Spinal cord injury (SCI), or traumatic myelopathy, was first described around 2500 BC by ancient Egyptians, as written in the ancient Egyptian medical text the Edwin Smith Surgical Papyrus. The textbook referenced six cases of spinal canal injury, with one case referencing paralysis of the extremities, bladder incontinence, abdominal distention, and priapism.1–4 Subsequently, Hippocrates is often credited with first describing traction for the reduction of spinal fractures with resultant spinal cord injuries, as well as describing chronic paralysis, constipation, bladder difficulty, venous stasis, and pressure injury resulting from spinal injury.1,2,4 Despite a long history of knowledge, most patients with SCIs died within a few weeks, usually from urosepsis, prior to the 1940s.2 It wasn’t until the end of World War II that management began to be standardized. SCI patients were treated in specialized units with multidisciplinary programs dedicated to treatment and rehabilitation of paralysis.2,4 Sir Ludwig Guttman of the United Kingdom and Dr. Donald Munro of Boston, Massachusetts, developed early standardizations of care in the United Kingdom and the United States, respectively.1,3 By the late 1940s, the average life expectancy increased to approximately 10 years and doubled to 20 years in the 1950s; this improvement was attributed to the use of antibiotics and multidisciplinary programs.2
In 1971, Dr. John Young obtained a federal grant to study these programs in order to demonstrate that multidisciplinary programs provided better health outcomes compared to fragmented care for SCI patients in Phoenix, Arizona. He called his system of comprehensive care a “Model System.”1,5,6 The Model System incorporates five components: development of an effective emergency medical services system, acute care, physical rehabilitation, psychosocial and vocational services, and a follow-up program.5 Since its inception, the Model System program expanded to more locations around the United States. Currently there are 14 centers sponsored by the National Institute on Disability and Rehabilitation Research collectively housing the largest database of traumatic SCI information.1,5–7 The U.S. Department of Veterans Affairs (VA) has its own system of care and is the largest single network of comprehensive SCI care in the United States. The VA system is composed of a hub and spoke system with 24 regional centers and 134 primary care teams serving as spokes.8
Advancements in medical care, technology, and acute surgical interventions have changed the standardization of medical practice and delivery of medical services contributing to the decrease in morbidity and mortality following SCI. Specialized medical training is needed to treat patients with SCI as well as the continuation of development of comprehensive treatment centers. The subspecialty of SCI medicine addresses prevention, diagnosis, treatment, and management of both nontraumatic and traumatic etiologies of SCI through an interdisciplinary approach.9 The American Board of Physical Medicine and Rehabilitation (ABPMR) offers subspecialty certification in SCI medicine whereby to be eligible, physicians must complete a 12-month Accreditation Council for Graduate Medical Education (ACGME)-accredited spinal cord injury medicine fellowship.9
Since 1973, the National Spinal Cord Injury Statistical Center (NSCISC), located in Birmingham, Alabama,5 has collected and housed data from the Model System centers. An additional database was created in 1987 by Shriners Hospital for additional data collection on newly injured patients. Although these data have been hugely important in the analysis of the epidemiology and outcomes after SCI, it is important to note that there are limitations to these data as they are estimated to contain only 10% to 15% of all traumatic SCI patients10 and excludes patients with nontraumatic SCI. There are multiple reasons for this, including those who are treated outside of a model system center, those who died, those who may not have sought care, or injury prior to the model systems program.
The overall incidence of new traumatic SCI cases in the United States is approximately 40 per million people per year, equaling 12,000 cases per year of those who survive after their injury. Although the underlying causes of SCI have changed over the last 40 years, the overall incidence remains the same.11 Throughout the world, incidence of traumatic SCI rarely exceeds 20 new cases per million people per year.12–19 This discrepancy is often attributed to the high incidence of violence-related SCI in the United States. According to the Paralyzed Veterans of America study in 1988, approximately 176,965 Americans, or 721 persons per million people, were living with a SCI.20 Lasfargues et al21 in 2014 projected a prevalence of 276,281 people. This increase in patients is attributed to improved life expectancy rather than an increase in the incidence.
Demographics of traumatic SCI vary across the United States, dependent largely on population characteristics including age, gender, and race. The mean age of injury for all patients enrolled in the combined databases is 33.4 years (± 16.7 years), and the most common age of injury is 18 years. The percentage of new patients enrolled in the database over the age of 60 has steadily increased from 4.7% in the 1970s, to 12.9% in the late 2000s. Men make up an overwhelming majority of 80.2%. African Americans have a higher incidence than other ethnicities, which is attributed to increased injuries from violence.24
As of 2015 published data, NSCISC estimates automobile collisions as the major cause of SCI (39.1%), followed by falls (29.5%), acts of violence (14.4%), sports and recreational (8.4%), and other causes (8.6%) which includes medical/surgical, pedestrian, hit by falling/flying object, and other. Since 2000, falls have increased significantly while violence and sports have declined.
