- •
Flumazenil, when used for benzodiazepine overdose, may unmask seizures caused by a coingested substance or precipitate acute withdrawal in the patient who habitually uses benzodiazepines.
- •
β-Adrenergic antagonists, when used to lower blood pressure in a sympathomimetic overdose, may lead to unopposed α-receptor stimulation and should be avoided.
- •
Reserve physostigmine administration for severe, life-threatening manifestations of anticholinergic toxicity because it may lead to asystole or seizures. It is contraindicated for reversal of anticholinergic symptoms produced by tricyclic antidepressant ingestion.
- •
Pulse oximetry is unreliable for determining oxygen saturation with methemoglobinemia and may show falsely elevated or decreased values.
- •
Use the skin to distinguish between sympathomimetic (pale, cool, and diaphoretic) and anticholinergic (flushed, warm, and dry) toxidromes.
- •
Malignant hyperthermia, serotonin syndrome, neuroleptic malignant syndrome, sympathomimetic poisoning, and anticholinergic poisoning constitute the major differential diagnosis for xenobiotic-induced hyperthermia.
- •
An elevated osmolar gap may suggest ingestion of a toxic alcohol, but a normal result does not exclude it. Serum toxic alcohol levels are the gold standard for diagnosis and prognosis.
- •
Succinylcholine is relatively contraindicated in cholinesterase inhibitor toxicity because its duration of effect will be significantly prolonged.
- •
Total iron-binding capacity may be falsely elevated in patients with acute iron overdose and is not a reliable marker in iron toxicity.
- •
In the United States, the local Regional Poison Control Center may be reached at 800-222-1222.
The 36th annual report of the American Association of Poison Control Centers described more than 2 million human exposures to toxic substances that were reported to poison control centers in the United States in 2018. Approximately 50% involved children younger than 12 years, but this age group accounted for only about 2% of the reported fatalities. Although most exposures were managed without further medical assistance, of the 30% of patients who were referred and seen in a healthcare facility, one in six was admitted to a critical care unit. Calls from healthcare facilities to poison control centers are increasing and account for a growing proportion of cases handled by poison control center specialists.
In most cases of childhood poisoning, the agents involved are known or circumstantial evidence points to one or more specific toxins. When the toxin is unknown or the clinical presentation is inconsistent with the available exposure history, physical and analytic clues will help guide therapy. Toxidrome is a contraction of toxic syndrome, a constellation of signs associated with certain substances or groups of substances. Recognition of a toxidrome provides for targeted investigation and treatment. Toxidrome expression may not be complete in every case, or more than one toxidrome may be present. During the initial patient examination, clinicians should pay particular attention to vital signs, mental status, pupil size and reactivity, skin characteristics (color, temperature, moisture), and bowel sounds. Other important aspects to consider include muscle tone, respiratory effort, and mucous membrane characteristics.
As discussed in Chapter 125 , laboratory investigations may narrow the differential diagnosis and determine the need for additional examination or guide therapy. However, clinicians must review these diagnostic tests with caution. Many assays lack sensitivity and specificity to confirm specific substances. Thus, an inaccurate diagnosis or inappropriate therapy, including withholding a specific antidote, is a consequence of relying on these assays alone. In conjunction with clinical findings and exposure history, assays are chosen based on their probability of indicating or guiding therapy and prognosticating end-organ toxicity.
This chapter describes commonly accepted toxidromes as well as less common, yet relevant, toxic presentations and their treatments. More in-depth information can be found in a comprehensive textbook of medical toxicology.
Opioids
Both natural and synthetic opioids produce the classic triad of respiratory depression, coma, and miosis. Additional features include bradycardia, hypotension, and decreased gastrointestinal (GI) motility. Not all patients exposed to an opioid present with the classic toxidrome. For example, mydriatic or midpoint pupils are seen with specific opioids, although brain anoxia or coingestants should also be considered ( Box 126.1 ).
Meperidine
Pentazocine
Diphenoxylate/atropine
Propoxyphene
Simple hypercarbia may be detected before severe overt respiratory depression. Because decreased respiratory drive is a result of μ-receptor agonism but analgesic effect results from action at both μ- and κ-receptors, some opioids are designed to function as agonist-antagonists to provide spinal analgesia and simultaneously antagonize μ-receptors. , Other opioids, like buprenorphine, are designed to function as only partial agonists at μ-receptors, providing pain relief at higher doses with a ceiling effect on respiratory depression. In overdose, lost receptor selectivity may still result in decreased respiratory drive with this type of opioid medication. In overdose and in opioid-naïve pediatric patients, buprenorphine often causes overt respiratory depression. Thus, close monitoring of respiratory status is warranted.
Several potent opioids are extremely toxic in small doses in both the opioid-naïve and pediatric populations. Methadone liquids are concentrated—even as little as one teaspoon can lead to fatality. , Fentanyl, in dosage forms that allow for buccal absorption, may be attractive to children. Used fentanyl patches still contain high doses of drug and are extremely dangerous when placed on skin, in the mouth, or ingested. Fentanyl, and even more potent analogs such as carfentanil, are increasingly being found on the street and represent a more serious overdose risk than heroin because of their potency.
Central α 2 -adrenergic agonists—including clonidine, guanfacine, tizanidine, and other imidazoline derivatives (found in over-the-counter eyedrops or nasal sprays)—can precipitate an opioid-like toxidrome. In addition to bradycardia and hypotension, exposure to these agents in small doses may lead to central nervous system (CNS) and respiratory depression and miosis.
