A Note About Common Data Elements for Traumatic Brain Injury Research
In recent years, the National Institute for Neurological Disorders and Stroke (NINDS) has funded interagency efforts to streamline research trials and move science forward via the formation of international panels of experts. These Working Groups have been charged with generating consensus definitions and Common Data Element (CDE) recommendations for use with all clinical trials, as well as for specific disorders or diseases. The purpose of a CDE is to facilitate the clear reporting, interpretation, and comparison of research across the growing international literature in this field of study. As a part of this project, the International and Interagency Initiative toward CDEs for Research on Traumatic Brain Injury (TBI) was formed to develop consensus definitions and CDEs for use in research regarding TBI. Overview information regarding the NINDS CDE project can be found at http://commondataelements.ninds.nih.gov.easyaccess1.lib.cuhk.edu.hk/ProjReview.aspx#tab=References . Current recommendations regarding data standards for clinical research in TBI can be found at www.commondataelements.ninds.nih.gov/tbi.aspx#tab=Data_Standards . We refer the reader to these web resources and the growing literature for additional reading. Definitions and information presented in this chapter will reflect CDE guidelines as indicated.
Definitions
Traumatic Brain Injury
TBI is defined as “an alteration in brain function, or other evidence of brain pathology, caused by an external force.” These types of injuries result from a jolt or blow to the head or are caused by an object penetrating the skull and injuring the brain. Examples include motor vehicle accidents, falls, assaults, or gunshot wounds. TBI should be differentiated from other types of acquired injuries to the brain, such as those caused by tumor or stroke, because of significant differences in mechanisms, treatments, and outcomes between traumatic and nontraumatic injury. TBI should also be differentiated from “head injury,” which is defined as a blow to the head or laceration that may occur without causing injury to the brain. A TBI can be further defined as either open or closed. An open, or penetrating, TBI occurs when the head is hit by an object that breaks the skull and enters the brain. A closed TBI occurs when the brain is injured but the skull remains intact. A diagnosis of TBI is often established through an evaluation of clinical symptoms, as well as positive neurologic signs and neuroimaging findings.
Defining Severity of Injury
TBI is often categorized as mild, moderate, or severe. The Glasgow Coma Scale (GCS)539 is the gold standard for primary initial assessment of severity of injury based on level of consciousness ( Box 43-1 ). During the initial stages of diagnosis in the field or emergency facility, TBI is suspected or indicated if there has been a significant blow to the head and/or there is an alteration or loss of consciousness at the time of injury. An initial GCS score is obtained through clinical evaluation. As indicated in Box 43-1 , the score is obtained by rating the best visual, verbal, and motor responses. The total score is simply a sum of these ratings, with scores ranging from 3 to 15. Coded notations may indicate that the total score, or specific subscale score, has been impacted by additional injury-related characteristics, such as medically induced chemical paralysis caused by intubation, substance intoxication, hypoxia, or other circumstances.
Eyes | Open spontaneously | 4 |
Open to verbal command | 3 | |
Open to painful stimuli | 2 | |
No response | 1 | |
Verbal Response | Oriented and converses | 5 |
Disoriented and converses | 4 | |
Inappropriate words | 3 | |
Incomprehensible sounds | 2 | |
No response | 1 | |
Motor Response | Obeys verbal commands | 6 |
Responds to painful stimuli by: | ||
purposeful localization | 5 | |
withdrawal | 4 | |
flexor posturing | 3 | |
extensor, posturing | 2 | |
no response | 1 | |
GCS Score | 3 to 15 |
Generally accepted guidelines identify three levels of severity based on GCS scores: mild (GCS = 13 to 15), moderate (GCS = 9 to 12), and severe (GCS = 3 to 8). Individuals scoring in the mild TBI range should be evaluated at an emergency facility, but may require minimal or no hospitalization. For more severe injuries involving loss of consciousness, the GCS is a widely accepted measure indicating the depth of coma, and it is generally serially measured until emergence from coma. However, the GCS has limited applicability to young children. The Pediatric Glasgow Coma Scale (PGCS) is a modified version of the GCS for use with pediatric patients with TBI.
Severity of injury is also defined by the duration of loss of consciousness/coma and the severity of symptoms. Individuals with mild TBI may experience brief loss of consciousness as well as transient confusion and neurologic symptoms. They may also experience a brief period of posttraumatic amnesia (PTA). PTA is generally characterized by a loss of memory for events surrounding the injury, disorientation, confusion, and significant cognitive impairment. Although there has been some discrepancy in the literature regarding the diagnosis of mild TBI, some general consensus has been established and will be discussed later in this chapter. The terms complicated mild injury and postconcussive syndrome are often used to refer to cases where diagnosis falls in the mild range; however, additional signs or symptoms of injury are present. Examples may include situations in which positive neuroimaging findings indicate injury to the brain and/or symptoms such as headache, dizziness, and cognitive dysfunction persist for a significant period following mild injury. Individuals sustaining moderate to severe TBI often have a more prolonged loss of consciousness or coma. Upon emerging from coma, they may initially be inconsistent in responding to environmental stimuli. As they become increasingly able to respond consistently, they may continue to exhibit symptoms of PTA for a significant period of time. Increased duration of coma and PTA have been associated with poorer prognosis and outcomes (discussed further in the outcomes section of this chapter). Some individuals with severe injuries do not regain consciousness, remaining in a vegetative state, although others emerge only to a minimally conscious state. The evaluation and care for individuals along the spectrum of injury and recovery will also be discussed in later sections of this chapter.
Epidemiology
United States and Worldwide (Overall)
Approximately 1.7 million TBIs occur each year in the United States. Of these, approximately 52,000 result in death and 1.365 million are mild injuries (often referred to as concussions) for which individuals are treated and released from the emergency room. This leaves approximately 275,000 individuals who survive moderate to severe TBI, generally requiring significant medical care and hospital stays. Many are left with long-term disability as a result of these injuries. According to one study, 40% of individuals hospitalized because of TBI reported at least one ongoing issue at 1 year following injury, with improvement of memory and problem solving listed as one of the most frequently unmet needs. Such cognitive impairments, in addition to physical and medical issues, have significant impact on an individual’s ability to perform everyday activities. In fact, the Centers for Disease Control and Prevention estimates that at least 5.3 million Americans currently need long-term or lifelong assistance with activities of daily living (ADLs) as a result of TBI.
Globally, TBI is a leading cause of death and disability. Although exact worldwide statistics are difficult to obtain, a fairly recent review estimated approximately 775,000 new TBI hospitalizations per year in Europe. Available information suggests that the epidemiology of TBI on a global scale is similar to trends found in the United States. Incidences of TBI by age and gender vary slightly across regions, but overall appear to follow patterns similar to those found in the United States. For example, the distribution of mild, moderate, and severe TBI is consistent across the United States, Europe, Australia, and Asia: 80% of injuries are mild TBI, although moderate and severe TBI each account for 10% of injuries.
Causes of Injury
From 2002 to 2006, the leading causes of TBI in the United States included falls (35%), traffic-related crashes (17%), struck by/against events (sports) (17%), assaults (10%), and other injuries (21%). Sports injuries account for 1.6 to 3.8 million TBIs a year, although most of these are mild injuries that are not treated in the hospital or emergency department. It should be noted that these statistics vary by age, gender, geographic location within and between countries, and group membership because of multiple factors, as explained below.
What is known of the global etiology of injury is similar to U.S. rates, with a few notable exceptions. Sixty percent of TBIs in Europe are caused by road traffic injuries. This figure is distributed evenly across most regions, suggesting that road traffic-related injuries are not associated only with high income countries. Twenty to thirty percent of injuries are caused by falls, 10% violence, and 10% sports or work related. India has the highest reported rate of TBI related to falls. Latin America, the Caribbean, and Sub-Saharan Africa have the highest reported TBI rates because of road traffic incidents and violence.
Associated Costs
The costs of TBI in terms of medical care and lost productivity in the United States were estimated to total $60 billion in 2000. Worldwide statistics are less easily obtained. However, the costs associated with TBI go well beyond the financial requirements for medical care. The societal or indirect costs associated with potential loss of productive work-years for those who have been injured, as well as family members or others who may need to care for them, must also be considered. TBI also results in significant alterations in personal roles and responsibilities for the injured individual as well as family members. Reduced participation in complex leisure or recreational activities is also often a personal cost associated with TBI.
Demographics/Risk Factors
Age and Gender.
The two age groups most at risk for sustaining a TBI are 0- to 4-year-olds and 15- to 19-year-olds. Motor vehicle accidents result in the greatest number of TBI injuries for people age 15 to 19. Another group at risk for TBI is adults over the age of 75. Fall-related injuries are highest among adults over the age of 75, and recent data suggest a 20% to 25% increase in trauma center admissions for TBI. Patients over the age of 75 who sustain a TBI have the highest rates of TBI-related hospitalization and death. Men are twice as likely as women to sustain a TBI in the civilian population. However, in the older population with TBI (age >65 years), women are increasing in number. As the population continues to age, age-specific considerations for treatment and management of those surviving their injuries will be of increasing importance.
Socioeconomic Status.
Lower socioeconomic status (SES) in American cities is correlated with an increased risk of injury because of increased exposure to high-risk occupations, personal violence, older vehicles, and substandard housing. Road traffic injuries are highest in areas of low SES. Socioeconomic disadvantages are often associated with racial characteristics and rural locations. Minority populations in urban US areas are often of lower SES and have elevated risk for injury. African Americans have a higher rate of TBI and associated mortality than other groups.
Statistically speaking, rural populations display a greater incidence of unhealthy lifestyle behaviors including high alcohol consumption and psychosocial stress, and these populations are less likely to participate in preventative measures such as wearing seatbelts. This increase in risk-taking behavior is evident in poorer health outcomes, specifically with injury-related outcomes like TBI. In urban areas, nearly half of adolescents who enter the institutional correction system have a history of TBI, with a calculated incidence of 3107 TBIs per 100,000 person-years. Other studies show that over 60% of prison inmates self-report a previous history of TBI.
Violence.
Assault or violence-related injuries account for 11% of TBI. Violence-related TBIs result from firearms, cutting instruments, blunt objects, or assaults such as pushing or hitting. Injury as a result of firearm use is the leading cause of death from TBI. Sixty percent of firearm-related TBI is self-inflicted, 32% results from intentional assault, and 4% is unintentional. People who sustain a TBI caused by a violence-related injury are more likely to be younger, single, male, member of a minority group, and have a history of alcohol abuse.
Child Abuse.
Intentional TBI in children is known by many names, including shaken baby syndrome (SBS) and inflicted childhood neurotrauma. Approximately 1 million children are severely abused each year, most often by parents or child care providers. The majority of perpetrators are male, either the child’s father or a boyfriend of the child’s mother residing in the home. Infants are at greatest risk for sustaining a TBI as a result of violent shaking, although older children are still at risk. One third of these children survive with no consequences, one third sustain permanent injury, and one third die. Risk factors for the occurrence of SBS include maternal factors such as mothers younger than 19 years, education less than 12 years, single marital status, African American or Native American, limited prenatal care, or newborns less than 28 weeks old. Additionally, child abuse rates caused by unintentional injury increase during periods of economic decline. TBI from child abuse tends to occur more frequently in younger children, and children with TBI caused by nonaccidental trauma (abuse) typically have more morbidity and mortality associated with their injuries than children with accidental injuries. International epidemiologic studies demonstrate that TBI from child abuse is now emerging as a significant issue in multiple countries outside of the United States, including China.
Psychosocial Factors.
Part of the reason for the higher incidence of TBI in young males may be a tendency to engage in higher-risk behaviors. In addition, substance use is related to TBI incidence, and up to 50% of individuals injured test positive for alcohol or other substances at the time of injury. Prior psychiatric history is a risk factor for TBI, particularly with those who have a history of anxiety, depression, and conduct disorder.
Military Traumatic Brain Injury Epidemiology.
Members of the U.S. military are an emerging group at risk for sustaining TBI. Walter Reed Army Medical Center reports that 30% of service members evacuated from the field had sustained a TBI between 2003 and 2005. Army service members are relatively young with an average age of 28. Approximately 85% of military service members are male. Military members who sustain a TBI are also more likely to be male since they represent a larger percentage of the military service population. In the military population, approximately 88% of TBIs are classified as mild injuries although the remaining 12% are moderate to severe.
Compared with the civilian population, the Department of Defense reports that the leading causes of military TBIs include blasts (72%), falls (11%), vehicular incidents (6%), injuries caused by fragments (5%), and other injuries (6%). Blast-related injuries are defined as “blast plus” as soldiers are often involved in more than one type of injury at a time, such as an explosive attack followed by a vehicular crash. Among moderate to severe injuries, recent evidence suggests that penetrating TBI is at least as common, if not more common, than closed TBI.
Demographics/Risk Factors (Global).
Global statistics suggest that countries with low to middle income have more risk factors that contribute to TBI. Risk factors vary by country and may depend on the incidence of factors such as poor road design, substandard vehicles, higher rates of violence because of war, and fewer prevention measures. Low to middle income countries also have inadequate health systems to address TBI-related care. Accurate information on demographics and etiology of TBI in low to middle income countries is especially difficult to obtain. Despite this, the burden of TBI is evident worldwide.
Pathophysiology
The pathophysiologic processes associated with TBI are complex and consist of (1) a primary injury that disrupts brain tissue and function at moment of impact; (2) secondary injury through multiple biochemical cascades that propagate cellular dysfunction and lead to cell death; and (3) a chronic degeneration, repair, and regeneration process that occurs over the long term after the injury has occurred. Figure 43-1 depicts the continuation of injury and repair associated with pathophysiology after TBI.

Primary Injury
TBI occurs in conjunction with mechanical forces that cause disruption to the brain tissue. Closed head injuries can occur because of mechanical forces that shear axons as well as forces at the site of the impact or at the point opposite the impact. Contact forces occur when the head is prevented from moving after it is struck. Inertial forces occur when the head is set into motion and results in acceleration.