With the amount of trauma sustained to induce a traumatic SCI, it comes as no surprise that a significant number of patients enrolled in the combined database report significant associated injuries. A reported 29.3% of patients had other broken bones, 28.2% experienced loss of consciousness at the time of injury with 11.5% classified with a traumatic brain injury, and 17.8% had traumatic pneumothorax.10 Concomitant injuries vary with etiology of injury. With motor vehicle collisions, 42.5% experienced loss of consciousness, 39.7% had broken bones, and 18.4% had head injury.10 Patients injured in a recreational sporting accident were most likely to suffer from loss of consciousness (22.4%), with less than 5% of cases having another associated injury.10 Patients injured in acts of violence had the highest incidence of pneumothorax (35.9%), but other associated injuries were rare among this population.10
Per the NSCISC database, 88.7% of patients were discharged to a private residence, 6.8% were discharged to another acute care hospital or nursing home, and 1.4% were discharged to a group-living facility in the community. It is estimated at 2.5% of patients die during hospitalization. Significant predictors of discharge to nursing home include cervical cord injury without significant motor function below the level of the lesion, being ventilator dependent, older age, unmarried, unemployed, having an indwelling catheter, dependent for activities of daily living (ADLs), Medicaid insurance, and being nonambulatory.25 Of the patients initially discharged to a nursing facility, approximately 30% later reenter the community.25
Life expectancy after traumatic spinal injury is less than average for the average American. Mortality within the first year after injury is roughly 6.7%, but drops to 1.7% in the second year, and reduces to 1.2% for the third year and beyond.26 Several considerations have been identified as risk factors for increased mortality in the first year, including male sex, violence, advanced age, higher neurologic levels of injury, completeness of injury, and using a ventilator.27 Mortality is increased later in life for those with overall poor health, increased dependency with ADLs, means below the federal poverty line, and lower satisfaction with life.28,29
Respiratory disease is the highest cause of mortality in SCI patients at 22%. Of those patients, 70.1% are due to pneumonias, which is 35.6 times the rate of death compared to the healthy population.26 Tetraplegic patients have a higher mortality due to pneumonia than paraplegics, with the higher the injury, the greater the risk (24.7% of deaths occurred in C1–C4 injury levels, while 19.7% of deaths occurred in C5–C8 injury levels). The second most common cause of death for all SCI patients is heart disease at 19.6% of deaths, with hypertensive and ischemic disease accounting for 7.8% of deaths, and other heart disease accounting for 11.8% of deaths. The third leading cause of mortality is infective and parasitic disease (10.4%), with 94.2% of those cases due to sepsis often due to pressure sores, respiratory infections, or urinary tract infections. Deaths due to sepsis are estimated to be 64.2 times that of the American population.26
A normal vertebral column consisting of 7 cervical, 12 thoracic, 5 lumbar, 5 fused sacral, and 4 coccygeal vertebrae and correlated to surface anatomy as can be seen in Fig. 14–1. Figure 14–2 illustrates the path of the spinal cord as it descends as a continuation of the base of the brainstem down to its termination at the conus around the level of L1–L2 in a typical adult. It also demonstrates how the spinal nerves exit in relationship to their bony elements. A closer look at the spinal cord reveals organized ascending sensory and descending motor tracts (Fig. 14–3). The principle ascending and descending pathways are represented in Fig. 14–4. Please refer to Chapter 3 for a detailed review of spinal column and spinal cord anatomy.
Figure 14–1
(A) Surface anatomy of the back showing bony landmarks on the left and cutaneous nerves on the right. (B) Axial section of the back showing the dorsal rami transmitting sensory neurons from the skin of the back to the spinal cord. Normal curvatures of the vertebral column in a newborn (C) and in an adult (D). (Reproduced with permission from Chapter 1. Back. In: Morton DA, Foreman K, Albertine KH, eds. The Big Picture: Gross Anatomy, New York, NY: McGraw-Hill; 2011.)

Figure 14–2
Schematic illustration of the relationships between the spinal cord, spinal nerves, and vertebral column (lateral view), showing the termination of the dura (dura mater spinalis) and its continuation as the filum terminale externum. (Reproduced with permission from Chapter 6. The Vertebral Column and Other Structures Surrounding the Spinal Cord. In: Waxman SG, eds. Clinical Neuroanatomy, 27e New York, NY: McGraw-Hill; 2013.)

Figure 14–3
The spinal cord. (A) Spinal nerves connect the spinal cord with the periphery. Each spinal nerve has a ventral root containing axons of efferent neurons and a dorsal root containing axons of afferent neurons. The dorsal root ganglion is a swelling containing the cell bodies of sensory neurons. The spinal cord is covered in three membranes that comprise the meninges: the pia, the arachnoid, and the dura mater. (Reproduced with permission from Neurophysiology. In: Kibble JD, Halsey CR, eds. Medical Physiology: The Big Picture, New York, NY: McGraw-Hill; 2014.) (B) Cross-section through the spinal cord indicating a butterfly-shaped central area of gray matter. Sensory neurons enter the dorsal horn, and motor neurons leave the ventral horn. The surrounding white matter consists of dorsal, lateral, and anterior columns, each containing nerve tracts running to and from the brain. The ascending and descending tracts shown are present on both the left and right sides. (Reproduced with permission from Hauser SL, Ropper AH. Diseases of the Spinal Cord. In: Kasper D, Fauci A, Hauser S, Longo D, Jameson J, Loscalzo J, eds. Harrison’s Principles of Internal Medicine, 19e New York, NY: McGraw-Hill; 2014.)

