthetic hand-like prehensor requires atleast three or four DOF: two for the thumb, one for the index finger, and one for the remaining three digits.6 Limited space availability restricts the size and number of motors available for use. Thus, prosthetic hands are often underactuated, attempting to provide similar function with fewer DOF and/or actuators.34
Electrically powered prehensors were developed more than a half a century ago and remain commercially available in several forms. Anthropomorphic prehensors take the general form of an anatomic human hand (Figures 4 and 5), whereas nonanthropomorphic prehensors may be formed in the shape of a hook or gripper (Figures 6, 7 and 8). Initially, prehensors only provided one DOF, with two or three digits in opposition.35 Recent developments in powered prehensor devices have focused on more anatomically influenced functional designs with the thumb and fingers having multiple DOF (Figure 9).
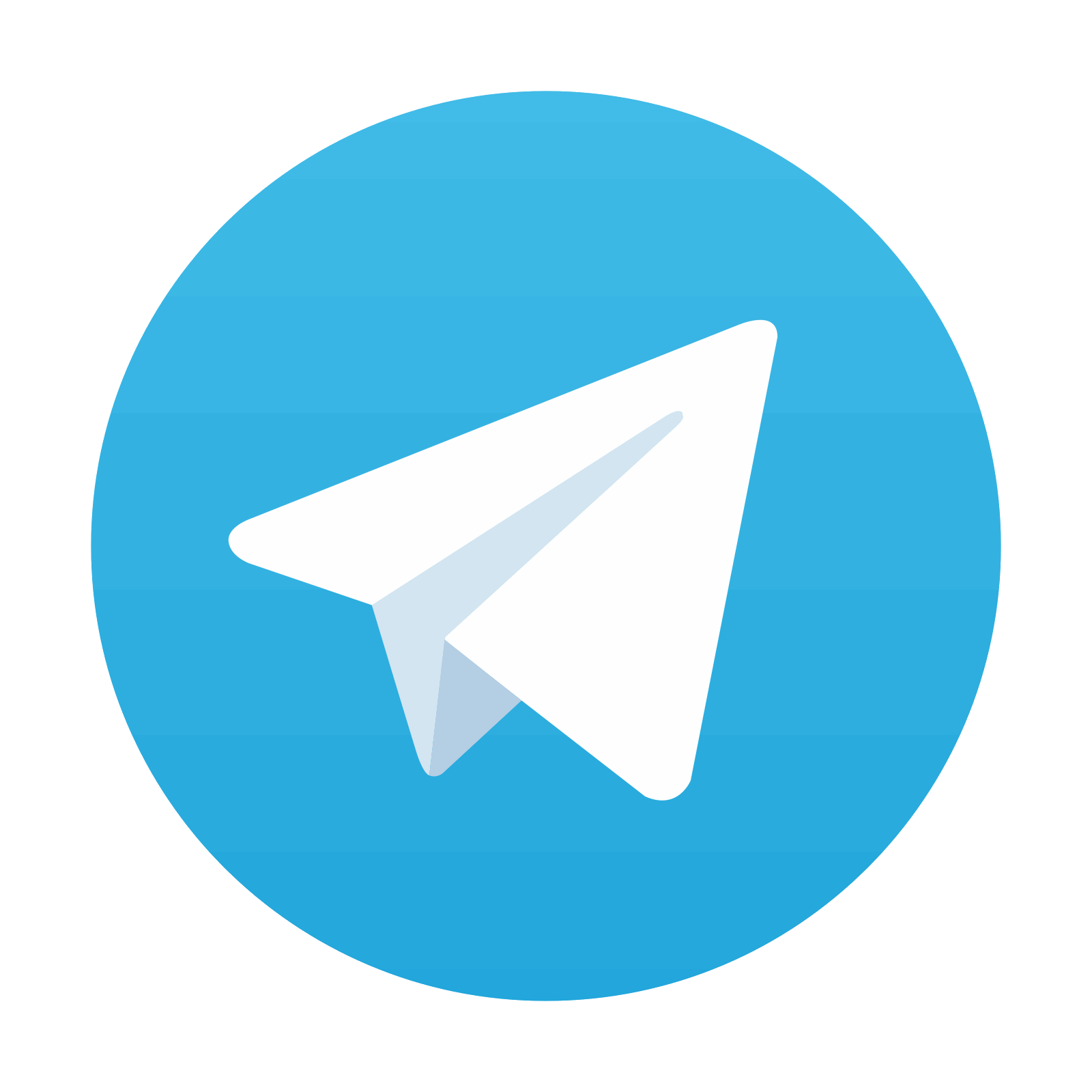
Stay updated, free articles. Join our Telegram channel

Full access? Get Clinical Tree
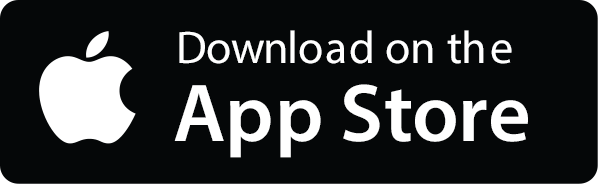
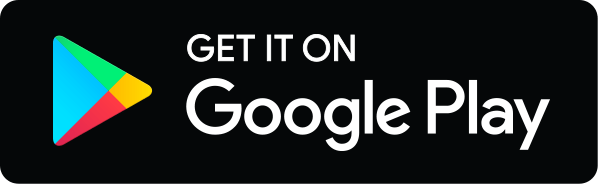