Inertial forces associated with angular acceleration result in diffuse axonal injury (DAI), a process that causes tensile strains resulting in microscopic disruption of axons, cerebral edema, and neuron disconnection. In angular acceleration, the brain’s center of gravity moves over a center of angulation, or fulcrum, located in the lower to middle cervical region. The severity of DAI depends on the duration, magnitude, direction of the angular acceleration, and associated impact. In severe cases of DAI, more than just superficial axons are affected and injuries affect deeper white matter structures. The gray-white matter junction is also particularly vulnerable to DAI. Midline brain structures, such as the corpus callosum, are often affected by DAI, which is also associated with loss of consciousness and coma. Recovery from DAI is gradual and can be linked to the duration of coma. Violent shaking associated with child abuse generates significant acceleration/deceleration forces, which result in shearing injury to brain tissue, disruption of blood vessels, and retinal bleeding.
Inertial forces associated with translational acceleration result in a head movement that is in line with the brain’s center of gravity. The resulting differential movement of the brain relative to the skull causes focal injuries such as contusions. Cortical contusions often occur on gyral crests, particularly on the undersurface of the frontal and anterior temporal lobes, where bony prominences located in the basilar skull can create tissue and vascular disruption. Contusions may occur under the impact site (coup injury) and result from a rapid change in skull distortion during impact. Contusions remote from the injury site and opposite of the impact are contrecoup injuries and occur because of negative pressure generated from the impact associated with translational acceleration. Patients with significant TBI may have one or more contusions, and deficits observed with cerebral contusions are linked with the lesion location and size.
DAI and focal contusions can result in neuronal disconnection, or diaschisis. First proposed by Constantin von Monakow in 1905, the concept of diaschisis refers to neurons remote from a site of injury, but anatomically connected to the damaged area, becoming functionally depressed. Because every structure in the brain is directly or indirectly connected to all other structures, the basic idea of this concept is that damage to one structure will be disruptive to others. Imaging studies suggest that diaschisis can result in the metabolic depression of areas both regionally associated with and remote from the site of injury. Others suggest that focal cortical lesions may affect contralateral cortical functioning by way of intercallosal connections and can affect the function of subcortical structures including the striatum. Resolution of diaschisis is one of several theories for recovery of function to be discussed later.
Epidural hematomas (EDHs) result from local impact and subsequent laceration of underlying dural veins and arteries. The meningeal artery is commonly the source of EDHs, and damage to dural sinuses can also cause EDH. When an EDH develops from a disrupted artery, a neurologic emergency occurs as the EDH quickly expands and rapidly causes neurologic deterioration. Subdural hematomas (SDHs) result from inertial forces and the tearing of bridging veins. Bridging veins are susceptible to shear and rupture from brief high-velocity angular accelerations associated with falls. Traumatic subarachnoid hemorrhage (SAH) occurs when angular acceleration shears vessels located in the subarachnoid space.
Secondary Injury
Secondary injury develops over the hours and days after the initial impact and is associated with disruption of cerebral blood flow and metabolism, massive release of neurochemicals, cerebral edema, and disruption of ion homeostasis leading to cellular injury and eventual cell death. Much of what is known about secondary injury is derived from postmortem analyses of human brain tissues, the analysis of blood, cerebral spinal fluid (CSF), and parenchymal microdialysate fluid obtained early after clinical TBI. Additionally, much work exploring secondary injury has used in vivo experimental models of TBI and in vitro injury methods. Experimental models of TBI produce one or more types of primary injury, which lead to the development of secondary injury cascades that closely resemble observations made in humans.
Brain swelling occurs in response to the initial injury and early events involved with secondary injury and results in elevated intracranial pressure (ICP) and decreased cerebral perfusion pressure (CPP). If severe enough, brain swelling can lead to herniation, which has potentially fatal consequences. In some cases, elevated ICP is caused by the development of focal extraaxial lesions like SDH and EDH described above. In other cases, elevated ICP and intracranial hypertension result from global mechanisms occurring on a cellular level that lead to brain edema. Some mechanisms that affect brain edema include vasogenic edema, increased tissue osmolarity, and vascular dysregulation resulting from increased cerebral blood volume. However, brain edema caused by neuronal swelling and astrocyte swelling secondary to increased glutamate metabolism after excitotoxic insult may impart the most damage.
Excitatory amino acids (EAAs) like glutamate and aspartate are ubiquitous and important neurotransmitters for normal brain function. After TBI, excess excitatory amino acid levels are present in extracellular microdialysate fluid as well as CSF. Excess EAA release occurs, in part, from the mechanical stresses associated with stretch injury of axons. Elevations in extracellular glutamate levels after TBI contribute to excitotoxic injury by affecting other paths of secondary head injury through two primary mechanisms. By binding their target receptors, excess EAAs can trigger an influx of sodium and chloride that leads to acute neuronal and astrocytic swelling. Excess EAA levels can also lead to an intracellular calcium influx as well as the release of Ca ++ from intracellular stores leading to delayed cellular damage or death. EAA-mediated Ca ++ -dependent production of nitric oxide, superoxide, and free radical damage to DNA and cellular membranes leads to cell death. Regional differences in the distribution of EAA sensitive receptors contribute to selective vulnerability of some regions to excitotoxic injury like the hippocampus, a critical region for learning and memory.
Lactate is the end product of glycolysis, and in neurons, lactate is converted to pyruvate for oxidative metabolism. Lactate/pyruvate (L/P) ratios reflect the cellular energy balance and the cytosolic oxidation-reduction state. Increased metabolic demand after TBI is due, in part, to increased astrocytic glutamate uptake and results in increased lactate. Reduced cerebral blood flow, including pericontusional hypoperfusion, has been documented in both experimental models and in humans early after TBI and results in ischemic injury. Elevations in L/P ratios have been measured in pericontusional tissue in humans and are associated with other aspects of secondary injury, including increased ICP and mitochondrial dysfunction.
Although extracellular lactate has classically been a marker for anaerobic metabolism with hypoxic injury, research also indicates that lactate is used by brain tissue during an insult as an important energy source in oxidative metabolism. Evidence supports a dichotomous role of lactate in which it is produced by the astrocyte via glycolytic metabolism, to be directly consumed by the neuron after uptake through lactate transporters. Physiologically and after TBI, EAAs are likely triggers for this metabolic process. After injury, estradiol facilitates lactate production for neuronal use by modulating lactate transporters. Interestingly, experimental studies suggest that lactate is used as a neuronal energy substrate after TBI and lactate administration after TBI is neuroprotective and decreases cognitive deficits.
Mitochondrial dysfunction is often the result of energy failure and excitotoxic insult and results in the formation of highly reactive free radicals and oxidative damage to cell membranes and DNA. Hydroxyl radicals and other reactive oxygen species react with many molecules found in living cells, including DNA, membrane lipids, and carbohydrates. CNS tissue is particularly vulnerable to oxidative injury caused by the high rate of oxidative metabolic activity, the nonreplicating nature of neurons, high levels of transition metals, and a high membrane-to-cytoplasm ratio in neurons. Superoxide radicals are a principal mediator of the microvascular damage after TBI. Free-reactive iron generated from hemorrhage catalyzes the formation of free radicals. Reactive oxygen species production may also be a downstream effect of caspase-mediated cell death (apoptosis).
Cell death in CNS tissues after brain trauma falls into two primary categories: (1) cellular necrosis and (2) apoptosis or programmed cell death. Necrotic cells display cellular and nuclear swelling as well as disruption of cellular membranes. In contrast, apoptotic cells are characterized by cellular shrinkage, nuclear condensation, and DNA fragmentation. Apoptosis is accompanied by a cascade of events required for cell death to occur. In TBI, cell death patterns often reflect a combination of both necrosis and apoptosis.
Apoptosis can be initiated through either extracellular or intracellular signaling pathways. c ysteine-aspartic acid prote ases (caspases) play an essential role in cell death via both pathways. Extracellular binding of cell surface death receptors, including tumor necrosis factor alpha (TNFα) receptor and FAS ligand receptor, initiates apoptotic signals, including caspase-8 and caspase-3, within seconds of ligand binding. Intracellular signaling is initiated by mitochondrial dysfunction in response to disturbances in cellular energy balance, oxidative injury, and changes in ionic homeostasis. This mitochondrial stress leads to the opening of the mitochondrial transition pore and cytochrome C release. Cytosolic cytochrome C release induces other caspases, including caspase-9 and caspase-3, which leads to the cleavage of key cellular elements like cytoskeletal proteins and DNA repair proteins, as well as activation of endonucleases. In addition to this intracellular caspase-dependent process for apoptosis, intracellular apoptosis signaling can also occur via a caspase-independent pathway with apoptosis inducing factor (AIF). AIF is released from the mitochondria and results in DNA fragmentation. The mitochondria house both prosurvival and pro-death proteins that are both members of the BCL-2 protein family, each of which can be manipulated experimentally to affect cell survival. As an example, estrogen enhances BCL-2 expression and cell survival after central nervous system (CNS) injury.
Inflammation after TBI is characterized by very complex cascades that largely include cytokines, or signaling molecules that communicate with resident and infiltrating inflammatory cells. There is growing evidence to implicate cytokines, including IL-1β and TNFα, in propagating the inflammatory response, as well as in promoting neurotoxicity and edema via excitotoxicity and oxidative injury. Inflammatory molecules are synthesized by peripheral cells as well as CNS neurons and glia and are increased early after injury. IL-1β has potent leukocyte-signaling properties that lead to cellular inflammatory responses after TBI, and IL-1β and other inflammatory mediators can facilitate blood brain barrier (BBB) disruption and transport of peripheral inflammatory components into the CNS. Multiple inflammatory mediators are also synthesized by activated microglia in the CNS. Specifically, IL-1β and TNFα can mediate synthesis of neurotoxic compounds like arachidonic acid and are implicated in excitoxic components of secondary injury.
Cytokines are constitutively expressed in the brain and promote neuronal differentiation and survival under normal conditions. Cytokines play a dual role in secondary injury with TBI, and inflammatory cascades may either enhance cellular injury or provide neuroprotection. Some studies suggest that cytokine’s effects may be dose-dependent, with higher levels more likely to be associated with detrimental effects and lower levels neuroprotective. Consistent with this previous work, recent clinical evidence implicates CNS cortisol as a regulator of this dualistic role after TBI. Also, some cytokines support neuroprotection and neurorepair through their effects on neurotrophin production and excitotoxicity and antiinflammatory properties. CNS inflammatory biomarker profiles can be influenced by extracerebral injury wherein major trauma itself can elicit a significant systemic inflammatory response. Recent evidence suggests that neuroinflammation can continue for months and years after injury, adversely contributing to outcome and implicating TBI as triggering a chronic inflammatory state. Specific cytokine effects in the setting of long-term or chronic inflammation remain largely unstudied; however, some evidence suggests beneficial effects can occur at delayed or chronic time points after injury. TNFα is an example in which time-dependent functions have been demonstrated, with TNFα expression being protective at later time points after injury.
Aside from BCL-2 and the prosurvival components of neuroinflammation, other CNS compounds can serve as endogenous neuroprotectants after TBI. Adenosine is a purine ribonucleoside; it is ubiquitous in the brain, where it largely functions as a major neuroinhibitory molecule. After experimental brain injury, extracellular adenosine levels increase by 50- to 100-fold when compared with baseline, a phenomenon that is implicated as an endogenous neuroprotective mechanism. Adenosine functions as a neuroinhibitory molecule through its primary target, the A1 receptor. A1 receptor activation is a key local regulator of excitatory amino acid release during ischemia and trauma. Adenosine can also hyperpolarize neuronal membranes and attenuate intracellular Ca ++ accumulation.
Neurotrophins may also have a neuroprotective role after TBI, particularly since early increases in neurotrophin expression have been reported in experimental TBI. The downstream effects of neurotrophins like brain-derived neurotrophic factor (BDNF) may be variable, based on the regional target milieu where increases in the proapoptotic target receptor p75 are observed with acute CNS insult and increased age. Thus, BDNF’s role in TBI recovery and mortality may be dependent on the relative balance of these target receptors. In support of this concept, a recent clinical report implicates BDNF in mortality after TBI, in which there were unique relationships among BDNF genetics, older age, and mortality risk.
Concomitant Injury
TBIs, particularly more severe cases, are not often isolated. High-speed vehicular crashes and active combat frequently result in multiple injuries that can affect outcome. In civilians, associated injuries are linked with more disability even 1 year after the injury. TBI associated with multiple other injuries is also associated with longer acute-care lengths of stay and need for rehabilitation resources. In addition to increased functional deficits, rehabilitation needs, and disability, concomitant injuries can directly affect secondary injury and associated pathology, and these phenomena have received some attention in the TBI literature. For example, experimental models incorporating TBI and hemorrhagic shock show increased hippocampal injury versus TBI alone, a phenomenon likely associated with increased secondary ischemia.
Military Blast Injury
With the ongoing military conflicts in Iraq and Afghanistan, TBI is a major problem for active combat military personnel. The frequent use of improvised explosive devices in these conflicts has resulted in a large number of military personnel (see previous epidemiology sections) sustaining TBI from blast injury. Blast injury can result in both blunt and penetrating trauma and follows a specific nomenclature ( Box 43-2 ). Explosives can be categorized as high-order explosives (HEs) or low-order explosives (LEs), and each can cause a distinct injury pattern. HE produces a supersonic overpressurization shock wave that is associated with “primary injury.” In addition to middle ear damage, brain injury can occur from primary blast injury without overt evidence of injury. The rapid pressure changes generated from the overpressure wave result in shear and stress forces that lead to trauma such as concussion, subdural hematoma, and diffuse axonal injury. Penetrating head injury from flying debris is the result of secondary blast injury. Both HE and LE can result in tertiary blast injury from being physically thrown by a blast wind into the ground or other objects. Quaternary injury includes asphyxia or inhalation of toxic chemicals that may also contribute to brain injury. Many patients have TBI induced from more than one facet of blast injury.
- •
Primary direct effects of overpressure blast
- •
Rupture of tympanic membranes
- •
Concussion
- •
Subdural and diffuse axonal injury
- •
- •
Secondary: penetrating trauma
- •
Fragmentation injuries
- •
- •
Tertiary: effects of structural collapse and of persons being thrown by the blast wind
- •
Open or closed brain injuries
- •
- •
Quaternary: asphyxia, and exposure to toxic inhalants
- •
Concomitant closed injury
- •
Increasingly, research is being conducted to develop relevant blast injury models of TBI to examine and characterize mechanisms of primary and secondary injury for blast TBI and assess behavioral effects. Clinically, difficulties after blast TBI of any severity can include headache, insomnia, decreased memory and attention, slower thinking, irritability, and depression. Additional research is focused on clinical symptom screening and outcomes as well as physiologic markers and biomarkers associated with this type of TBI. A more recent focus is the effects of repetitive blast injury exposure on the development of neurodegenerative disorders and the comorbidity of posttraumatic stress disorder.