Figure 14–4
Transverse section through the spinal cord, composite representation, illustrating the principal ascending (left) and descending (right) pathways. The lateral and ventral spinothalamic tracts ascend contralateral to the side of the body that is innervated. C, cervical; D, distal; E, extensors; F, flexors; L, lumbar; P, proximal; S, sacral; T, thoracic. (Reproduced with permission from Hauser SL, Ropper AH. Diseases of the Spinal Cord. In: Kasper D, Fauci A, Hauser S, Longo D, Jameson J, Loscalzo J, eds. Harrison’s Principles of Internal Medicine, 19e New York, NY: McGraw-Hill; 2014.)

The initial injury after trauma occurs initially by mechanical process characterized primarily by shearing of cell membranes and axons and the disruption of the blood brain barrier. Subsequent injury results from several complex events which are driven by inflammation, production of free radicals and glutamate excitotoxicity – these culminate in neuronal cell death.
These subsequent events are considered the secondary injury cascade and begin within the first 24 to 48 hours of injury. The initial mechanical trauma causes traction and compression of the cord, disrupting axons and surrounding structures. Toxic materials are leaked from these injured cells, causing additional damage to neighboring neurons. Trauma to blood vessels causes hemorrhage and release of a host of cellular mediators of the inflammatory cascade.31 As the spinal cord begins to swell, the outer aspect of the cord can become compressed within the spinal canal, inflicting further damage and ischemia.
Studies have shown release of serotonin, thromboxanes, platelet-activating factor, and other leukotrienes immediately following SCI.31 These agents lead to vasoconstriction, causing further ischemic damage to surrounding tissue.32 Additionally, studies reveal increased concentrations of calcium at the site of injury within minutes of the traumatic event, remaining elevated for a week postinjury.33–36 Increased concentrations of intra-axonal calcium lead to activation of phospholipase C and A2, triggering recruitment of platelet-activating factor, which reduces blood flow and compromises the blood–brain barrier. The activation of phospholipase C and A2 also produces arachidonic acid. Metabolism of arachidonic acid leads to production of free radicals, causing further tissue injury37 and rupture of the cell membranes.38 In addition to calcium, potassium plays a critical role in the acutely injured spinal cord, including depolarization, which can lead to conduction block.39
Microglia respond to the by-products of cellular necrosis by recruiting systemic inflammatory cells, specifically neutrophils, within hours of the injury.31 These neutrophils act to protect against potential bacterial infection; however, the lysosomal enzymes and free radical products of the neutrophilic response degrade the connective tissue, leading to further tissue damage. In the following 2 to 3 days, the neutrophils are replaced by macrophages, with the goal of removing necrotic and toxic cellular debris.40–44 Macrophages mediate the degradation of myelin but are also important in stimulation of fibroblast-mediated release of nerve growth factor (NGF) and fibroblast growth factor (FGF), a powerful angiogenic factor.45 After a week of injury, surrounding astrocytes begin to hypertrophy and proliferate, forming a glial scar, in an attempt to reduce the spread of damage to surrounding healthy tissues.46 While this glial scar is beneficial in preventing spread of inflammatory markers, it prevents axonal regeneration and future reinnervation attempts. After 3 weeks of injury, Wallerian degeneration begins to occur whereby the nerve, which is no longer connected to the cell body, degenerates distal to the injury. In the chronic phase (6 months to 2 years), the site of injury is marked by a multilocular cystic cavity filled with cerebrospinal fluid (CSF), with multiple vascular–glial bundles and regenerated nerve roots. The regenerated nerve roots are characterized by shortened internodes and abnormally thin myelin. Astrocyte and collagenous fibers run through the lesion, stabilizing it further.
The International Standards for Neurological Classification of Spinal Cord Injury (ISNCSCI) exam, colloquially known as the ASIA Impairment Scale (AIS), provides a standard assessment after a traumatic SCI.47 The ISNCSCI exam, last updated in 2011, systematically tests 28 dermatomes and 10 myotomes in order to classify both the level of neurologic injury and the severity of the injury and whether it is considered complete or incomplete.48–50 This exam is only validated in the traumatic spinal cord population and is used for classification of the spinal cord injury only and is not a complete neurologic assessment. This exam can be repeated over time (Fig. 14–5).
Figure 14–5
American Spinal Injury Association International Standards for Neurological Classification of Spinal Cord Injury, 2011. (From the American Spinal Injury Association: International Standards for Neurological Classification of Spinal Cord Injury, Atlanta, GA. © 2011 American Spinal Injury Association. Reprinted with permission.)

