Abstract
Locomotor behavior is controlled by specific neural circuits called central pattern generators primarily located at the lumbosacral spinal cord. These locomotor-related neuronal circuits have a high level of automaticity; that is, they can produce a “stepping” movement pattern also seen on electromyography (EMG) in the absence of supraspinal and/or peripheral afferent inputs. These circuits can be modulated by epidural spinal-cord stimulation and/or pharmacological intervention. Such interventions have been used to neuromodulate the neuronal circuits in patients with motor-complete spinal-cord injury (SCI) to facilitate postural and locomotor adjustments and to regain voluntary motor control. Here, we describe a novel non-invasive stimulation strategy of painless transcutaneous electrical enabling motor control (pcEmc) to neuromodulate the physiological state of the spinal cord. The technique can facilitate a stepping performance in non-injured subjects with legs placed in a gravity-neutral position. The stepping movements were induced more effectively with multi-site than single-site spinal-cord stimulation. From these results, a multielectrode surface array technology was developed. Our preliminary data indicate that use of the multielectrode surface array can fine-tune the control of the locomotor behavior. As well, the pcEmc strategy combined with exoskeleton technology is effective for improving motor function in paralyzed patients with SCI. The potential impact of using pcEmc to neuromodulate the spinal circuitry has significant implications for furthering our understanding of the mechanisms controlling locomotion and for rehabilitating sensorimotor function even after severe SCI.
1
Introduction
Epidural spinal-cord stimulation is an effective tool for regulating locomotor behavior and can allow for regaining voluntary control of movements by paralyzed patients . Here we describe a novel non-invasive technique — transcutaneous electrical spinal-cord stimulation — used to neuromodulate the physiological state of the non-injured and injured spinal cord.
1.1
The concept of the automaticity in understanding the neural control of movement in the non-injured and injured spinal cord
Spinal locomotor neuronal circuitries, called central pattern generators, can induce stepping EMG patterns without supraspinal input and/or peripheral afferent input. This phenomenon came to be called fictive locomotion (i.e., locomotion in the absence of movement). The ability to produce actual locomotion in the absence of brain input can be attributed to a combination of intrinsic properties of the circuitry generating fictive locomotion with the ability to process complex proprioceptive and cutaneous patterns. These features enable the lumbar circuitry to adapt the motor patterns to accommodate different speeds and levels of weight-bearing and sustain successful stepping in a continuously changing environment. To utilize this potential, the spinal circuitry seems to persist in a highly dynamic state, which reflects the immediate and chronic patterns of sensory input being processed by the spinal networks.
The idea that networks of neurons within biological systems can generate cyclic motor output is decades old. Key experiments demonstrating the significance of the automaticity of motor control in the mammalian spinal cord were performed by Brown in 1911 and Shik et al. in the 1960s and 1970s. These experiments built a strong conceptual basis for the automaticity of the neural control of locomotion and posture. Shik and Orlovsky proposed that one level of control provides nonspecific tonic input that determines the intensity of locomotion (speed and grade), while the other is responsible for fine adjustments for the control of the limbs, including for maintaining equilibrium. This fine control system normally interacts with sources of sensory information, such as proprioceptive and visual inputs, to execute fine adjustments in the locomotor pattern. After the appearance of these key findings , many studies have attempted to define the mechanisms underlying the phenomenon of automaticity of movement control.
We now know that the spinal circuitry can learn a task that is taught (practiced) and that it can forget the task if it is not practiced . These and similar observations of the plasticity of the spinal locomotor circuitry provide the fundamental basis for re-examining current concepts and considering new ones that might lead to a greater potential for recovery from spinal-cord injury (SCI).
In the following sections, we describe the development of a novel technique to take advantage of the basic concepts of automaticity in maximizing motor functions in non-injured subjects but more importantly in patients who have lost motor function due to SCI.
1.2
Methodology of transcutaneous electrical spinal-cord stimulation
We introduce a novel non-invasive stimulation strategy of painless transcutaneous electrical enabling motor control (pcEmc) to neuromodulate the physiological state of the spinal cord. This method includes electrically activating the spinal circuitry via electrodes placed on the skin overlying the vertebrae of the lower thoracic and/or lumbosacral vertebrae. One of the innovative features is the use of a specific stimulation waveform that does not elicit pain even when used at energies required to transcutaneously reach the spinal networks. This waveform consists of 0.3- to 1.0-ms bursts with a carrier frequency of 10 kHz administered at 5 to 40 Hz . By modulating the spinal cord with this non-invasive device, we can safely use energies that were previously prohibitive due to pain. In our initial studies, pcEmc stimulation was delivered by a 2.5-cm round electrode (Lead-Lok, Sandpoint, USA) placed midline at the C5, T11, and/or L1 spinous processes as cathodes and two 5.0 × 10.2 cm 2 rectangular plates made of conductive plastic (Ambu, Ballerup, Germany) placed symmetrically on the skin over the iliac crests as anodes. Biphasic rectangular 0.5- to 1.0-ms pulses with a carrier frequency of 10 kHz and at an intensity ranging from 30 to 200 mA were used.