Neurotransmission Repair, Regeneration, and Recovery
Acute mechanisms of secondary injury heavily influence the degree and type of injury and deficits that individuals with TBI experience. The chronic period after injury is characterized by multiple neurotransmitter deficits and cellular dysfunction. During this period, however, research demonstrates that the brain is amenable to neuroplasticity, repair, and recovery, and the potential for these processes can be augmented with relevant rehabilitation strategies.
One mechanism by which TBI recovery occurs is through the reversal of diaschisis. Contemporary thought on the concept of diaschisis suggests that the functional effects of diaschisis can be either reversible or permanent. However, the mechanisms by which diaschisis resolves are poorly understood and likely multifactorial. The resolution of cerebral edema and blood flow regulation may contribute to early recovery. Later, the alleviation of diaschisis may involve factors like synaptic plasticity, axonal sprouting, and cortical reorganization. Diaschisis after brain injury can be manipulated by both behavioral experience (rehabilitation) and pharmacologic intervention. For example, depressed thalamic metabolism after cortical infarction has been reversed with administration of neurostimulants such as amphetamine.
Experimental and clinical research suggests that dopamine systems are key pathways involved in attention, task salience, and cognition, and these pathways are impaired chronically after TBI. Alterations in both presynaptic and postsynaptic dopaminergic proteins in the striatum and frontal cortex have been documented, including important proteins involved in dopamine synthesis and uptake. Decreases in striatal dopamine transporter (DAT) expression, a key protein in regulating the extracellular half-life of dopamine, have been documented in humans. Further research demonstrates that striatal neurotransmission is impaired and may be the result of TBI-induced changes in DA protein functionality. Recent clinical research suggests impaired striatal DA transmission, including reduced DAT expression profiles that mirror experimental modeling data (described below) and that are both DAT and D2 genotype specific.
Noradrenergic systems affect arousal, sleep-wake cycles, vigilance, and cognition, and the norepinephrine (NE) transporter appears to affect NE neurotransmission as well as interact with dopamine (DA) systems to affect DA neurotransmission. Noradrenergic function is impaired early after TBI. Previous reports suggest that cortical NE levels are decreased within 24 hours following brain injury, and adrenergic receptors are altered after injury. However, NE augmentation early after TBI is neuroprotective, and NE agonists aid in neurorecovery. Importantly, NE is critical for governing cortical plasticity and facilitating recovery post-TBI. By stimulating adrenergic systems with amphetamine and L-DOPS, a precursor of NE, neuronal damage after TBI can be minimized and sprouting is promoted.
Cholinergic systems are important for memory and cognition, and evidence suggests chronic impairments occur after TBI. Hippocampus expression of the vesicular acetylocholine transporter is increased; however, evoked release of acetylcholine in the neocortex and hippocampus is reduced following experimental TBI. Chronic changes in cholinergic receptor expression patterns and affinities have also been noted experimental TBI models. Further, daily local application of nerve growth factor has been shown to enhance memory and hippocampal neuron function after experimental TBI.
In addition to acute protection and cholinergic function, other studies support a role for neurotrophin administration in promoting long-term recovery. In contrast to acute increases in neurotrophin production, other work suggests that hippocampal BDNF is decreased during chronic recovery from experimental TBI. Although an enriched environment can reverse these decreases, chronic pericontusional administration of BDNF does not improve behavioral outcome or hippocampal cell survival. Studies suggest that BDNF target receptor expression ratios can vary following ischemia and TBI, which can promote apoptotic pathways in the acute phase following experimental TBI. BDNF’s early role in TBI recovery may be dependent on the relative balance of these target receptors. Recent work in our group suggests that genetic variation in the BDNF gene may be related to mortality following TBI. Both intraparenchymal administration of basic fibroblast growth factor and nerve growth factor do reduce cognitive deficits in experimental TBI. With these positive studies, improved recovery may be secondary to stimulation of brain repair and neuroplasticity.
Principles of Experimental Neurotransmitter Dysfunction and Treatment After Traumatic Brain Injury
The neurobiology of catecholaminergic dysfunction, particularly DA dysfunction, is the most characterized in the experimental literature. Early changes in DA levels occur after experimental TBI. Chronic DA receptor and synthetic protein changes after TBI also have been characterized with experimental TBI models. The dopamine transporter (DAT) is a key protein regulating the extracellular half-life of DA in the synaptic cleft and the therapeutic target of neurostimulants like methylphenidate (MPH) and amphetamine. Long-term changes in striatal and cortical DAT expression and intracellular trafficking have been identified that are gender specific. Further, functional changes in DAT after experimental TBI lead to altered striatal neurotransmission. Other changes in postsynaptic secondary signaling mechanisms, as well as changes in presynaptic DA synthetic enzyme levels, have been identified.
Behavioral pharmacology studies show that daily treatment with the DAT inhibitor MPH has a beneficial impact on cognition after experimental TBI. Recent work suggests that the cognitive benefits of MPH are more pronounced in males and that daily MPH therapy has a neurorestorative effect on striatal neurotransmission in males through MPH-mediated changes in DAT function. Daily treatment with the pleiotropic DA enhancer amantadine has been reported, and a recent clinical trial supports amantadine use in severely injured individuals in the postacute and early chronic recovery phases after TBI. Experimental data also show that the D2 receptor agonist bromocriptine can benefit learning and memory. Interestingly, early treatment with bromocriptine also appears to decrease oxidative damage initiated by the injury.
Evidence also suggests that NE systems are disrupted after experimental TBI but can be manipulated to improve recovery. Cortical NE levels are decreased in the first 24 hours after injury, and NE turnover is impaired chronically after injury. Further, α1-adrenergic receptor expression is decreased. Local NE infusions early after injury enhance recovery, and stimulating adrenergic systems with amphetamine can decrease neuronal damage after TBI. Recent studies suggest that at least some of the beneficial effects of amphetamine may be because of its ability to increase neurotrophin production after injury. Daily treatment with low doses of the selective NE transporter (NET) inhibitor atomoxetine shows benefit on cognitive recovery after experimental TBI.
Experimental TBI studies also demonstrate decreased hippocampal cholinergic transmission and alterations in cholinergic synthetic and metabolic enzyme production. For example, a single dose of the acetylcholinesterase inhibitor rivastigmine improves cerebral edema, cognition, and motor functioning early after experimental TBI. Interestingly, daily treatment with CDP-choline, a key intermediary in the biosynthesis of phosphatidylcholine, improves cognitive recovery in experimental TBI and increases hippocampal and cortical acetylcholine levels. However, the beneficial effects of CDP-choline have not translated in a recent large multisite clinical trial involving acute and chronic administration in individuals with complicated mild to severe TBI.
Evaluation and Treatment of Traumatic Brain Injury
Management of Mild Traumatic Brain Injury (Concussion)
Similar to more severe injuries, concussion results in significant alterations in brain physiology. After an initial increase in glucose use, brain metabolism is impaired and is associated with the development of energy crisis, oxidative injury, and initiation of biochemical cell death pathways. These derangements can occur for up to weeks after the concussion and persist even with a normal GCS and general neurologic examination findings.
There are several criteria and scoring systems for classifying degree or severity of mild TBI. However, the World Health Organization (WHO) Collaborating Centre Task Force on Mild TBI states that key criteria for identifying persons with a mild TBI include at least one of the following: confusion, disorientation, loss of consciousness for less than 30 minutes, PTA for less than 24 hours, or other transient focal neurologic abnormalities and a GCS score of 13 to 15 after 30 minutes or presentation to a health care facility. Findings with these criteria should be made in the absence of illicit drugs, alcohol, medications with sedating side effects, or other injuries or problems. Patients with uncomplicated TBI typically do not have associated abnormalities on standard imaging tests like CT. Concussion severity nomenclature has been developed for the purposes of injury characterization and injury management. Some examples of frequently used grading systems include the Cantu and Colorado concussion scales ( Table 43-1 ).
Guideline | Grade 1 | Grade 2 | Grade 3 |
---|---|---|---|
Colorado |
|
| Loss of consciousness (of any duration) |
AAN |
|
|
|
Cantu |
|
|
|
Frequently, patients with concussion will complain of any number of associated symptoms, including memory loss, poor concentration, impaired emotional control, posttraumatic headaches, sleep disorders, fatigue, irritability, dizziness, visual acuity, depression, anxiety, personality changes, and seizures. These problems can affect ADLs, social relationships, and work abilities. For most with mild TBI, symptoms resolve over time. However, a subset of patients will have persistent symptoms classified as postconcussion syndrome and may require outpatient follow-up. The types of symptoms, proportion of patients who have persistent symptoms, and duration of symptoms required to define symptoms as persistent have been a point of debate. Reports of symptom duration to define persistent deficits can range between 3 and 12 months. The fourth edition of the Diagnostic and Statistical Manual of Mental Disorders (DSM-IV) suggests that individuals showing objective evidence of cognitive deficits as well as three or more subjective symptoms that represent a change in baseline function for at least 3 months can be diagnosed with postconcussion disorder. Other classification systems, such as the International Classification of Diseases , Tenth Edition, do not include objective evidence of cognitive deficits. Despite variability in definitions and classification, some studies suggest that females are more likely to have postconcussion syndrome.
Athletes comprise a large portion of the population who sustain mild TBI, and many are children. Special issues arise with this population with regard to “on-site” concussion screening tools, determining the most effective algorithm for return to play, safety equipment use, management and prevention of recurrent injuries, and academic performance. In general, athletes should be free of postconcussive symptoms before returning to competition. One report shows that 30% of high school football players return to play on the same day of injury. However, many return-to-play guidelines recommend that athletes with a grade 1 or 2 concussion have a minimum of 7 days free of symptoms before returning to play. Same-season injuries often occur within 7 to 10 days of the initial injury, a time frame in which there is increased vulnerability to damage with the second insult. Most return-to-play (RTP) algorithms emphasize a graded protocol for reintroducing the athlete to physical exertion, although a recent systematic review does not support graded return-to-sports protocols as significantly influencing prognosis, and conservative management with return to play is still standard of care. Notably, recent studies suggest that academic and cognitive exertion can impact postconcussive symptoms and cognitive testing performance and should be considered when developing RTP protocols for athletes. Recent evidence and expert consensus suggest a stepwise approach to return-to-school guidelines for pediatric concussion. Several guidelines recommend termination of sports participation for the remainder of the season if an athlete sustains a third concussion within that season. However, athletes who have one concussion are at increased risk for subsequent concussion, making return to contact sports even after a single concussion a decision that requires careful thought and consideration.
Acute Medical Management of Moderate to Severe Traumatic Brain Injury
The care of the patient with TBI begins in the field. Evidence-based guidelines from the Brain Trauma Foundation and the American Association of Neurological Surgeons support a regional organized trauma care system that addresses prehospital care and triage. Direct transport of a patient with severe TBI to a level 1 or 2 trauma center is associated with decreased mortality when compared with indirect transport. Facilities defined by the Guidelines for Prehospital Management of Traumatic Brain Injury as being appropriate for transport include those offering CT scanning, neurosurgical care, ICP monitoring, and treatment capabilities. These facilities can provide 24-hour neurosurgical care and intensive care treatment, and ICP monitors are routinely used. Each state can designate trauma facilities based on the availability of such care. Although treatment guidelines for initial management are not specific, the recommendations include complete and rapid physiologic resuscitation. Correction of hypoxia, including intubation, is appropriate. Sedation and neuromuscular blockade may be used to optimize the transport of the head injury patient.
ICP has been the mainstay of monitoring after severe TBI. ICP can be monitored with a ventriculostomy, the procedure of choice, because it allows therapeutic CSF drainage. ICP monitoring is appropriate in those patients with a GCS of 8 or less after resuscitation, head CT with contusions or edema, or systolic blood pressure less than 90 mm Hg. ICP monitoring can also be considered in patients with severe TBI and negative head CT findings if they are older than age 40, posturing, and/or have a systolic blood pressure less than 90 mm Hg. Elevated ICP is defined as 20 to 25 mm Hg in clinical practice. Maneuvers to decrease ICP include elevating the head of the bed 30 degrees, treatment of hyperthermia, mannitol administration, sedation, and brief hyperventilation. Prolonged chronic hyperventilation can negatively impact cerebral blood flow.
CPP is defined as the mean arterial pressure minus ICP and is the pressure gradient driving cerebral blood flow. Studies have documented worsened clinical outcomes in patients with TBI with episodes of low CPP. Current therapeutic recommendations are to maintain CPP above 60 mm Hg in adults. Measurement of cerebral oxygen tension allows the practitioner to directly measure ischemia, rather than rely on indirect measurement of CPP or ICP. Tissue oxygenation monitors, directly placed in brain tissue via an external ventricular drain, can detect focal ischemic changes that may go undetected with global measures. Interventions are then tailored to the cause of the drop in tissue oxygenation. Studies have shown benefit on outcome and mortality reduction with addition of multimodal monitoring.
Other treatment interventions have been studied in acute-care clinical trials. A multicenter trial evaluating the role of corticosteroids in management of acute TBI showed an increase in mortality 2 weeks after TBI when compared with placebo. Elevated CSF cortisol levels in severely injured patients, and their link to inflammation, may contribute to this outcome. Progesterone is a drug with pleiotropic neuroprotective properties identified in several preclinical studies occurring over decades. Recent clinical trials have shown its safety, and progesterone appeared to have therapeutic potential. However, recently, two multi-site clinical trials did not show overall benefit with progesterone therapy. As progesterone is the primary substrate for both stress and sex hormones, each with known adverse influences on secondary injury, progesterone treatment effects on these hormone pathways and secondary injury cascades require further study.
Animal studies have evaluated the protective use of hypothermia, with several showing positive results. Clinical trials with moderate hypothermia have shown some reduction in excitotoxic insult and neurologic impairment; however, results have not been consistent. For instance, a large multicenter trial of hypothermia did not show improved outcomes in patients with severe TBI. To transition this treatment to routine human use, factors such as treatment window, rewarming rates, and type of anesthesia must be defined. In addition, age, sex, and hormone effects on treatment efficacy and physiologic response to treatment should be considered to determine whether specific subgroups with TBI might benefit from this treatment. In contrast, therapeutic hypothermia has been adopted as a part of early treatment for the TBI population, with significant reductions in mortality and neurologic morbidity noted in clinical studies.