The exam is performed with the patient lying supine with the part of the body being tested completely exposed. The sensory portion of the exam includes testing both light touch and pinprick in 28 dermatomes (C2–S4/5) on the left and right sides of the body. Each key testing point is associated with anatomic landmarks.47 Each component of the sensory exam is scored on a 3-point scale. A score of 0 is recorded for absent sensation, 1 is recorded for altered sensation (hypoesthesia, hyperesthesia, or inability to distinguish sharp from dull), and 2 for normal sensation. Deep anal pressure (DAP), tested with a lubricated gloved finger inserted into the rectum, is graded as absent or present.
The motor portion of the exam includes manual muscle strength testing of ten muscles corresponding to ten nerve roots bilaterally (C5–T1 and L2–S1) along with voluntary anal contraction (S2–S4). The strength of each muscle is graded on the standard 6-point scale with scores from 0 to 5.51–54 Each muscle is tested in predetermined positions.47 Voluntary anal contraction (VAC) is graded as absent or present.
Once all sensory and motor components have been tested, a score can be calculated. Although there are nuances to the scoring and exceptions to each rule, generally the sensory level is determined by the most caudal intact dermatomes for both sensory tests, and the motor level is determined by the lowest level where the key muscles tested are scored at least a 3, as long as all muscles above are 5. The single neurological level (NLI) is the most caudal segment with intact sensation and key muscle >/=3, with all other key muscles above it graded as 5. An injury is labeled as complete, or “A,” if there is a lack of sensory or motor function in the S3 or S4/5 segments, including absence of DAP and VAC. An incomplete injury has some form of sacral sparing with the determination of “B,” “C,” or “D” based on how many segments below the NLI there is motor function and how many key muscles are graded >/=3. The grading worksheet can be downloaded for record keeping during the exam and for the patient’s charts from the website www.asia-spinalinjury.org.55 Additional details regarding how to perform and score the ISNCSCI exam can be found at https://lms3.learnshare.com/home.aspx.56
The Jefferson fracture, or atlas (C1) fracture, is a burst fracture of the C1 ring commonly caused by axial loading such as diving or getting hit on the head57 (Fig. 14–6). Unless there is compromise to the cervical spinal canal or disruption of the transverse ligament, these injuries are typically stable without neurologic deficit58 and can be treated with an external orthosis.
Figure 14–6
Jefferson fracture. Axial CT demonstrates four fracture lines (arrows) separating C1 in four parts. Jefferson fracture is usually caused by axial impact to the head such as diving in shallow water. (Reproduced with permission from Furtado AD, Dillon WP. Atlas of Neuroimaging. In: Kasper D, Fauci A, Hauser S, Longo D, Jameson J, Loscalzo J, eds. Harrison’s Principles of Internal Medicine, 19e New York, NY: McGraw-Hill; 2014.).

Injury to the axis (C2) occurs at the odontoid process (dens) or through the pars interarticularis. A hangman’s fracture is a result of bilateral fractures of the pars interarticularis, appearing as a spondylolisthesis of C2. Hangman’s fractures can be stable or unstable. Fractures to the odontoid process can be caused by excessive flexion, extension, and lateral bending and are classified into three types as can be seen in Fig. 14–7.59 Type I and type III fractures are considered stable fractures, whereas type II fractures, which are the most common, are unstable.
Figure 14–7
Diagram showing the three types of fractures of the odontoid process. (Reproduced with permission from Tay BB, Freedman BA, Rhee JM, Boden SD, Skinner HB. Chapter 4. Disorders, Diseases, and Injuries of the Spine. In: Skinner HB, McMahon PJ, eds. Current Diagnosis & Treatment in Orthopedics, 5e New York, NY: McGraw-Hill; 2014.)

Similar to a Jefferson fracture, axial loading to the cervical spine can burst fractures at C3–C7, most commonly at C5. Frequently burst fractures are associated with compression of the spinal cord from retropulsed bony fragments causing neurologic deficits (Fig. 14–8).
Hyperflexion injuries can result in compression fractures, unilateral facet dislocation, bilateral facet dislocation, or teardrop fractures. Unilateral and bilateral facet dislocations are most common at the C5–C6 level and typically result in incomplete and complete spinal cord injuries, respectively. Associated with diving, motor vehicle accident (MVA), or falls, flexion teardrop fractures are characterized by an anterior fragment superheated from a larger retropulsed portion of the vertebral body into the spinal canal. Teardrop fractures are often unstable, as they are associated with disruption of anterior and posterior longitudinal ligaments. Hyperextension injuries involve distraction of the anterior elements of the spinal column while compressing posterior elements. Posterior column compression can result in the buckling of ligamentum flavum into the spinal canal causing compression of the spinal cord.60 Hyperextension injuries are commonly associated with central cord syndrome.