1.3
Effects of pcEmc on stepping movements
We demonstrated that pcEmc at 30 Hz applied to T11 results in step-like movements in non-injured subjects when their legs are placed in a gravity-neutral position. Such stimulation produced involuntary coordinated, cyclic, oscillatory, locomotor-like steps in 5 of 6 normal subjects when placed in a gravity-neutral position . An example of the kinematics of the hip, knee, and ankle and electromyography (EMG) activity from selected muscles as well as right–left coordinated movements during pcEmc in non-injured subjects is shown in Fig. 1 . Furthermore, we refined the technology of pcEmc to involve multi-segmental stimulation to further improve locomotor ability. Use of a three-channel stimulation device permitting independent modulation via 3 different spinal locations at the C5, T11, and L1 vertebrae in a normal subject induced robust oscillatory and coordinated stepping movements that reached maximal excursions and EMG burst amplitudes within 2 to 3 step cycles; these movements were much greater than with stimulation at T11 alone ( Fig. 1 ). Reciprocal, alternating patterns were more evident with multiple- than single-site stimulation. The synergistic and interactive effects of pcEmc suggest a multi-segmental convergence of descending and ascending and most likely propriospinal effects on the spinal neuronal circuitries associated with locomotor activity. These observations are consistent with the concept of differential modulation of the activation levels of combinations of motor networks projecting to specific combinations of interneurons that coordinate the levels of recruitment of different combinations of motor pools throughout a step cycle .
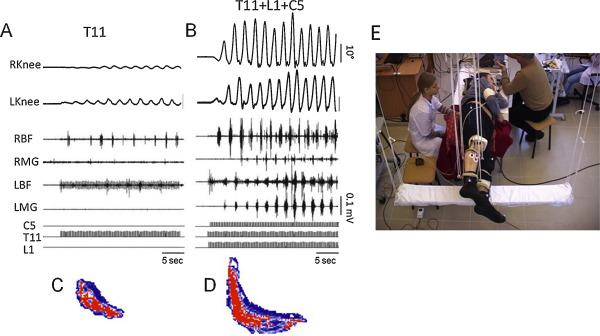
1.4
Multielectrode surface array — a new approach for controlling locomotion in humans
In mammals, the rhythmogenic capacity of the spinal networks may be distributed throughout the lumbosacral cord along a rostro-caudal gradient . Recently, we demonstrated the site-specific effect of spinal-cord stimulation in facilitating assisted stepping in a motor-complete subject with SCI. With an epidural 16-electrode array placed on dura between L1 and S1 spinal segments, the most effective assisted stepping occurred when the spinal cord was stimulated by rostral and caudal electrodes of the array . Also, transcutaneous multi-site spinal-cord stimulation modulated the spinal locomotor circuits and facilitated locomotion more effectively than did single-site stimulation (see above). We developed a 9 (3 × 3) multielectrode surface array allowing for independent stimulation of the spinal cord at multiple sites to control the locomotor behavior with different combinations of the stimulation paradigm ( Fig. 2 E) . Fig. 2 shows the effects of spinal-cord stimulation at one level (T11) with 3 electrodes (A,B,C) located at midline (B) and laterally (A and C) to the spinal cord versus stimulation at 2 levels (T11 + L1) with electrodes 1ABC + 3ABC in a non-injured subject with legs placed in a gravity-neutral position. The amplitude of knee displacement and EMG activity of leg muscles were significantly higher with multi-site stimulation at 2 levels than at one level ( Fig. 2 ). Our preliminary data reveal that use of the multielectrode surface array can fine-tune the control of the locomotor behavior.
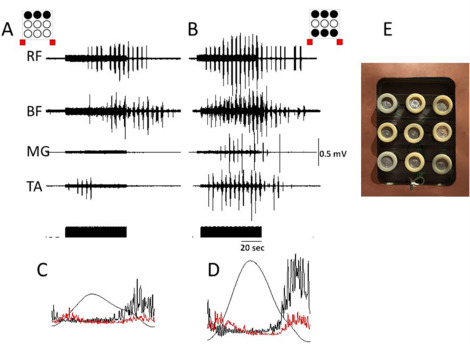
1.5
Spinally evoked motor potentials using transcutaneous electrical spinal-cord stimulation
The complex behavior and characteristics of the spinally evoked potentials with transcutaneous spinal-cord stimulation can be similar to the potentials evoked with epidural stimulation. We have characterized the relative selectivity of recruitment of different motor pools innervating leg muscles in non-injured subjects by using transcutaneous stimulation along the rostro-caudal axis of the lumbosacral enlargement ( Fig. 3 ), at sites similar to a previous study with epidurally implanted electrodes . Variation in many characteristics of the evoked potentials reflects a relative preferential activation of proximal and distal leg muscles based on the rostro-caudal position of the sensory-motor pathways and motor neuron pools. Transcutaneous electrical stimulation of rostral and caudal areas of the lumbosacral enlargement resulted in a selective topographical recruitment of proximal and distal leg muscles, based on their threshold intensity, maximal slope of the recruitment curves, and plateau point intensity and magnitude.