Surgical treatment of TBI is indicated when intracerebral fluid collections exert a significant mass effect. Mass effect can be gauged by degree of midline shift on neuroimaging and the decision to intervene may require serial neurologic exam inations and CT scans. Depressed skull fractures greater than the thickness of the skull should be elevated to decrease the risk of infection, especially in the setting of a dural laceration. Surgical treatment may also be used to lower ICP. The use of decompressive craniectomy to decrease ICP is an accepted intervention, although the reduction of morbidity and mortality has not been proven. Such procedures can also lead to additional issues such as herniation of the brain outside the skull bone defects, subdural effusion, and hydrocephalus requiring ventriculoperitoneal shunt.
Secondary complications in the intensive care unit (ICU) can also negatively affect TBI outcomes. Elevated glucose levels are associated with increased mortality after TBI, presumably because of increased lactic acid production leading to ischemia as well as impaired phosphorus metabolism. Recent studies suggest that glucose variability, including low serum glucose levels, may adversely impact outcome. Sodium imbalance can potentiate risks of seizure as well as worsen level of consciousness, as this electrolyte abnormality is directly related to volume status. Hyponatremia can be exacerbated by giving excessive volumes of intravenous fluids. This phenomenon occurs even in the setting of normal saline or lactate Ringer solution used for fluid resuscitation, making electrolyte monitoring and volume status important in preventing secondary complications. Prevention and aggressive treatment of infections are also important, with one recent study indicating that hospital-acquired pneumonia can have significant effects on long-term outcome. Hyperthermia, by increasing ischemia, is another source of acceleration of secondary insults to brain parenchyma. Thus, maintenance of normothermia is often a part of clinical critical care for those with severe injuries.
Early initiation of nutrition is vitally important in the patient with TBI. Metabolic rates can increase 40% during the early postinjury stages. Guidelines recommend replacement of 140% of resting metabolism expenditure (100% in paralyzed patients). The gastrointestinal tract is preferred to maintain nutritional status. The goal of treatment is to maintain a positive nitrogen balance, although this is difficult to achieve. Nitrogen balance via a metabolic cart study, prealbumin levels, and liver function should be followed in the ICU. Although literature does not yet support standard practice guidelines to optimize nutrition after moderate to severe TBI, significant evidence exists suggesting that delayed nutrition replacement is associated with increased mortality. In a metaanalysis, small bowel feeding in the ICU reduced rates of hospital-acquired pneumonia and some immune-enhancing feeding formulas reduced sepsis, suggesting a direct link between nutritional support and acute/chronic inflammation and complications known to adversely impact long-term outcome.
The physiatrist plays a role in evaluating patients early who have sustained TBI. The early assessment includes prevention of orthopedic and immobility complications and contributes to management of behavioral issues (e.g., agitation) and begins early stimulant trials aimed at improving arousal and early participation in therapies. Early consultation can result in improved mobility, improved functional outcomes, and decreased acute-care length of stay. In this model, rehabilitation assessment is a portion of the acute medical care, providing education and support for patients, families, and caregivers.
Physiologic Measurements During Acute Care
Somatosensory-evoked potentials (SSEPs) are recorded from the scalp after stimulation of a sensory or mixed nerve in the peripheral nervous system. SSEPs are often used in severe TBI to predict survival and evaluate posttraumatic coma and persistent vegetative states. In some cases, SEEP testing is used as an adjunct to the clinical examination to determine brain death. Hume and Cant found that bilateral loss of the median SSEP was indicative of impending death, and asymmetrical findings observed in the cortical response were indicative of severe residual deficits. Their study indicated that serial SSEPs often change through the hospital course, and each study does not always correlate well with ultimate outcome. Given increased use of sedatives and paralytics, SSEPs can be a useful adjunct to the clinical examination to aid in prognosis, with recent evidence suggesting a similar role for prognostication in cardiac arrest.
Like other neurologic evaluations, electroencephalography (EEG) can be beneficial when assessing patients with TBI to detect injury severity and depth of coma. Degree of unconsciousness can quickly change, and continuous EEG (cEEG) monitoring can detect electrophysiologic signs of clinical deterioration. Initial EEG recordings taken within 24 hours of injury, however, are generally more abnormal and of less prognostic significance than those performed after 24 to 48 hours. The cEEG is a standard part of care at some level I trauma centers, and it can be used to effectively identify subclinical electrographic seizures, which are associated with other physiologic factors that contribute to poor outcome, including ICP elevations and increases in metabolic stress.
Pupillary reflexes have been used not only as clinical indicators of surgical emergencies and complications in TBI associated with edema and herniation, but also as a tool in determining lesion location, mortality, and global outcome after injury. Presence or absence of pupillary reflexes and degrees of anisocoria have been used in models predicting the probability of intracerebral hematoma and lesion location. The International Mission on Prognosis and Analysis of Randomized Controlled Trials in TBI has demonstrated high predictability of post-TBI mortality/outcome using a core model of age, injury severity (motor subscale of the GCS), pupillary reactivity, and midline shift.
Although not yet used routinely at the bedside, biomarkers have considerable potential as adjunctive tools in TBI diagnosis, prognosis, and monitoring treatment effect. Typically, protein biomarkers that have been studied in TBI include either markers of structural damage or markers reflective of the cellular and molecular cascades observed with secondary injury and repair. Protein biomarkers have also been studied in both CSF and serum. CSF biomarker studies are valuable in that measured levels are a largely a reflection of CNS pathology. For other markers, CSF levels for peripheral markers (e.g., hormones, inflammatory markers) may reflect extent of BBB disruption. However, CSF collection is routine only in severely injured patients who require an extraventricular drain for management of ICP. Thus, serum markers are attractive because of their potential application to mild and severe cases of TBI. However, serum biomarker levels are often limited by the extent of BBB permeability present after the injury. Markers of acute injury have been much more thoroughly studied than markers of chronic injury. Although none are yet used for clinical management, research progress (discussed later) continues to generate novel biomarker discovery and characterization studies that will contribute to their eventual translation to clinical care.
Neuroimaging for Medical Management
The head CT scan is a mainstay of diagnostic imaging in TBI, and findings have use in identifying mass lesions that constitute a neurosurgical emergency and in correlating with outcome. Mass lesions, including SDH and EDH ( Figure 43-2 ), can often cause shifting of the midline structures and can be life-threatening if not surgically evacuated ( Figure 43-3 ). Intraparenchymal contusions can also cause shifting of midline structures ( Figure 43-4 ). A DAI is often difficult to appreciate on head CT unless it is severe in nature with associated small hemorrhages forming near sheared axons ( Figure 43-5 ). In mild TBI, the head CT is often negative; however, other modalities may capture subtle pathology associated with less severe injuries.




CT is the current standard neuroimaging modality for the initial evaluation of patients with suspected moderate-to-severe TBI. CT methods, which are based on the same principles as plain-film x-rays, are advantageous as they allow for rapid, noninvasive three-dimensional imaging, which accurately detects facial and skull fractures, as well as acute hemorrhaging and mass effect. CT allows for optimal medical management of trauma patients who may require immediate surgical intervention. Repeat CT scans may be used to evaluate recovery or to monitor for additional complications. For example, if a patient with TBI does not progress as expected or declines in neurologic status, repeat CT scans are often used to diagnose issues such as new or evolving bleeding or hydrocephalus. Because of their high clinical use and relatively low cost, CT scanners have become readily available for use with TBI evaluation. As with x-ray, there are some risks associated with ionizing radiation, which require clinical judgment and a risk/benefit analysis when considering multiple CT evaluations over time, particularly with the evaluation of certain higher risk groups, such as pregnant women and children.
Less than 10% of mild TBI cases have positive CT findings, and even less, 1%, require neurosurgery. As one primary purpose for conducting the initial CT is to determine the potential need for early neurosurgical intervention, these statistics have resulted in significant debate regarding the appropriate use of CT in cases of mild TBI. The New Orleans Criteria and the Canadian CT Head Rule indicate that CTs should be obtained in cases of mild head trauma, if specific additional criteria suggesting the possibility of more severe or evolving neurologic injury are met. Examples include age and symptoms such as seizure, vomiting, headache, and significant PTA. The American College of Emergency Physicians put forth guidelines for clinical decision making based on available evidence in 2002 with an update in 2008 that were also consistent with and included the recommendations from the Canadian and New Orleans groups. A recent study has suggested that the Canadian CT Head Rule may have higher sensitivity than the New Orleans Criteria, with higher negative predictive value. However, additional study is needed regarding the impact of using one method over another.
MRI is a second method of structural neuroimaging that has demonstrated clinical use in TBI. MRI can produce high-resolution structural brain images by exploiting the magnetic properties of hydrogen atoms, and variations in the density and water content of body tissues. In comparison with CT, MRI scans take longer to complete and are more susceptible to motion artifacts. This makes it more difficult to scan individuals who are agitated. In addition, MRI is less able to detect skull fracture and acute blood than CT. Because of the magnetic environment of the MRI scanner, it also may be more complicated to scan patients with the continuous clinical monitoring and treatment equipment required during acute care or with medically implanted devices or objects that may be affected by the magnet (e.g., pacemakers, aneurysm clips, plates, rods). However, many MRI-compatible instruments and surgical implants have become available, reducing the frequency of this issue. MRI may also be contraindicated when there are causes of injury such as gunshot wounds or other accidents involving the presence of metal fragments. MRI does have superior resolution to CT, and it provides much higher detail in soft tissues. Therefore, MRI becomes the methodology of choice for evaluating brainstem and frontal area injuries and for detecting small hemorrhages and nonhemorrhagic white matter injury. As a result, MRI becomes increasingly useful with time from trauma, when patients become more medically stable and additional diagnoses of DAI or other small areas of hemorrhage may be helpful for treatment and prognosis.
The four types of MRI sequences currently considered to be standard for the evaluation of TBI are T1- and T2-weighted, diffusion-weighted imaging (DWI), and T2*-weighted gradient echo imaging. T1-weighted imaging is generally used to attain anatomic maps of the brain. T2-weighted images and T2 fluid-attenuated inversion recovery (T2 FLAIR) sequences are primary additional sequences for conventional MRI scanning to evaluate for injury. T2 images are sensitive to changes in water content indicative of edema, as well as iron content indicating the presence of blood. FLAIR sequences suppress the signal from CSF, resulting in improved visualization of cortical and periventricular lesions, as well as nonhemorrhagic shear injury associated with DAI. T2-weighted gradient recalled echo (GRE) has additional sensitivity to blood breakdown products, further adding to its sensitivity for DAI. DWI is useful for detecting acute ischemia/microchanges via sensitivity to micromovement of water molecules, but not as sensitive for evaluation of larger hemorrhagic lesions. As MRI images can be obtained in sagittal, coronal, and axial planes without moving the patient, additional nonconventional MRI sequences can be helpful. For example, coronal and sagittal FLAIR images can be helpful at discerning DAI involving the fornix and corpus callosum, compared with routine axial T2-weighted images.
Recent efforts to develop CDEs for TBI have included imaging protocol recommendations for both basic imaging and more advanced modalities depending on the imaging and research capabilities of clinical sites. These modalities will be discussed further in the research section of this chapter, as they are not currently used as standard clinical care, although the growing research literature provides evidence of significant translational potential for the use of these methods to inform clinical decision making.
Management of Patients with Disorders of Consciousness
Some patients with severe TBI have prolonged periods of depressed consciousness that may never resolve. Their accurate classification is vital for appropriate prognostication. Numerous terms have been used to classify these different states. Coma is defined as a state of pathologic unconsciousness in which the eyes remain closed and there is no evidence of purposeful motor activity. Vegetative state typically follows a period of coma, in which there is some evidence of wakefulness, in the form of eye opening, without any sustained or reproducible responses to the environment. Vegetative states should be described with the length of time since injury. The terms persistent and permanent should not be used. A minimally conscious state (MCS) is the condition of severely altered consciousness in which there is definite, reproducible evidence of self or environmental awareness. The behaviors examined include command following, intelligible verbalization, recognizable yes/no responses, and movements or emotional responses triggered by environmental stimuli.
Functional neuroimaging has been useful to understand the neuropathology of depressed consciousness, especially in defining those patients who are classified as MCS or may have a higher likelihood of recovery of communication or functional object use. Both functional MRI and positron emission tomography (PET) provide correlates of the differences between vegetative state and MCS. In PET studies, patients with clinical evidence of vegetative state have reductions of overall cerebral metabolism of 50% or greater. In comparison, patients with MCS have been shown to have activation of appropriate cerebral networks in response to environmental stimuli, although the activation may not be the same as in normal control subjects with novel or complex stimuli. Connections between the medial parietal, frontal, and thalamus have been defined as the networks most affected in disorders of consciousness, leading to focus on these areas with functional neuroimaging. Although neuroimaging may eventually be used to discriminate between vegetative state and MCS, it currently does not have a role in clinical diagnosis or prognostication.
The current evaluation of patients with depressed levels of consciousness includes a thorough neurologic examination, including pupillary responses, brainstem reflexes, and ocular movements. Brainstem reflexes commonly elicited include corneal responses, gag reflex, and oculocephalic reflexes, referred to as doll’s eyes. These reflexes show integrity of brainstem pathways, with reproducible behavioral responses to specific areas of sensory stimulation. Also recorded are the direct and consensual pupillary responses as well as a response to visual threat. Observation of spontaneous activity and responses to environmental stimuli is important. Common mistakes include attributing purposefulness to responses that are reflexive, allowing insufficient observation time for patient response to a stimulus, and underconsideration or overconsideration of family observation of behavior. To best observe behaviors, it is important to optimize environmental conditions and patient positioning and avoid sedating medications. Standardized rating scales are available to differentiate vegetative state from MCS. These scales allow for detecting subtle improvements and can offer inter-rater and test-retest reliability when incorporated into daily clinical testing.