The thoracic spine is thought to be more stable secondary to ribs and intercostal musculature. Overall, the most common level of thoracic injury is T12. Unlike the cervical spine, facet dislocations are uncommon in the thoracic spine. Chance fractures are flexion-distraction fractures commonly associated with thoracic hyperflexion injuries, and typically result in vertebral body, pedicle, and lamina splitting (Fig. 14–9). They most commonly occur in the lower thoracic and lumbar spine. These fractures are frequently unstable, requiring surgical fixation.65 Depending on canal compromise, there are varying degrees of associated spinal cord injuries with Chance fractures, but patients are often neurologically intact. As Chance fractures are high-velocity type injuries, visceral injuries in the abdomen are frequently associated and require further investigation.66,67
The L1 vertebra is a significant bony landmark in the lumbar spine, as the conus medullaris typically lies just inferior to this segment. Due to the close relationship between the conus medullaris and the L1 vertebra, spinal cord injuries in the lumbar spine are most frequently caused by burst fractures. L1 burst fractures are associated with conus medullaris or cauda equina syndromes (Table 14–1). Injuries below the L1 are associated with nerve root injuries, as only the cauda equina is located within the spinal canal at these levels (Fig. 14–10).
Figure 14–10
(A, B) Sagittal and axial CT imaging of an L3 burst fracture in patient who fell off a ladder while intoxicated. These burst fractures are caused by direct axial force, such as landing on both feet. Transitional junctions, such as the cervicothoracic and thoracolumbar regions are particularly vulnerable to this axial loading force. In this fracture there is approximately 85% spinal canal compromise due to retropulsed bone fragments, especially on the right side. Burst fractures at the cauda equina level are more forgiving in terms of neurological function and this patient was neurologically intact. Patients presenting with neurological deficits or bladder dysfunction and diminished rectal tone are candidates for early decompression. Treatment for thoracolumbar burst fractures (operative vs nonoperative) remains controversial in neurologically intact patients. This patient underwent surgical decompression and stabilization. (Reproduced with permission from Oropello JM, Mistry N, Ullman JS. Spinal Injuries. In: Hall JB, Schmidt GA, Kress JP, eds. Principles of Critical Care, 4e New York, NY: McGraw-Hill; 2014.)

Conus Medullaris | Cauda Equina | |
Distribution, Location | Bilateral and symmetric; perineum or thighs | Unilateral or asymmetric; back, perineum, thighs, or legs |
Radicular Pain | Less common, less prominent | Common severe |
Sensory | Perianal and/or saddle anesthesia | Saddle anesthesia |
Motor | Less effected | Marked weakness, may develop atrophy |
Reflexes | Preserved patellar but absent Achilles | May have absent patellar and Achilles |
Bladder, Bowel | Early on in course, marked incontinence | Late in course, less prominent incontinence |
Impotence | Frequently occurs | Less common |
Originally developed by Denis, the three-column model of spinal stability helps classify injuries as stable or unstable.69,70 The three-column model divides the spine into anterior, middle, and posterior columns. Anterior column elements include the anterior two-thirds of the vertebral body, the corresponding anterior intervertebral disk, and the anterior longitudinal ligament. The middle column consists of the posterior one-third of the vertebral body, the posterior intervertebral disk, and the posterior longitudinal ligament. Lastly, the posterior column is composed of the laminae, pedicles, facet joints, facet joint capsule, ligamentum flavum, supraspinous ligament, and interspinous ligament. Generally, if there is disruption in two of three columns or the middle column, the spine is unstable and likely requires surgical intervention for stabilization69,70 (Fig. 14–11).
Central cord syndrome generally occurs in the cervical spine and is characterized by weakness primarily affecting the upper extremities more than the lower extremities and usually spares the sacral nerves.61 It is the most common of all incomplete spinal cord injuries, comprising approximately 50%.
Brown-Sequard syndrome is most commonly seen in the cervical or thoracic spine. The most common traumatic cause is a penetrating trauma to the spinal canal such as a stab wound or gunshot wound resulting in hemisection of the spinal cord and is characterized by asymmetric paresis with contralateral sensory impairment.62
Anterior cord syndrome can occur at any spinal level and develops as a compromise to the anterior spinal artery supplying the anterior two-thirds of the spinal cord. It is most commonly caused by vascular injury or occlusion of the anterior spinal artery,63 direct injury to the anterior spinal cord, or bone fragments or retropulsed discs impeding arterial flow.64 The anterior horn cells are most affected, causing a loss of motor function below the level of the injury as well as loss of pain and temperature sensation but sparing function of the posterior columns. In nontraumatic cases, anterior cord syndrome may develop from cardiac or vascular surgery that involves clamping of the aorta above the renal artery (Fig. 14–12).
Cauda equina and conus medullaris lesions at the terminal aspect of the spinal cord are often difficult to distinguish due to the overlapping of associated symptoms, which are detailed in Table 14–1. Cauda equina injuries have a better prognosis for recovery, as affected nerve roots are more resilient to injury than the conus and have the possibility of regeneration.