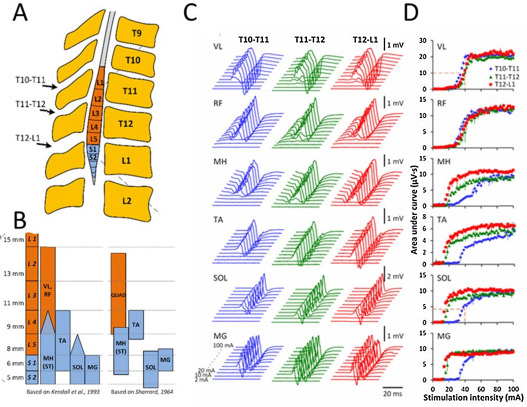
Fig. 3 presents the evoked potentials ( Fig. 3 C) and corresponding recruitment curves ( Fig. 3 D) obtained at different stimulation intensities at 3 spinal locations in one subject. Transcutaneous spinal-cord stimulation delivered even within a relatively narrow range between T10 and L1 vertebrae, which approximately correspond to L2-L4 or L4-S2 spinal segments, resulted in a different order of activation of the proximal and distal motor pools. During stimulation at T10-T11 with low stimulation intensities, the magnitude of response of vastus lateralis and rectus femoris muscles was higher than for medial gastrocnemius, soleus, and medial hamstrings muscles. However, with stimulation at T12-L1 and the same intensities, this relationship was reversed. The threshold of activation of medial gastrocnemius, soleus, tibialis anterior, and medial hamstrings muscles also depended on the location of stimulation, with thresholds increasing progressively with the more rostral site of the stimulation. In proximal and distal muscles, recruitment curve characteristics varied by stimulation location. Our data are generally consistent with previous reports and with the anatomy and myotomal maps of the spinal cord and lumbosacral roots ( Fig. 1 ).
These data demonstrate that transcutaneous electrical spinal-cord stimulation can be used to differentially activate motor pools and projecting dorsal roots based on their anatomical arrangements along the rostro-caudal axis of the lumbosacral enlargement. As compared with epidural stimulation, transcutaneous stimulation allows for the preferential activation of spinal structures at specific segments, even with the considerably wider configuration of the stimulating and reference electrodes and thus, a less focused current flow. Selective recruitment of proximal and distal motor pools can be titrated at low intensities. As with epidural spinal stimulation, with variation of the stimulation intensity and location, different neural structures may be activated. The maximal slope of the recruitment of particular muscles allows for characterizing the properties of afferents projecting to specific motor neuron pools as well as to the type and size of motor neurons. In light of the spatial arrangement of the motor pools and networks along the lumbosacral enlargement, electrical stimuli delivered to multiple sites over the lumbosacral enlargement may recruit different motor pools and also activate different combinations of networks. The resulting spatiotemporal activation patterns yield an electrophysiological map reflecting the responsiveness of multiple motor pools. This map may represent a neurophysiological marker for selecting effective stimulation sites for facilitating performance of a given motor task.
1.6
Possible neural structures activated during transcutaneous electrical spinal-cord stimulation
In a modelling study, transcutaneous stimulation could depolarize at least a subset of the same neural structures as recruited by implanted epidural electrodes . Data from experiments with epidural stimulation revealed that it could recruit both sensory and motor fibers as well as interneurons. Similar to these findings, our data indicate that transcutaneous spinal-cord stimulation can involve diverse elements along the spinal cord, based on the location and intensity and other neuromodulatory factors such as pulse shape and frequency ( Fig. 3 ). Low stimulation intensities may result in preferential recruitment of lower threshold afferent fibers, accompanied to some extent by involvement of motor axons. With increased intensity, more motor axons may become activated, thereby leading to decreased latency of the response and an occlusion effect of the afferent pathways. This notion concurs with prior results obtained from experiments with transcutaneous and epidural spinal-cord stimulation and in modelling studies . Considerable evidence from animal models and humans suggests that the modulation of multiple oligosynaptic pathways interconnecting multiple sensory types of afferents with interneurons also receive supraspinal input that generate a highly intricate coordination of multiple motor pools. We have clear evidence that the excitability of spinal interneuronal networks can be readily modulated (changing the networks’ physiological state) without directly activating any action potentials that actually generate a muscle contraction .
Increasing the stimulation intensity, in addition to the Ia afferents, the smaller-diameter afferents including group Ib, larger-diameter cutaneous afferents, group II muscle spindle afferents, and even more intraspinal connections and spinal interneurons as well as direct motor activation may all contribute to the response with progressively increased stimulation intensities ( Fig. 4 ). A wide range of facilitation and/or inhibition among constantly changing networks during an in vivo movement can be achieved by using multiple neuromodulatory techniques.