A variety of interventions have been studied in these patients. The use of sensory enrichment in both naturally occurring events and in the administration of multimodal sensory stimuli has been reported in case studies and retrospective data analyses. The effectiveness of these techniques is not clear, although there is no report of harm associated with sensory stimulation. Pharmacologic interventions can be used to promote arousal and behavioral persistence. Medications frequently include psychostimulants, dopamine agonists, and tricyclic antidepressants. Bromocriptine, a dopamine agonist, was studied in five patients in a vegetative state. They had greater physical and cognitive recovery at 12 months compared with historic control subjects. However, this study lacked a large sample size and did not address the possibility of spontaneous recovery. Based on reports of efficacy of zolpidem, a sedative-hypnotic medication, prospective open-label trials were conducted to assess efficacy in patients with chronic disorders of consciousness (DOC). No clinically significant improvement in functional object use was seen, although there were changes in the Coma Recovery Score. Recent trials have investigated the implantation of electrodes in the brainstem and thalamus to stimulate the reticular system and arousal. Although studies are limited, behavioral changes were observed in some patients.
Behavioral Measures of Responding and Cognition
Decisions regarding readiness to transfer a patient from ICU to step-down units and to inpatient rehabilitation are generally based on medical stability and progress, as well as the ability to respond to the environment and to actively participate in therapy. Several behavioral measures are useful in determining the status of a patient’s emergence from coma and progress through the acute phases of recovery from injury.
Emergence from Coma
Evaluation of functioning during the initial stages of emergence from coma generally involves the monitoring and serial assessment of a patient’s ability to respond to external stimuli. This is often done through the use of a standardized measure of responsiveness, such as the Coma Near Coma (CNC) scale. This 11-item scale was designed to measure small changes in response in patients with severe brain injuries functioning at a low level. An additional scale is the JFK Coma Recovery Scale-Revised (CRS-R), which is a 23-item scale used to assess functioning in six areas: auditory, visual, motor, oromotor, communication, and arousal. Instruments such as the CNC and CRS-R are generally useful during acute-care hospitalization or, at times, if an individual who is minimally responsive is transferred to the inpatient rehabilitation setting or another longer-term care facility.
Evaluation of Posttraumatic Amnesia
As an individual emerges from coma and is able to respond to verbal inquiry, instruments such as the Galveston Orientation and Amnesia Test (GOAT) or the Orientation Log (O-Log) are used to track progress through the PTA phase of recovery. Both of these instruments essentially measure and score serial responses to orientation questions and allow for objective scoring of the patient’s responses. Emergence from PTA is then defined by the consistent attainment of a score on one of these instruments indicating orientation.
Although rehabilitation scales, such as the GOAT and O-Log, generally focus on disorientation and amnestic symptoms, other elements of confusion (i.e., delirium and psychiatric types of symptoms) have also been noted during the acute phase of TBI recovery. There is increasing awareness of the need to measure these symptoms and to develop instruments that bridge gaps between scales traditionally used to measure PTA and those traditionally used to measure delirium. One example is the Neurobehavioral Rating Scale, which combines portions of the Brief Psychiatric Rating Scale with additional items to measure other psychiatric symptoms associated with TBI. The Confusion Assessment Protocol focuses more on delirium than other scales.
The Ranchos Levels of Cognitive Functioning Scale, commonly referred to as the Ranchos scale, is widely accepted to describe the process of cognitive recovery as an individual emerges from coma, then progresses towards emergence from posttraumatic amnesia/delirium, and emerges to near normal cognitive functioning ( Table 43-2 ). This scale presents recovery as a progression through eight typical stages. It has been widely adopted to assess patient functioning for purposes of rehabilitation planning and treatment and to explain patient progress to families.
Score | Description |
---|---|
_______ (1) | Level I—No Response. Patient does not respond to external stimuli and appears asleep. |
_______ (2) | Level II—Generalized Response. Patient reacts to external stimuli in nonspecific, inconsistent, and nonpurposeful manner with stereotypic and limited responses. |
_______ (3) | Level III—Localized Response. Patient responds specifically and inconsistently with delays to stimuli, but may follow simple commands for motor action. |
_______ (4) | Level IV—Confused, Agitated Response. Patient exhibits bizarre, nonpurposeful, incoherent, or inappropriate behaviors, has no short-term recall, and attention is short and nonselective. |
_______ (5) | Level V—Confused, Inappropriate, Nonagitated Response. Patient gives random, fragmented, and nonpurposeful responses to complex or unstructured stimuli. Simple commands are followed consistently, memory and selective attention are impaired, and new information is not retained. |
________ (6) | Level VI—Confused, Appropriate Response. Patient gives context-appropriate, goal-directed responses, dependent on external input for direction. There is carry-over for relearned, but not for new tasks, and recent memory problems persist. |
________ (7) | Level VI—Automatic, Appropriate Response. Patient behaves appropriately in familiar settings, performs daily routines automatically, and shows carry-over for new learning at lower than normal rates. Patient initiates social interactions, but judgment remains impaired. |
________ (8) | Level VIII—Purposeful, Appropriate Response. Patient is oriented and responds to the environment but abstract reasoning abilities are decreased relative to premorbid levels. |
Further information as well as rating forms for the Confusion Assessment Protocol, CNC, CRS-R, GOAT, O-Log, and LCFS and may be found on the “scales” page of the Center for Outcome Measurement in Brain Injury website ( www.tbims.org/combi/index.html ).
Inpatient Rehabilitation Assessment and Management
The focus of inpatient rehabilitation following TBI is to assist each patient in improving functional independence. Ideally, with good recovery, each will return home and be successful in eventual community reentry. The basic rehabilitation team comprises a multidisciplinary group of specialists including a physiatrist, physical therapists (PTs), occupational therapists (OTs), speech language pathologists (SLPs), and other hospital staff including nursing and case management. As cognitive and behavioral issues associated with TBI may pose unique challenges to the provision of rehabilitation treatment, ideally all members of the team should be specially trained to work with patients who have sustained TBI. Neuropsychologists are also often included as key members of the team.
Role of the Neuropsychologist in Inpatient Rehabilitation
The role of the neuropsychologist is multifaceted. He or she provides ongoing assessment of recovery of cognitive function and works with therapy and nursing staff to integrate information about cognitive abilities and limitations into implementation of effective and appropriate rehabilitation goals for the patient. Ideally, the neuropsychologist is available to observe and cotreat with other therapists as needed. Neuropsychologists have specialized training in brain-behavior relationships and expertise in the testing and evaluation of cognition. They also apply that expertise to the evaluation of interactions between cognitive strengths/limitations and behavior. As a result, they are generally experts in behavior management and may often assist with organization and implementation of behavior plans for patients as needed. This role is often particularly relevant on TBI units, where agitation related to recovery from TBI may threaten or impede patient progress. In some settings, psychologists function both as neuro and rehabilitation psychologists. Rehabilitation psychology focuses on adjustment to disability and other personal, social, and situational issues in an effort to assist individuals with all types of disabilities in working toward maintaining healthy and satisfying lifestyles.
Medical Rehabilitation Evaluation, Complications, and Management
During the initial evaluation of a new patient on the inpatient rehabilitation unit by a physiatrist or rehabilitation specialist, a thorough neurologic examination should be conducted. This often includes traditional physical examination components described elsewhere in this textbook. Often, depending on a patient’s medical status, a complete evaluation cannot be conducted at first attempt and may need to be completed at a later date. As awareness and communication improve, it is not uncommon to discover additional injuries that were previously missed because of the patient’s inability to express pain or participate in assessment.
The clear documentation of concurrent fractures or other orthopedic injury is important to allow safe treatment in a rehabilitation setting. Physical examination measures include the description of motor weakness, abnormal muscle tone, and presence or absence of contractures. For those patients with a decreased level of consciousness and cognitive disorders, it is vital to perform serial measures to document level of awareness. Multiple instruments (GOAT, GCS, CNC, CRS-R, as described previously) can be performed serially by physicians and therapists. The evaluation should also include documentation of brainstem reflexes such as pupillary reflexes, doll’s eyes, and response to caloric stimulation.
Although some degree of medical stability has been achieved by the time a patient has been transferred from ICU to inpatient rehabilitation, medical complications can and do occur while patients are on the rehabilitation unit. The next sections describe some medical issues and complications of particular concern for individuals with a recent history of TBI. Assessment and feedback from members of the rehabilitation therapy team often provide information vital to the diagnosis of these medical issues.
Posttraumatic Seizures.
Posttraumatic seizures (PTSs) are a significant complication arising from TBI. PTS has commonly been defined as occurring in the immediate period (<24 hours after injury), early period (24 hours to 7 days after injury), and late period (>7 days after injury). Late PTS can also be defined, based on the 2014 criteria from the International League Against Epilepsy, as posttraumatic epilepsy (PTE). PTS accounts for 20% of symptomatic seizures and 5% of all seizures in the general population. Up to 86% of patients with one seizure after TBI will have a second within 2 years of their injury. Moreover, PTE is associated with increased disability and limitations with ADLs. Although the neurobiology of PTE is not well understood, neuroinhibitory molecules like adenosine and GABA afford relative protection from this complication. In fact, genetic variability within the ubiquitous adenosine A1 receptor has been linked with risk for PTS occurring within the first week and also with the development of PTE. Further, genetic variation in the glutamic acid decarboxylase gene also contributes genetic risk to PTE development. Conversely, excitotoxicity likely contributes to the development of PTE. PTS occurrence is related to injury severity. A recent report suggests that acute inflammatory burden for IL-1β and genetic variation within the gene coding for IL-1β may accelerate epileptogenesis and development of PTE. Other reported risk factors include biparietal contusions, dural penetration with bone and metal fragments, multiple intracranial operations, cortical contusions, subdural hematoma, significant midline shift, early PTS, and skull fractures.
Phenytoin is commonly used for early prophylaxis against PTS development and for treatment of PTS. It acts by diminishing excitatory tone and augmenting inhibitory neurotransmitter systems in cortical and subcortical structures. Phenytoin therapy for 1 week provides protection against early PTS. Notably, published treatment guidelines for phenytoin are now over 10 years old and are based on recommendations from a (seminal) clinical trial published over 20 years ago showing that patients with severe TBI should receive prophylactic treatment with phenytoin for 7 days after injury. The Association of American Neurologists (AAN) still recommends early intervention with phenytoin (intravenously) given as a loading dose as soon as possible followed by a 7-day course in the asymptomatic moderate-to-severely brain-injured population. Phenytoin use beyond this first postinjury week was not supported, and both clinical and experimental studies suggest adverse effects on recovery with prolonged phenytoin use. Newer drugs, including the synaptic vesicle protein 2A (SV2A) modulator levetiracetam, which do not require active monitoring of drug levels, are growing in use for PTS prevention. However, research on their efficacy in preventing PTS specifically is limited. Recent small-size clinical reports suggest that levetiracetam use during the first postinjury week has similar efficacy as phenytoin, but additional studies are needed to confirm this.
Despite recommendations supporting limited treatment for seizure prophylaxis, people with TBI often receive PTS prophylaxis on a long-term basis with anticonvulsant medications that require regular monitoring and are often associated with unwanted side effects, including sedation. Patients who go on to develop PTE also experience similar issues with long-term treatment. Recent evidence with an experimental model of TBI demonstrates that long-term treatment with phenytoin after injury leads to increased cell death and poorer function on cognitive behavioral tasks. This work further supports following current guidelines for PTS prophylaxis with phenytoin and minimizing prolonged therapy when possible. However, there is recent work suggesting that daily treatment with levetiracetam for 3 weeks postinjury has multiple neuroprotective effects and improves multiple neurobehavioral outcomes in a rat model of TBI, a finding that is consistent with neuroprotective characteristics of this drug in other studies.
Heterotopic Ossification.
Heterotopic ossification (HO) ( Figure 43-6 ), a common complication occurring after TBI, is a poorly understood process by which ectopic bone is formed outside the skeleton. Incidence of HO after TBI ranges from 11% to 28%. Incidence may be higher in those sustaining military-related blast injuries, where TBI and traumatic amputation, both of which increase the risk of HO development, often co-occur. Patients with more severe TBI, as well as associated immobility, spasticity, and fractures, seem to be at relatively greater risk for this complication. Dysautonomia is also linked with higher HO risk. Among acute-care patients undergoing rehabilitation for TBI, HO is considered a risk factor for poorer outcomes and decreased home discharge rates. Blood markers associated with bone metabolism, such as alkaline phosphatase and osteocalcin, are nonspecific for HO. X-rays can identify HO in more advanced cases. However, bone scan is more sensitive in identifying early and asymptomatic cases.

Although the pathophysiology of HO after TBI is poorly understood, evidence suggests that CNS processes facilitate HO bone formation. Bone remodeling is subject to central control through the sympathetic nervous system, which is partially regulated by the endocannabinoid system. After experimental TBI, cannabinoid-mediated downregulation of norepinephrine at osteoblasts contributes to increased bone formation. Other research suggests that humeral factors in human CSF after TBI are osteoinductive. However, specific factors have not been identified. Leptin, a metabolic protein, is also a potential mediator of HO through both central and peripheral mechanisms.
Common prophylactic methods include antiinflammatory medications such as indomethacin, irradiation, and Ca ++ binding chelating agents, e.g., etidronate. NSAIDS are effective in preventing HO formation in total joint replacement patients, and mounting evidence suggests that NSAIDS are a useful preventative in spinal cord injury (SCI). Less is known about the specific effects of NSAIDS on HO after TBI. At this time, two systematic reviews failed to provide adequate research evidence to support these therapies for acute HO treatment. Once HO formation occurs, it can significantly impact joint range of motion and mobility. Areas of HO formation take several months to mature. After maturation is complete, excision of the ectopic bone can improve joint motion and mobility, and studies suggest that surgical excision is the most effective therapy for HO after TBI.
Deep Venous Thrombosis.
Deep venous thrombosis (DVT) is a significant source of morbidity and risk of mortality for patients with TBI. In patients with severe TBI, pulmonary embolus secondary to DVT is an important cause of death, and the estimated incidence of DVT is 40%. The effectiveness of routine screening for clot at rehabilitation admission has not been proven, and there is no standard of care for initiation of DVT prophylaxis and treatment in patients with TBI. Increasing evidence supports the safe use of either heparin or low-molecular-weight heparin within 24 to 72 hours after severe TBI or intracranial bleed. This decision can be made in consultation with the neurosurgeon about the risk of rebleed after review of neuroimaging studies.