A significant majority of spinal cord injuries are caused by motor vehicle accidents, falls, violence/intentionally inflicted trauma, and sporting injuries. While the primary responsibility of the first responders is to ensure adequate airway, breathing, and circulation, care should be taken to avoid excessive hyperextension of the cervical spine, particularly in the unresponsive person. Studies show that up to 10% of unresponsive motor vehicle accident victims are diagnosed with a cervical spinal cord injury.71 When possible, a rigid cervical collar should be applied to the person at the scene of injury prior to extrication from the wreckage, followed by immediate manual inline stabilization and placement along a standard rigid spine board for transfer to the nearest appropriate acute hospital.72 If the person was injured while playing football, protective equipment (helmet, pads) should remain in place until arrival in the acute hospital setting73; however, the facemask should be removed immediately in case of rapid respiratory decline.74 Transfer to the nearest specialized trauma center should be accomplished in the fastest way possible, by way of ambulance or helicopter if needed.
Upon initial arrival in the emergency room, most trauma patients undergo a focused assessment with sonography for trauma (FAST) survey, which is a quick assessment for internal damage especially useful in hemodynamically unstable patients. Within the first few hours of injury, a surge of catecholamines produced by the initial stressor causes a period of hypertension. As these catecholamines wane, a period of spinal shock can occur. This phenomenon is defined as a temporary loss of spinal reflexes caused by complete or near-complete spinal cord injury at the level of damage and below. Physical exam findings include flaccid muscle tone and loss of deep tendon reflexes.75 Differential diagnosis of spinal shock includes neurogenic shock, characterized by hypotension, bradycardia, and hypothermia. Both spinal shock and neurogenic shock can be present in the acute traumatic phase of injury, particularly if the SCI is at or above the level of T6. This is thought to be a result of reduced sympathetic outflow, leaving the parasympathetic nervous system responses unopposed. Loss of sympathetic output leads to inability to control vasogenic tone, further contributing to the inappropriate hypotension found in this population. In patients with injury above T1, the vagus nerve can precipitously drop the heart rate despite profound volume loss and impaired vascular tone causing reduced cardiac output76,77 (Fig. 14–13).
Figure 14–13
The sympathetic autonomic innervation of the cardiovascular system arises from the high thoracic (T1-T6) and cervical regions, below the parasympathetics that arise at the brainstem level. (Reprinted with permission from Furlan JC, Fehlings MG. Cardiovascular complications after acute spinal cord injury: pathophysiology, diagnosis, and management. Neurosurg Focus. 2008;25(5):E13.)

Adequate volume resuscitation should be directed by the urinary output, with a goal of 30 mL of urine per hour. An indwelling Foley catheter is essential in monitoring urine output and will be required, as the detrusor muscle of the bladder is affected by spinal shock, causing flaccid paralysis and urinary retention. Intra-arterial pressure monitoring becomes essential in determining overall volume status, guiding clinicians in medical management. Maintenance of the mean arterial pressure (MAP) at greater than 85 mm Hg has been shown to improve neurologic outcomes in individuals with cervical and thoracic cord injuries and is discussed later in this chapter.
With stabilization of vital signs, further investigation should be done regarding the neurologic injury with an attempt to assess for spinal cord level of deficits. This can be difficult in the patient who arrives to the emergency room intubated or sedated by the first responders. If safe to do so, sedatives should be weaned to allow adequate neurologic assessment. If the patient is not intubated, a forced vital capacity (FVC) should be measured as soon as possible to assess for potential impending respiratory demise. An FVC of greater than 1.5 liters indicates adequate inspiratory muscle strength, while an FVC of less than 1 liter is an indicator of imminent need of intubation. An FVC between 1 and 1.5 liters is considered borderline and requires close monitoring for any signs of distress.
Because trauma and spinal cord injuries can occur in tandem with traumatic brain injuries (TBIs), initial emergency assessment should including screening for TBI. Use of the Glasgow Coma Scale and/or the Galveston Orientation and Amnesia Test (GOAT) can be used in the acute setting.
Current trauma guidelines recommend computerized tomography (CT) scanning of the spinal column when spinal cord injury is suspected.80 Trauma patients typically receive anteroposterior and lateral x-ray images of additional areas of suspicion to rule out long-bone fractures or other injuries. As mentioned previously in the epidemiology section, up to 47% of spinal trauma patients and 65% of spinal cord injury patients will have a concomitant TBI or orthopedic fractures, warranting further imaging of the brain and skeletal system.81 It is important to remember that the patient may be insensate or unreliable in responses; therefore, imaging may be the only modality to reveal other fractures or brain injuries. If radiographic studies reveal a spinal cord injury, the surgical team must then decide if/when to proceed with operative intervention.