Patients at highest risk for DVT include those at advanced age, severe injury, prolonged immobilization, significant fractures, and presence of clotting disorder. Unfortunately, patient behavior, including fall risk, agitation, and ability to be provided with a safe environment, might prevent one from taking a risk with anticoagulation or even prophylaxis. In those patients who cannot undergo pharmacologic prophylaxis of DVT because of risk of bleeding, mechanical compression devices can be used. Although their efficacy has never been completely studied, their effectiveness in clinical practice is likely to be lower than that in controlled trials. If they are used, it is important they are used consistently and for the maximum hours each day and appropriately sized. For patients with agitation or requiring restraints, tolerance of these devices may be low. The length of DVT prophylaxis is not clearly defined in the TBI population. Among general rehabilitation patients, the ability to ambulate greater than 100 feet is an important milestone to discontinue prophylaxis.
Swallowing/Nutrition.
Moderate-to-severe TBI is associated with specific nutritional needs. Patients demonstrate increased caloric requirements caused by hypermetabolism, increased energy expenditure, and increased protein loss. Early institution of enteral nutritional support may decrease morbidity and mortality, shorten hospital length of stay, and potentially improve immune function. Nutritional status monitoring can include review of laboratory tests and weights, and in more complex patients, metabolic cart studies. Clinical dieticians are important team members in this regard. Caloric supplementation is not the only focus of nutritional support. Data support the role of nutriceutical supplementation, including probiotics to decrease risk of infection as well as vitamin D, omega-3, and zinc supplementation for their potential benefits. All need to be further clarified through specific studies.
The route of nutritional support, gastrostomy versus jejunostomy, is not resolved. Factors that lead to the decision for a more permanent form of enteral nutrition include whether nasogastric nutrition can be consistently maintained, if the location of the tube is an agitating factor, and potential length of time nonoral nutrition is needed. Once a permanent tube is placed, it is expected to be in place for more than 30 days to decrease complications associated with removal. Whether to place a tube directly in the stomach versus more distally in the jejunum should be based on presence of gastroesophageal reflux. In those patients who will require nonoral nutrition when they go home, the gastric tube allows for bolus feeding, which better approximates natural feeding.
As the patient’s level of alertness improves, swallowing evaluation can include a bedside swallowing assessment or video fluoroscopy. Because normal gag reflexes and good cough reflex are not completely predictive of normal swallow function, video fluoroscopy often identifies silent aspiration and improves clinical decision making when relaxing dietary restrictions. Even after video fluoroscopy, institution of oral nutrition is often done in a stepwise manner, evaluating the effects of behavior on feeding, as well as variations in level of arousal and fatigue during a day.
Bowel and Bladder Dysfunction.
Loss of bladder and bowel control is common after TBI. Injury to cortical and subcortical structures can lead to loss of control over these functions or discoordination of sphincter management. The early incidence of urinary incontinence after significant TBI is approximately 62%. Patterns of dysfunction include an uninhibited overactive bladder, as well as poor perception of bladder fullness and poor sphincter control. During acute care and rehabilitation, urinary tract infections are common, impacting functional outcome on discharge, skin integrity, and increasing risk of discharge to long-term care. Treatment options include behavioral interventions such as timed voiding. Caution must be used in initiating anticholinergic medications, because of their adverse cognitive effects.
Bowel dysfunction after TBI includes incontinence and constipation. Constipation is common, because of lack of mobility, use of constipating medications, and dietary influences. Bowel programs include stool softeners, stimulant suppositories, and hydration. Bowel incontinence is often associated with more severe injury and poorer functional status. It may also be caused by diarrhea, frequently seen with the use of enteral feeds or infectious causes.
Airway/Pulmonary Management.
Pulmonary complications after TBI may be directly related to the effects of trauma, including pneumothorax, hemothorax, flail chest, and rib fractures. Neurologic level of injury may lead to respiratory failure, pulmonary edema, and airway complications. Pneumonia is the most common complication observed in acute care and rehabilitation, occurring in 60% of patients. The presence of respiratory failure and the need for tracheostomy have a strong relationship with acute care and rehabilitation length of stay. They are also associated with functional status on the Disability Rating Scale and the Functional Independence Measure 1 year after injury. Early tracheostomy (≤8 days) is associated with shorter mechanical ventilation and shorter hospital stay but not hospital mortality. It may be a way of decreasing hospital morbidity and shortening time to initiation of acute rehabilitation although that has not been specifically addressed.
The majority of patients with TBI who need a tracheostomy in acute care regain sufficient pulmonary and neurologic function later to undergo decannulation. This can be considered when patients no longer require ventilation, can manage their secretions, and are at low risk for aspiration. Usually, decannulation is achieved by serially decreasing the cannula diameter and then by capping the tube. Before capping the tracheostomy, a speaking valve can be used to allow phonation through the open tracheostomy. This is generally only tolerated with a smaller-diameter tracheostomy, and the cuff on the tracheostomy must be deflated for safety. If this step is used, it is often recommended that the valve is only placed by trained staff after they have verified that the cuff is deflated. Tolerance is measured by ability to maintain oxygen saturations and speak clear, long sentences without signs of breathlessness. If the patient tolerates these steps, the tracheostomy may be removed. Visualization of the airway before decannulation via laryngoscopy can assess for tracheal stenosis, subglottic stenosis, glottic stenosis, and tracheal granuloma. These tracheal abnormalities are associated with a high risk of respiratory difficulties requiring reintubation and possible surgical intervention.
Decision making in decannulation of patients at Rancho levels 2 and 3 is more difficult. Poor pulmonary toilet and variable central respiratory status in this group led to pneumonia and sepsis and increased morbidity and mortality with decannulation. Predictors of successful decannulation include younger age, alert cognitive status, and adequate swallowing and cough reflexes. One should consider whether decannulation will make care easier or more difficult in the next level of care. Also, timing of decannulation is important; it is more troublesome in the winter when respiratory illnesses are common.
Spasticity and Contractures.
Spasticity is a common problem observed with upper motor neuron damage such as that occurring with TBI. It is one portion of the upper motor neuron syndrome that contributes to motor dysfunction in these patients. Spasticity is clinically defined as a velocity-dependent increase in tonic stretch reflexes with exaggerated tendon jerk responses. Other components of the upper motor syndrome include loss of autonomic control, decreased dexterity, and limb weakness, which also often occurs in these patients. Although the incidence in the TBI population is generally unknown, among those with injuries severe enough to require inpatient rehabilitation, it has been reported to be as high as 84%.
After significant damage to central motor pathways as occurs with TBI, acute paralysis often occurs. This damage leaves the affected muscles and joint immobilized. Immobilization leads to reduction in longitudinal tension in a muscle, the basis for muscle contracture. In animal models, only 24 hours of unloading of tension in a muscle caused a 60% shortening of muscle fiber length. Over the next few weeks, both plastic neural and muscular reorganization leads to muscle overactivity defined as spasticity. This further aggravates the development of muscle and joint contractures.
The early identification of contracture development and spasticity is critical. The risk factors for spasticity development include more severe injury (lower GCS), motor dysfunction (hemiplegia or tetraplegia), associated anoxic injury, spinal cord injury, and age. Development can occur as early as days after injury but is more classically observed months later. Patient evaluation includes a clinical and functional history, stretch reflex examination, range of motion assessment, and an active motor examination. Measurement of degree of passive abnormal muscle tone is often done with the Ashworth scale or modified Ashworth scale ( Table 43-3 ). The modified Ashworth scale assigns 0 to 4 points based on the amount of resistance measured by an evaluator when attempting to range a joint through an available range of motion. The Tardieu scale is a true measure of spasticity, comparing the differences noted when a muscle is stretched at different velocities and comparing the angles at which the catch is noted ( Table 43-4 ).
Score | Description |
---|---|
0 | No increase in muscle tone |
1 | Slight increase in muscle tone manifested by a catch and release at end range of motion |
1+ | Slight increase in muscle tone, manifested by a catch Followed by minimal resistance throughout the remainder of the range of motion |
2 | More marked increase in tone, through most of the range of motion but joint easily moved |
3 | Considerable increase in muscle tone, passive movement is difficult |
4 | Affected part is rigid in flexion or extension |
Score | Description |
---|---|
0 | No resistance throughout the course of the passive movement |
1 | Slight resistance throughout the course of the passive movement with no clear catch at a precise angle |
2 | Clear catch at a precise angle, interrupting the passive movement followed by release |
3 | Fatigable clonus, less than 10 seconds when maintaining pressure, appearing at a precise angle |
4 | Nonfatigable clonus, more than 10 seconds when maintaining pressure, at a precise angle |
The cornerstone of spasticity treatment is the use of static and dynamic splinting devices. When used in combination with passive range of motion exercises, splints serve to lengthen muscles and tendons that are shortening. Although controlled trials of the use of passive range of motion are few, the pathophysiology of spasticity and clinical experience have guided their use. A stretching program can also be easily taught to family members and transitioned to a home environment. In combination with static splinting such as serial casting, measures of spasticity can decrease. In a setting with close monitoring for adverse effects such as pressure sore development, serial casting can be very efficacious after TBI. Caution must be used in initiating it while patients are still experiencing high ICPs. Physical modalities, such as cryotherapy, superficial heat, and ultrasound, can be used in concert with splinting or pharmacotherapy. Multiple forms of electrical stimulation have been studied in the management of spasticity, with positive results identified in some placebo-controlled studies.
Systemic pharmacologic agents may also be used. Dantrolene is indicated for the treatment of spasticity of CNS origin, including TBI. It acts at the sarcoplasmic reticulum by inhibiting calcium activity. Dantrolene has been associated with hepatic toxicity and requires regular monitoring of liver enzymes. Baclofen acts both presynaptically and postsynaptically at the GABA B receptors, effectively inhibiting spinal reflexes. Side effects include fatigue, sedation, weakness, hallucinations, and lowering of the seizure threshold. When studied in a TBI population, lower extremity Ashworth scores were improved, but a similar effect was not obtained in the upper extremity. Somnolence limited maximum dose in 17% of subjects. Other oral agents for spasticity include benzodiazepines, tizanidine, and clonidine. Somnolence and cognitive effects often limit the usefulness of these medications in the TBI population, and some worsen recovery in experimental models.
Focal chemodenervation can be an important tool for practitioners, without concerns for cognitive side effects. Phenol used for either motor point block or mixed nerve block can cause denaturation of the nerve, thus reducing focal spasticity. Advantages include low cost and immediate effects. Complications include sensory loss, painful dysesthesias with mixed nerves, and vascular complication. Chemodenervation with botulinum toxin types A and B is used “off label” for the treatment of focal spasticity. When used in combination with physical modalities such as splinting or serial casting, improved range of motion and Ashworth scores can be achieved. There are common patterns of upper motor neuron system dysfunction in patients with TBI that can be identified and effectively treated with botulinum toxin.
Baclofen can also be delivered intrathecally, directly to the lumbar subarachnoid space via an intrathecal pump. This delivery system circumvents the BBB and the peripheral breakdown of the orally administered medication. Although sedation often limits the oral dosing of baclofen, sedation occurs less frequently with intrathecal treatment, and the dose limit is much higher. The surgical complications of intrathecal pump placement include infection, catheter dislodgement, and CSF leak or seroma. The abrupt withdrawal of baclofen, which may occur with catheter complications, can result in rhabdomyolysis, multiple organ failure, and death. However, when studied in patients with TBI, after 1 year of treatment, Ashworth scores were improved and maintained over the year of treatment. Animal data suggest the effectiveness of early use of intrathecal baclofen (initiated 1 week after experimental injury) in decreasing spasticity and anxiety-like behavior without negative effects on cognition. This technique is being transitioned to human models with safety and efficacy observed in implants done in the first 6 months after injury.
Normal Pressure Hydrocephalus.
Normal pressure hydrocephalus is one of the most common treatable neurosurgical complications after severe TBI, with an estimated incidence of 45% in those with severe injury. Three-quarters of hydrocephalus occur during the inpatient rehabilitation process. It is more frequent with increased severity of injury and with longer rehabilitation length of stays. Posttraumatic hydrocephalus is largely of the communicating variety; thus, there is still free flow of cerebrospinal fluid within the ventricular system. Absorption of CSF into the arachnoid granulations is limited by blood products, protein, or fibrosis, leading to dilatation of the ventricular system. Clinical presentation of acute hydrocephalus, early after injury, includes headache, nausea, vomiting, and lethargy. The symptoms of delayed hydrocephalus or normal pressure hydrocephalus are more subtle. The clinical triad of dementia, gait ataxia, and urinary incontinence is described, with the gait disorder being most amenable to shunting of CSF. However, in those patients with TBI who have neurologic impairment from their injury, the diagnosis should be considered in anyone who worsens or fails to progress adequately. A CT scan of the head without contrast to evaluate ventricular size is necessary. However, as many as 72% of patients with severe TBI have been reported to have ventriculomegaly. In patients with cerebral atrophy leading to ex-vacuo dilatation of the ventricle, sulcal prominence is often observed, making it less likely they will be responsive to shunting. Further evaluation in questionable cases of hydrocephalus may include large-volume CSF drainage via lumbar puncture, including postprocedure neurologic evaluation, as well as MRI cine flow studies in which spinal fluid flow through the third ventricle is observed.
Endocrine Dysfunction Associated with Traumatic Brain Injury.
Although reported frequencies and numbers vary by study, neuroendocrine disorders affect a significant portion of the population with TBI, regardless of injury severity. Up to 100% of patients with acute TBI have some type of acute anterior pituitary dysfunction, and up to 37% of long-term survivors continue to do so. Data are sparse, but one report suggests acute posterior pituitary dysfunction rates as high as 21%, with 7% evolving into permanent dysfunction. Much of the pathology is attributed to primary and/or secondary injury to the hypothalamus and pituitary gland, structures that govern most neuroendocrine function. The hypophyseal portal vascular system that supplies the hypothalamic-pituitary region is vulnerable to traumatic injury, and frequent pituitary hemorrhage and necrosis have been noted in autopsy studies in patients who die acutely from their injuries.
Both oxytocin and vasopressin are synthesized in the hypothalamus and secreted by the posterior portion of the pituitary. In women, oxytocin functions to facilitate uterine contractions during labor and milk excretion during lactation. Oxytocin receptors are present in several regions in the brain, although their importance is not fully understood. Vasopressin, also called antidiuretic hormone (ADH), regulates water retention. Vasopressin release is diurnal and has many actions on the brain, including aggression and thermal regulation. Although less common than anterior pituitary dysfunction, posterior pituitary-mediated neuroendocrine dysfunction does occur after TBI. Diabetes insipidus is associated with excessive thirst, excretion of large amounts of dilute urine that is refractory to fluid restriction, and elevated plasma sodium. Neurogenic diabetes insipidus is caused by ADH deficiency, and vasopressin hormone replacement is effective therapy. In contrast, the syndrome of inappropriate antidiuretic hormone (SIADH) is characterized by excessive ADH secretion accompanied by excess natriuresis and low plasma sodium. Fluid restriction and ADH inhibitors are a part of the therapeutic approach to this disorder, with one study suggesting that hydrocortisone could be effective in reducing excessive natriuresis.