Upon initial injury, the spine is evaluated to determine if surgery is indicated to either stabilize the bony column or decompress the spinal cord in an attempt to preserve or improve function. Several preclinical and clinical trials have demonstrated improved outcomes of early surgical intervention in traumatic SCI. In a meta-analysis performed by Van Middendorp in 2013, “early” (24 to 72 hours depending on the study) spinal surgery was associated with significantly higher total motor score improvement and shorter length of stay when compared to “late” surgery.82 Liu et al performed a similar meta-analysis in 2016 but defined “early” surgery as within 24 hours and “late” surgery as equal to or greater than 24 hours from the time of injury, and the authors found statistically significant differences between these two groups for improvement in total motor score, neurologic improvement rate, length of hospital stay, and complications, all favoring surgery within 24 hours.83 Surgical Timing in Acute Spinal Cord Injury (STASCIS) found that 19.8% of patients undergoing early surgery (mean of 14.2 hours) demonstrated at least a two-grade improvement in AIS motor score compared to 8.8% in the late decompression group (mean of 48.3 hours).85 To further support use of early decompressive surgery, based on results of STASCIS, Furlan found a cost saving and minor gain in quality of life for early decompression in both motor complete and motor incomplete traumatic SCI.86
Despite the evidence supporting earlier surgical intervention, there still remains a delay to operation for some patients. In STASCIS, the 42% undergoing late surgery were found to have a significantly older mean age and higher proportion of less severe injuries. Similarly, in a 2016 study published by Wilson, only 53.3% of patients had surgery within 24 hours, with barriers found to be older age and increased number of stops at intermediate health care centers.87 Other factors that have been found to delay care include comorbidities of the patient, fall-related SCI etiology, and no clear standardized algorithm for management of SCI.87
There are many variables to consider when choosing the appropriate type of surgical intervention, including understanding the mechanism of SCI, location of compromise, and the surgeon’s expertise with specific surgical techniques. Decompressive and stabilization surgery can be done by either an anterior or posterior approach, with decision based on neuronal elements involved, ease of access, and risk of complications. An anterior approach allows for easier accessibility to bone fragments or disk material in the spinal canal, but the posterior approach is considered technically easier and safer to avoid surrounding neurovascular structures. A spinal orthosis may be prescribed for 6 to 12 weeks postoperatively, with duration or use based on surgeon’s preference, technique used for stabilization, or subsequent radiographic evidence of bony fusion.
Not all injuries require surgery. In cases of spinal stenosis and a fall where there is an acute hyperextension/flexion injury but no evidence for ongoing nerve compression or functional decline, management is usually nonoperative. In traumatic SCI due to a penetrating injury such as a gunshot, surgery is rarely indicated, as the spine is typically stable and removal of the penetrating object may cause more damage. However, in these cases if there is evidence for progressive neurologic decline, then spinal surgery would be indicated.