Several hormones affecting reproduction and metabolism are located in the anterior pituitary and include follicular stimulating hormone, leuteinizing hormone, ACTH, thyroid-stimulating hormone, prolactin, and growth hormone (GH). Early after injury, several aberrations in serum gonadal hormone profiles occur with severe TBI, and are likely associated with an enhanced stress surge in response to the injury. Testosterone is elevated in women, and progesterone is elevated in men after severe TBI. Both testosterone and progesterone levels rapidly decrease in men and women after severe TBI, refuting the long-held hypothesis generated from experimental models that women are afforded sex hormone-dependent neuroprotection. Hypogonadism is largely secondary to pituitary dysfunction, and menstrual cycle function may be impaired for women up to several months after severe TBI and can have a negative impact on outcome.
Hyperprolactinemia can affect over 50% of the population with TBI. Trauma can lead to acute depression of the thyroid axis. Chronic hypothyroidism affects between 1% and 22% of the population with TBI and can contribute to cognitive decline. Therefore, thyroid function should be screened routinely, particularly if recovery is slow. GH deficiency in children is associated with short stature. In adults, GH deficiency decreases muscle mass, energy levels, and cognitive performance. In TBI, one report suggests that chronic GH deficiency occurs in about 16% to 18% of the population and is correlated with lower quality of life (QOL) and increased rates of depression. Other studies suggest impaired aerobic capacity with GH deficiency after TBI. However, GH replacement can have a beneficial impact on recovery and QOL.
Posttraumatic Headache.
Posttraumatic headache (PTH) is the most common secondary headache disorder, corresponding to approximately 4% of all symptomatic headaches. PTH is a cardinal feature of the postconcussive syndrome. It is unclear how frequently PTH represents an exacerbation of an already existent primary headache syndrome versus new onset of secondary headache. Headaches are often transient and abate in parallel with other postconcussive symptoms, but for some, headaches can linger as persistent. Headache types in the TBI population are diverse, and include tension type and migraine/probable migraine type. Many of those affected self-treat (with variable success) with acetaminophen and/or nonsteroidal antiinflammatory drugs (NSAIDs). In particular, those with migraine/probable migraine may need to seek medical care and use more traditional abortives (e.g., triptans, long-acting NSAIDs) and/or preventives. As many classes of preventative drugs (e.g., tricyclics, β blockers, calcium channel blockers, anticonvulsants) have significant side effect profiles, practitioners will need to tailor therapy to minimize adverse effects on other recovery domains, and treatment selection may differ depending on factors such as time from injury, age, and premorbid headache history, as well as concurrent cervicalgia, cognitive/behavioral dysfunction, or mood disorders, among others.
Neurodegenerative Disorders and Chronic Traumatic Encephalopathy.
Relatively little is known about the long-term effects of TBI in relation to neurodegenerative disease; however, the concept of long-term neurodegenerative consequences of repetitive TBI has garnered much attention recently with increased community awareness about possible adverse effects associated with sports and military concussion. At a neurobiologic level, long-term disease thought to contribute to neurodegenerative disorders includes chronic inflammation, excitotoxicity, gliosis demyelination, neurotrophin dysfunction, amyloid plaques, and tauopathy, among others, with evidence available in animal models of TBI to support these considerations. Further, clinical studies are suggestive of these mechanisms with recent clinical biomarker studies showing evidence of long-term inflammation, autoimmune dysfunction, neurotrophin deficits, and neurodegenerative markers. These findings lend additional support to the possibility that genetic variations within genes that are associated with other disorders (e.g., APOE4 and Alzheimer dementia) have an important role in biosusceptibility to late neurodegenerative complications after TBI. In addition to late development of neurodegeneration and related dysfunction, these pathomechanisms may influence susceptibility to complicating conditions discussed earlier such as mood disorder, epilepsy, and neuroendocrine dysfunction. Although much research is needed to definitively describe the epidemiology, pathology, biosusceptibility, and treatments required to address these disorders, long-term rehabilitative care programs will likely emerge to include multimodal strategies to deal with TBI as a chronic disease requiring continued management.
Functional Evaluation and Treatment Concepts in Rehabilitation
In addition to the medical issues described previously, some functional difficulties commonly assessed and treated after TBI by the rehabilitation team are highlighted below.
Vestibular Dysfunction.
Patients with both severe and mild TBI often present with complaints of dizziness and imbalance. The incidence of such symptoms is reported to be between 30% and 60% in various TBI populations. The incidence could be as high as 100% when the patient has sustained a temporal bone fracture.
There are many etiologies for these symptoms. Injury to the peripheral nervous system may result in vestibular symptoms with or without hearing loss. Benign paroxysmal positional vertigo is the most common cause for complaints of dizziness and imbalance. It is clinically characterized by brief episodes of vertigo provoked by movement of the head. Although this can resolve with time, canalith repositioning procedures can shorten the duration of symptoms. Another peripheral vestibular injury includes labyrinthine concussion, or the sudden onset of hearing loss and vertigo after TBI in the absence of temporal bone fracture. These patients are good candidates for vestibular and balance rehabilitation. Temporal bone fractures can lead to disruption of the bony labyrinth or internal auditory canal. Trauma to the external canal clinically manifests with bloody otorrhea and severe pain and must be differentiated from a traumatic tympanic membrane perforation.
Central causes of dizziness can include direct trauma to the brainstem and/or cerebellum. These lesions are often associated with typical ocular motor abnormalities on neurologic examination. They include various forms of nystagmus at rest, eye movement abnormalities resulting in diplopia, and abnormalities of pupillary response. Rehabilitation techniques to promote alternative strategies for gaze stability can be successful to induce long-term adaptation in the central nervous system. The focus of such rehabilitation programs is to decrease symptoms and allow patients to be more active. In patients with postural instability, exercises to promote static and dynamic postural stability are conducted by manipulating visual, vestibular, and somatosensory cues in the environment.
Visual/Perceptual Dysfunction.
TBI can lead to many symptoms related to vision such as diplopia, photophobia, difficulties with tracking and fixation, and visuoperceptual complaints. Injury may occur to the optic nerve, visual cortex, visual processing centers, or oculomotor nerves. Each of these areas of injury has variable recovery patterns and rehabilitation interventions.
Common examination findings observed with physiatric examination include oculomotor abnormalities, visual field deficits, and deficiencies in visual acuity. The most common cranial nerve palsy after TBI is third nerve palsy. On examination, one sees exotropia, ptosis, and mydriasis on the affected side. Fourth cranial nerve palsy results in difficulty with convergence or near vision. Clinically, a head tilt may be noted. Sixth cranial nerve palsy is associated with difficulty of abduction of the affected eye, resulting in diplopia on lateral vision to that side. Visual acuity can be evaluated with the Snellen Acuity Test, testing both monocular and binocular vision. A bedside visual field examination should be done, and functional testing by an occupational therapist is often helpful if the patient can participate fully in this evaluation.
A neuro-ophthalmologic evaluation can assist therapists in evaluating the impact of vision on ADLs and perceptual motor function. In patients referred for evaluation because of visual complaints after TBI, 85% have visual acuity of 20/20 and 33% have cranial nerve palsy on examination. Vision therapy is the clinical treatment of visual disorders with the use of nonsurgical techniques. Over a series of treatment sessions under the supervision of an optometrist or occupational therapist, individually planned activities based on physical examination are repeated. Techniques include prisms, computer-based treatments, biofeedback, and stereoscopic devices.
Exercise and Traumatic Brain Injury.
In addition to the known therapeutic effects on physical condition and function, a significant amount of literature demonstrates that physical exercise and conditioning can have beneficial effects on neuroplasticity and cognition as well as slowing neurodegenerative processes. Increasing evidence suggests that voluntary exercise is beneficial in TBI recovery. In experimental TBI, voluntary exercise with a running wheel has been linked with increased neurotrophin production as well as improved cognition when compared with a sedentary control group. Multiple experimental studies, however, suggest that exercising too early after injury can be detrimental to recovery and have translational implications to clinical concussion management issues such as return to sporting activities after injury.
For those with concussion, physical exertion or training too early after injury can worsen cognition and associated symptoms. As such, exercise programs should be reintroduced in a graded fashion such that the patient remains symptom free during training. This approach is supported by experimental TBI work in which voluntary exercise early after TBI resulted in worse performance on tasks of learning acquisition and memory and a reduction in plasticity-related proteins compared with a nonexercising injured control group that underwent a sham treatment. In contrast, a delayed voluntary exercise paradigm increased neurotrophin levels and improved performance on cognitive tasks, which recent studies suggest corresponds with resolution of the acute physiologic stress response that accompanies TBI.
In the experimental literature, environmental stimulation, along with opportunity for social interaction and exercise, provides a loose context for a rehabilitation-relevant experience. Several experimental TBI studies demonstrate the relative benefits of this type of “enriched environment” on cognitive performance after TBI when compared with an impoverished environment with little environmental stimulation or social interaction. Interestingly, environmental enrichment can enhance the effectiveness of pharmacologic interventions in improving cognition. However, the beneficial effects of short-term environmental enrichment appear to be more effective in males than in females.
In patients with more severe injuries, behavioral factors, including agitation, impulsivity, and aggression can lead to unique challenges in implementing an exercise program. Cognitive and behavioral rehabilitation strategies outlined in this chapter can be helpful to create an environment most conducive to rehabilitation participation. For those with more severe injuries, physical impairments related to hemiplegia, movement disorders, and spasticity may also limit the degree of exercise and conditioning incorporated into individual rehabilitation programs. Other factors, such as balance and postural control as well as visual deficits, may affect abilities and performance with exercise training or programs.
Locomotion assistance systems that provide body weight support and robotic gait orthoses can be implemented for exercise in some patients with these types of deficits to provide additional support and feedback during locomotion training. Body weight–supported treadmill training has been more widely studied in stroke, and some studies suggest that treadmill training with body weight support may be more beneficial than treadmill training alone in this population. One study suggests that body weight-supported treadmill training does not necessarily improve gait mechanics in a chronic TBI population with multiple gait abnormalities. However, other studies suggest that body weight-supported treadmill training can improve cardiorespiratory status in independent walkers with severe TBI.
Cognition After Traumatic Brain Injury
Cognitive Deficits After Traumatic Brain Injury.
Cognitive deficits are among the most debilitating and complex aspects of TBI to manage and treat. Cognitive problems after TBI can span a number of neuropsychological domains, including arousal, attention, memory, and executive control. The neurobiologic bases of both physiologic cognitive function and TBI-induced dysfunction are not completely understood, and neuropharmacologic approaches for treating TBI-mediated deficits have largely drawn upon literature from related disorders that present with cognitive dysfunction. Among these are attention deficit hyperactivity disorder (ADHD), Parkinson disease, narcolepsy, and Alzheimer dementia. Pathomechanisms involved in TBI-related cognitive deficits are unique and not wholly similar to these other diseases.
Arousal is one of the most basic cognitive functions required to engage in other higher levels of cognition. Arousal is governed primarily by the reticular-thalamic, thalamocortical, and reticular-cortical networks. Multiple neurotransmitter systems, particularly monoamines, affect arousal. These monoaminergic nuclei originate in the brainstem at the reticular formation, and each has multiple forebrain terminal projections. Although other neurotransmitters influence arousal, NE is central to this function, which affects other cognitive domains, such as attention and memory.
Attention can refer to a wide variety of different cognitive processes that are both voluntary and involuntary. In addition, attention is a widely distributed cognitive function that involves both cortical and subcortical pathways, including striatal and thalamic inputs and reticular activation. It is generally accepted that DA plays a significant role in attention. Attention can be operationalized to include multiple processes. Sensory gating is a preattentive process that filters incoming stimuli to limit information into attention-processing networks. Selective attention involves the ability to direct cognitive resources to a salient stimulus, although sustained attention allows for maintenance of attention processing on a specific stimulus. Like arousal, there is overlap of many of these attention processes with other cognitive functions such as memory and executive functioning. Further, attention deficits can contribute to dysfunction in other cognitive domains.
Relative to arousal and attention, the anatomy involved with memory is better characterized, with specific memory-processing events (e.g., retrieval, consolidation) ascribed to specific brain regions. For example, damage to the hippocampus has historically been associated with reproducible deficits in spatial and temporal memory processing. Memory involves both encoding and retrieval processes, each of which may involve different structures. Explicit learning and memory are considered to be hippocampal-dependent processes of which declarative memory, or memory for facts and events that can be consciously discussed, is a major type. Declarative memory requires the hippocampus for memory consolidation and uses the frontal cortex for memory retrieval. Declarative memory retrieval is also considered associative in nature in that any area of the brain involved in encoding the information can be tapped to help initiate retrieval. In contrast, implicit learning and memory are a hippocampal-independent process that involves subconsciously learning how to do something, with procedural memory being a common type of implicit learning. Procedural learning and memory have a relatively inflexible retrieval process in that they are limited specifically to the processes involved in learning the task. However, strategy formulation, another relevant form of implicit learning, has shown relatively good carryover effects to a range of tasks. Implicit learning and memory are relevant for many other types of cognitive functions and both experimental and clinical data show that, compared with explicit memory, implicit learning and memory networks are relatively intact after TBI. As such, implicit learning and memory network capacity is commonly leveraged in cognitive training programs to produce guided responses that facilitate functional improvement in other domains such as ADLs. Because of the central role that the hippocampus plays in memory, cholinergic systems projecting to the hippocampus are implicated in memory dysfunction. The prefrontal cortex (PFC) and corticostriatal DA signaling system, in addition to the hippocampus, are also important for memory formation.
Executive function (EF) encompasses numerous functions, but it is generally defined as the capacity to organize, plan, execute, and change cognitive functions. Executive functioning is needed with abstraction, problem solving, self-direction, systematic memory searching and retrieval, and moving from one set of information to another. It is also used when maintaining information and generating goals and concepts. Working memory (WM) commonly refers to those cognitive processes that provide the capacity to both maintain and manipulate a limited amount of information over a brief period of time. WM is closely related to EF in that both processes involve the PFC. DA projections to the PFC along with PFC striatal networks are required for normal EF.
Clinical Neuropharmacology for Traumatic Brain Injury–Mediated Cognitive Deficits.
In contrast to experimental TBI models that have characterized specific alterations in neurotransmitter systems, evidence that neurotransmitter systems, including dopaminergic systems, are altered in humans following TBI is based largely on reports that DA agonists can be beneficial in attenuating cognitive deficits. Although amphetamine has been used clinically as both a DA and an NE agent, MPH is more widely accepted as an effective neurostimulant to improve cognition. According to current evidence-based guidelines, MPH is recommended as a neurostimulant to improve cognition, particularly attention. Much of the evidence for this is derived from small but well-controlled clinical trials showing improvements in cognitive processing speed and caregiver ratings of attention.
The evidence-based guidelines support the use of amantadine to enhance general cognition and attention in patients with moderate and severe TBI. There are case reports demonstrating improved scores on ADL scales in patients treated with amantadine. Case reports also indicate general improvements in global functioning and processing speed. PET imaging studies show amantadine-mediated increases in cortical glucose metabolism in patients with TBI. Notably, a recent large clinical trial demonstrated the benefits of amantadine in individuals with disordered consciousness who were in the subacute to early chronic phases of recovery. In patients with DAI, amantadine appears to be effective in improving cognition independent of the timing of administration. Although bromocriptine is less well studied clinically than MPH and amantadine, small case series show some evidence that low-dose bromocriptine may improve EF. Recent work suggests though that daily bromocriptine is not effective for treating attention deficits.
Some work has been undertaken regarding noradrenergic agonists in TBI recovery. The tricyclic antidepressant and NET inhibitor desipramine has been used for years for depression, and increased arousal and initiation in a TBI case series and improved post-TBI depression. Clinical reports also suggest some potential efficacy with the selective NET inhibitor atomoxetine.
Previous large randomized controlled trials with CDP-choline have shown improvements in memory and global function in patients with TBI across the injury severity spectrum. Notably, though, a recently completed definitive trial of CDP-choline did not result in overall improvement in a complicated mild to severe TBI population. Alternatively, both case series and small randomized controlled trials suggest that the cholinesterase inhibitors can improve memory and attention as well as general cognition. Interestingly, daily treatment with the cholinesterase inhibitor donepezil results in cortical increases in metabolism, and magnitude of metabolic change is associated with degree of clinical treatment response. Clinical trials are needed to further define donepezil treatment effects on cognition.
Purpose of Neuropsychological Assessment.
The purpose of neuropsychological assessment varies across the course of recovery from TBI. The initiation of assessment may occur during the initial stages of emergence from coma. Later, neuropsychologists often are responsible for evaluating a patient’s progress through PTA. Neuropsychological testing is useful in evaluating the domains of cognitive functioning described above. However, the ability to complete formalized testing of any sort is dependent on the ability of the patient to tolerate or respond to the tools used. As a result, extensive formal neuropsychological testing is generally not completed while an individual is in PTA; instead, brief tools and batteries are introduced as an individual is able to participate. There are also times when formalized testing of certain cognitive areas may not be possible for a period of time secondary to other brain injury-related issues, such as aphasia, severe limitations in motor or sensory abilities, medical issues, or behavioral issues that may impede participation. Studies suggest that it is feasible to administer brief testing during acute inpatient rehabilitation, and these data suggest some prognostic value when completing certain types of neuropsychological testing within the early weeks of the recovery process. Full neuropsychological test batteries are often not administered for several months after moderate to severe TBI. These detailed outpatient batteries may be used to answer questions related to return to school, work, driving, or other activities and to assess ongoing recovery.
Practical Interventions for Cognitive Impairment After Traumatic Brain Injury.
When interacting with patients during the inpatient rehabilitation phase of recovery, it is helpful to have an understanding of the “typical” presentation of cognitive impairment associated with TBI, so that information for promoting effective rehabilitation interactions between staff and patients may be presented. Patients who are in inpatient rehabilitation following moderate to severe TBI are typically still in PTA. During this period of confusion, patients may be disoriented to person, place, time, and circumstance. They generally do not remember what has happened to them. If it is explained to them that they have been injured, they are often not able to process and retain the information. As a result, it may be very difficult for them to make sense of their surroundings and circumstances. They may pull at tubes, sutures, braces, helmets, and so on, because they find them uncomfortable and are not able to process the fact that such items are important and necessary for their care. They may refuse therapies because they feel tired and in pain and are not able to appreciate the benefits of participation. They will likely have difficulties remembering multistep instructions in therapy, what was presented to them during a previous session, or perhaps even moments before. Patients who have sustained TBI likely also have additional cognitive impairments caused by injury. Examples include language deficits, sensory impairments, difficulty initiating or stopping behaviors, or difficulties interpreting visual or spatial information. Individuals may wander or engage in inappropriate, unsafe, and repetitive behaviors. When the patient’s cognitive status is taken into consideration, these behaviors become more understandable; however, staff and families may find these behaviors difficult to manage. Education of staff and families regarding cognition is often key to understanding a patient’s needs.
As patients are often not able to make sense of their environment during this period of acute confusion, an overarching goal of rehabilitation should be to provide a structured program to assist patients through this period of recovery. As recovery progresses, they will learn what to expect from their daily schedule more easily if structure has been established. In addition, although orientation is tested frequently to assess progress through PTA, therapists, nursing staff, and family members should also gently provide orientation information in conversation with the patient throughout the day. It is often helpful to have a daily calendar in the patient’s room, as well as a clock so that the patient may have a reference point. Staff members should reintroduce themselves each time they interact with the patient, even if they have worked with the patient just a short time before. As the patient recovers and is independently able to identify staff members and to call them by name, this practice may be discontinued. As many patients are much more sensitive to sensory stimulation or may have field cuts or visual neglect following TBI, staff members are less likely to startle a patient by approaching a patient from the front, gaining the patient’s attention, and explaining what they will be doing before touching a patient, moving a patient, or engaging in other interactions. Nursing, medical procedures, and therapy tasks should be explained in a step-by-step manner, even if the patient does not appear able to respond or show overt awareness.
In general, persons with TBI can recall information about their family and personal history, even though their ability to encode and recall more recent events is impaired. As a result, it is often comforting, for example, to have pictures of family members, pets, and favorite places in the patient’s hospital room. It is helpful for therapists to ask family members for detailed information about the patient’s history, including education, work history, hobbies, and interests, so that components of these aspects of the patient’s life can be incorporated in therapies when possible and therapy can be more personalized.
Patients who are not aware of their deficits or are impulsive may be at risk for engaging in unsafe behaviors. During the initial stages of recovery, the best approach is to guard them through therapeutic interactions. One-to-one sitters or restraints may be required for safety. As patients recover, however, it is often helpful to teach them strategies to reduce impulsivity (i.e., teach them step-by-step plans until overlearned or have them count/pause to review steps before moving). For lack of awareness, it is sometimes necessary to compare patient performance and progress with an objective standard. Logical verbal discussion is often not effective when a lack of awareness is caused by cognitive impairment related to injury. Group therapy with peers who are able to challenge the person may sometimes be effective at later stages of recovery.
All of the behavioral and cognitive issues discussed in this section tend to improve over the recovery period. That said, some of the issues observed in the inpatient setting may continue to be present as the patient transitions to home or other settings. In fact, according to one study, approximately 40% of individuals reported ongoing unmet needs at 1 year, with the most frequently cited issues including memory and problem solving, managing stress and emotional upset, and temper control. However, many of the routines and techniques learned in therapy may be applied successfully by families in the home setting. Staff should be certain to educate families regarding the purpose and use of these techniques. Continuing to set up routines and structure to assist individuals and to increase the likelihood of success may play a significant role in decreasing depression, irritability, and difficulties with explosive anger. Treatment with medication, combined with therapy to manage adjustment to disability, anxiety, and depression, as well as to teach anger management skills is often recommended. Box 43-3 reviews helpful behavioral and cognitive strategies for common issues following TBI.
Early in recovery, individuals with brain injury are often confused and have difficulty remembering new information. Staff should:
- •
Introduce and reintroduce themselves, even if they have done it earlier in the day or week.
- •
Provide consistency and structure (ideally a consistent schedule with consistent staff).
- •
Provide reorientation frequently.
- •
Use simple, short sentences and don’t give too much information at once.
- •
Cue the patient as needed, providing some direction and guidance.
- •
Provide extra time and repetition as needed.
Individuals with brain injury may become easily overstimulated. Overstimulation will likely have a negative impact on cognition and may increase the likelihood of irritable or agitated behaviors. It can be helpful to:
- •
Limit distractions and noise around the patient as needed (move treatment to a quiet location, dim lights, reduce noises such as television and radio, limit the number of people talking and interacting with the person at one time).
- •
Break down difficult tasks into smaller steps and give simple, step-by-step directions.
- •
Be flexible. Redirect patients when they become frustrated with an activity, allow rest breaks, and alternative activities as needed.
- •
Remain calm in interactions and speak in a clear, concise manner using quiet volume and calming tones.
- •
Keep issues such as pain and fatigue in mind, and treat as needed.
Individuals with brain injury are often “impulsive.” This means they may act without thinking. For example, patients may move quickly and unsafely and try to do things that they are not capable of doing independently. To promote safety:
- •
Be sure to have the patient’s attention before you speak.
- •
Speak slowly, clearly, and softly using the patient’s name frequently to keep their attention.
- •
Use demonstration in addition to verbal instructions when possible.
- •
Promote team and family communication so that consistent strategies are being used.
Additional tips for working with individuals with brain injury:
- •
Always approach patients slowly and gently and approach them from the front, not from behind.
- •
Do not take behaviors personally.
- •
During periods of significant agitation, create situations where the patient can be successful and avoid situations in which they may fail or will need to be corrected.
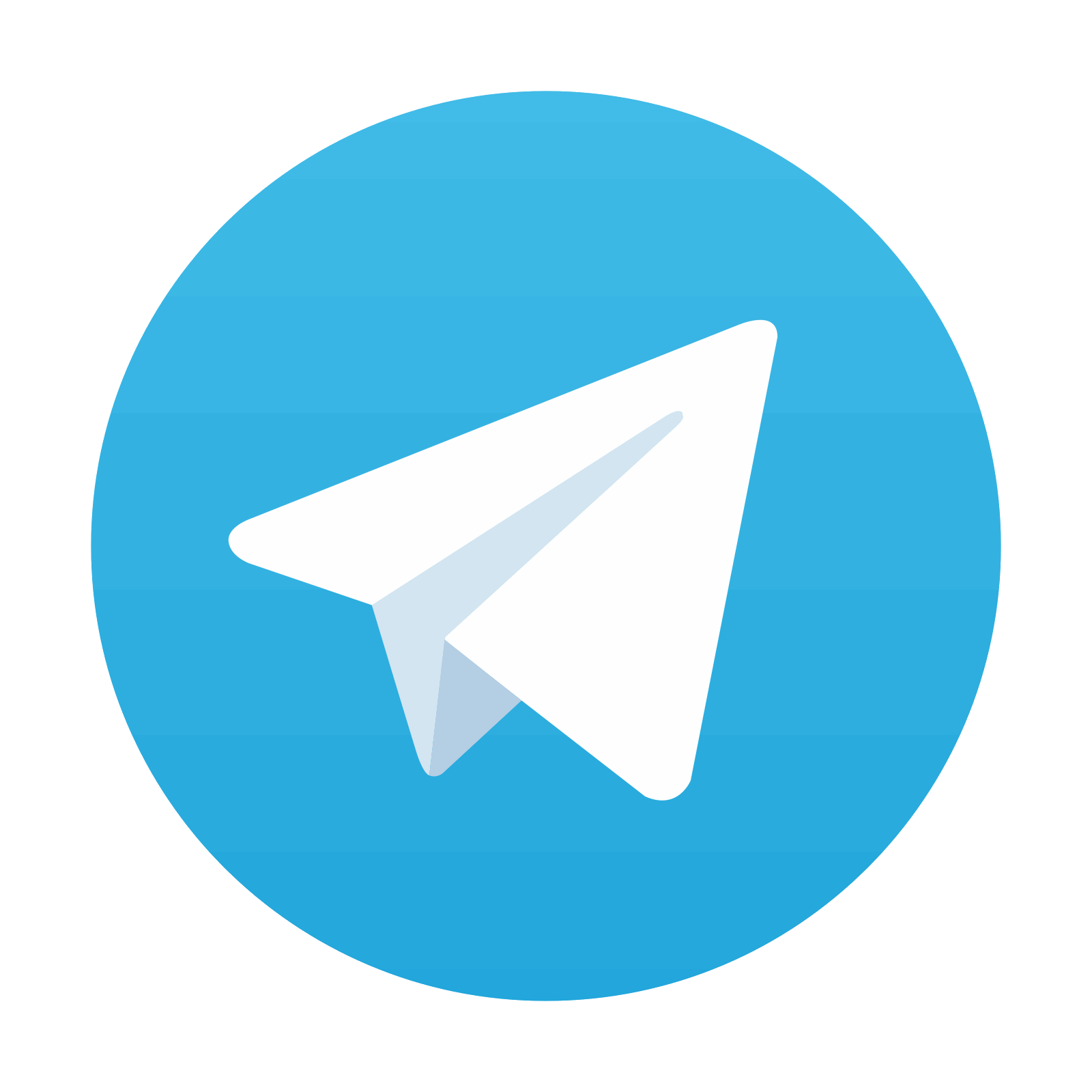
Stay updated, free articles. Join our Telegram channel

Full access? Get Clinical Tree
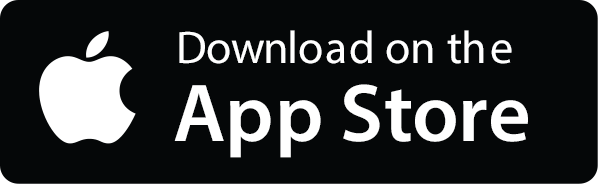
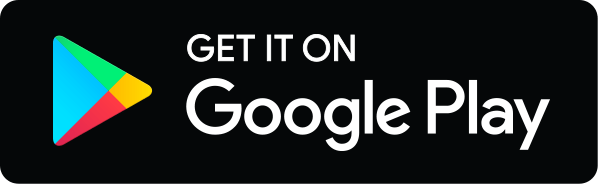