The use of steroids after acute traumatic SCI is still debated. In the 1970s, Hall and Baker found an intense, but short-lived, increase in synaptic discharges in cats following methylprednisolone (MP).89 Further cat studies in the 1980s proposed several potential mechanisms of MP’s action, including altering tissue lactate and pyruvate metabolism, decreasing lipid peroxidation, enhancing sodium-potassium-ATPase activity, hyperpolarizing motor neuron resting membrane potentials, and accelerating impulse conduction along the motor axon.90–92 In 1981, Means et al demonstrated significantly greater recovery of neurologic function and smaller lesion volume in cats treated with MP after induced SCI when compared with no treatment.93
In 1984, the first of three studies known as National Acute Spinal Cord Injury Study (NASCIS) was published by Bracken. The NASCIS trials have received significant publicity but ultimately have failed to demonstrate a clinical advantage for the use of MP in setting of SCI. NASCIS 1 suggested a higher incidence of adverse events in the MP group and no significant difference in neurologic outcomes.94 NASCIS 2 did demonstrate improved motor and sensory function but again supported a higher complication rate and failed to show a change in mortality.95 The conclusions from NASCIS 3 support the use of MP for 24 hours after injury if initiated within 3 hours, and treatment for 48 hours if initiated within 3 to 8 hours of the SCI, but with the risk of a higher complication rate.96 Despite the controversial clinical impact of these studies, a 2006 survey of spine surgeons revealed that the majority (90.5%) of respondents continue to follow the protocol established in NASCIS 3 but that only 24.1% do so due to a belief in improved clinical outcomes.97 In 2013, the American Association of Neurological Surgeons and Congress of Neurological Surgeons stated there is insufficient evidence to support treatment standards or guidelines with corticosteroids but that they could be considered as an option understanding the potential side effects have been demonstrated as more consistent than the clinical benefit.98
Hypotension from acute blood loss, neurogenic shock, and/or other comorbid injuries associated with the initial trauma is common following acute SCI. Higher levels of SCI often have more severe hypotension due to loss of sympathetic-induced peripheral vasoconstriction. Penetrating trauma is often associated with major blood loss as the cause of hypotension. It is important to maintain adequate blood pressure to prevent cord hypoperfusion and potential further damage. The blood pressure needed to prevent further cord injury remains unknown. The current recommendation from the American Association of Neurologic Surgeons is a goal MAP of 85 to 90 mm Hg in the first 7 days after acute SCI. This recommendation is based largely on an uncontrolled study by Vale and associates, in which a MAP of 85 mm Hg for a minimum of 7 days in acute SCI had reported favorable outcomes,78 and on a study by Hawryluk in 2015 demonstrating higher rate of AIS improvements in those with the highest MAPs in the first 24 to 72 hours.90
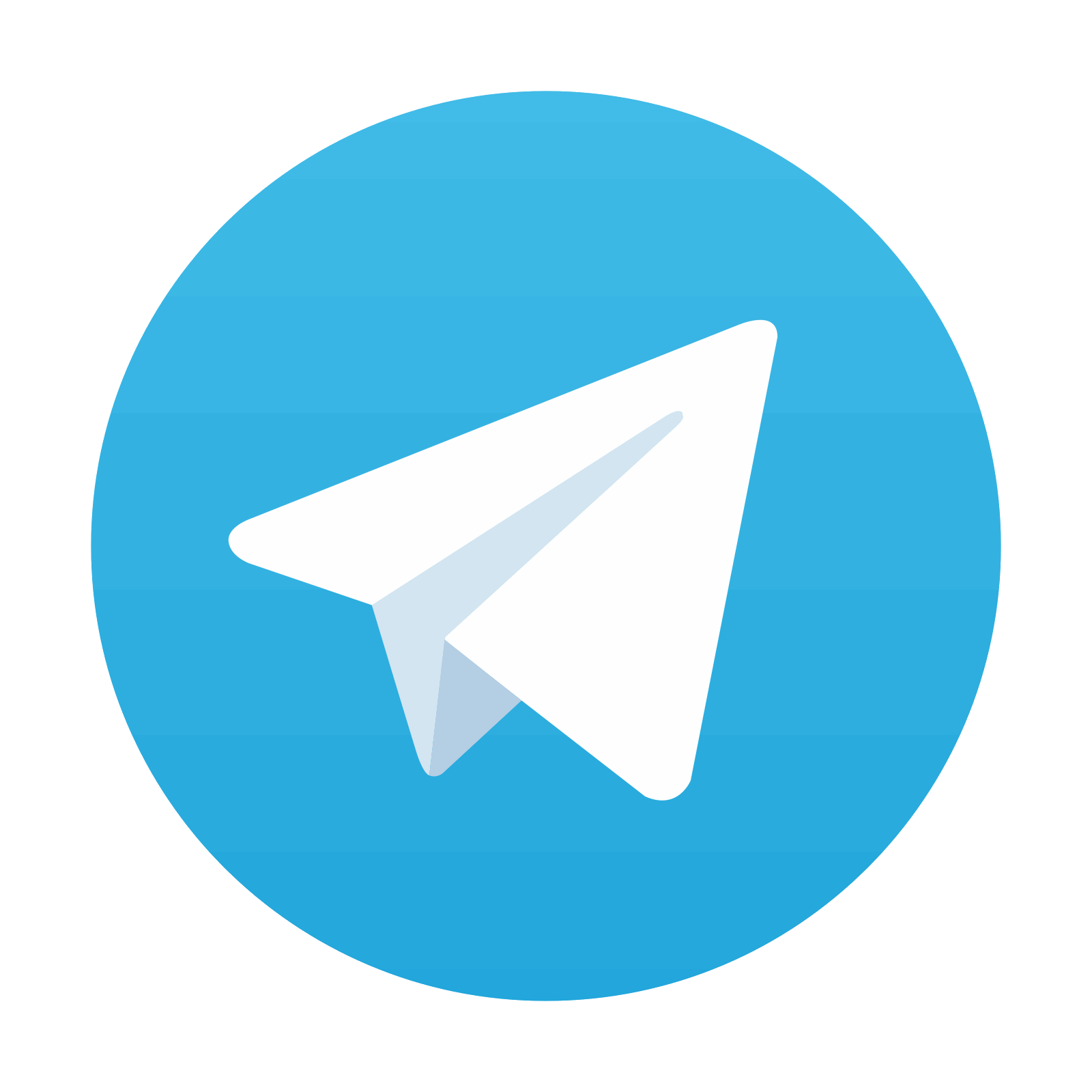
Stay updated, free articles. Join our Telegram channel

Full access? Get Clinical Tree
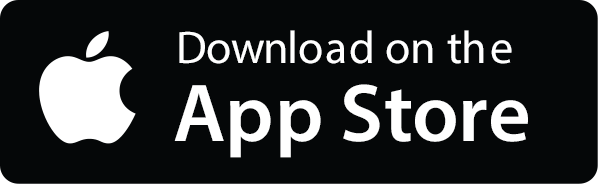
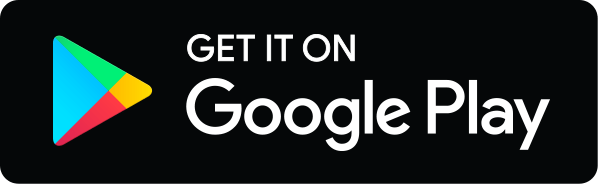