Naloxone is a μ-receptor antagonist that reverses the toxic effects of opioids. To prevent the need for artificial ventilation in life-threatening opioid-induced respiratory depression, start with a dose of 0.1 mg/kg. If no intravenous access is available, administration can proceed subcutaneously, intramuscularly, or via endotracheal tube (although the intramuscular route exhibits variable pharmacokinetics with a less predictable duration of action). Naloxone may precipitate acute withdrawal in opioid-dependent individuals, warranting a lower starting dose, titrated upward to effect and not exceeding 10 mg cumulatively. Because the duration of action of naloxone is shorter than that of some opioids, repeated doses or a continuous intravenous infusion may be necessary. The suggested infusion rate is two-thirds the initial reversal dose per hour, titrated to effect, with half the initial reversal dose given as a bolus. Respiratory and mental statuses are monitored for 24 hours following cessation of a prolonged naloxone infusion. Although naloxone has precipitated transient hypertension or pulmonary edema in rare cases, these risks do not preclude its use. Reports that naloxone has reversed clonidine overdose are anecdotal, and naloxone failure has also been described in this setting. Thus, the benefit of naloxone in the face of central α 2 -adrenergic agonists is unclear.
Sedative hypnotics
The class sedative hypnotics comprises medications that reduce CNS excitation, prescribed for their hypnotic and anxiolytic effects. Usually, sedative hypnotics enhance γ-aminobutyric acid (GABA) activity and its effect on chloride channels in the brain and spinal cord. Barbiturates act as agonists at GABA A receptors prolonging the flow of chloride through the channel, resulting in hyperpolarization. Benzodiazepines, on the other hand, work at the benzodiazepine location on the GABA A receptor to enhance GABA binding and increase the frequency of chloride channel opening—they will not work in the absence of GABA. , Newer nonbenzodiazepine sedative hypnotics (zolpidem, zopiclone, and eszopiclone) also bind to the benzodiazepine location on GABA A . However, because of their affinity for different isoforms of the receptor, they act quickly to induce hypnosis with little effect on anxiolysis. Initial reports indicated that these so-called z-drugs were a safe alternative to benzodiazepines, but more recent data depict a similar toxicity profile.
Barbiturate overdose, characterized by CNS and respiratory depression, may be associated with hypotonia, hypotension, and hypothermia, as well as bullous skin lesions. On the other hand, benzodiazepine and z-drug toxicity are often referred to as “coma with normal vitals,” in which patients exhibit CNS depression without overt respiratory depression or autonomic dysfunction. , In one published report, ataxia was the most common symptom. Thus, benzodiazepine ingestion should be considered in the differential diagnosis of ataxia with no additional findings. Unlike opioid toxicity, low benzodiazepine receptor density in the brainstem means that benzodiazepines and z-drugs pose a low risk for centrally mediated apnea. Although apnea has been reported with intravenous benzodiazepine administration, therapeutic and supratherapeutic oral doses cause airway resistance and increased work of breathing. In benzodiazepine overdose, patients are often described with sonorous respirations, and apnea is rare.
Significant respiratory depression with benzodiazepines usually only occurs from mixed-drug ingestions or in the presence of ethanol. Most pediatric exposures are unintentional single-substance ingestions posing less risk. Reversal of benzodiazepines with the benzodiazepine receptor antagonist flumazenil is usually not warranted and is, in fact, contraindicated in a polysubstance overdose, especially when cyclic antidepressants are suspected. Removing the antiepileptic effect of a benzodiazepine may unmask seizure activity and increase morbidity. Caution is also warranted in patients with an underlying seizure disorder in whom flumazenil may lower the seizure threshold and in patients habituated to benzodiazepines in whom reversal may precipitate life-threatening withdrawal. Flumazenil may reverse CNS depression; however, in dose-effectiveness studies, it did not significantly affect respiratory insufficiency.
Withdrawal from benzodiazepine and nonbenzodiazepine sedative hypnotics, similar to ethanol withdrawal, results from downregulation of inhibitory GABA receptors and increased excitatory N -methyl- d -aspartate (NMDA) binding sites. This imbalance is characterized by CNS stimulation, flushing, hypertension, tachycardia, hyperthermia, and seizures. Treatment is based on symptom reduction via replacement of benzodiazepines at the established dose and tapering as tolerated.
Sympathomimetic agents
Signs and symptoms of sympathomimetic excess result from both therapeutic and illicit agents that either mimic endogenous excitatory neurotransmitters or act on receptors to increase their release. Manifestations of the sympathomimetic toxidrome and some drugs that produce it are listed in Table 126.1 . Predominantly β-adrenergic agents cause peripheral vasodilation more likely resulting in tachycardia and hypotension than predominantly α-adrenergic agents (severe hypertension with reflex bradycardia). Hyperthermia, rhabdomyolysis, and myoglobinuria may result from increased metabolic activity; ischemic or hemorrhagic stroke and myocardial infarction have been documented. Following cocaine exposure, patients may present with chest pain from coronary vasospasm. However, myocardial infarction must be ruled out.
Sympathomimetic Toxidrome | Causative Agents |
---|---|
Agitation Seizures Mydriasis Tachycardia Hypertension Diaphoresis Pallor Cool skin Fever | Albuterol Amphetamines Caffeine Catecholamines Cocaine Ephedrine Ketamine Phencyclidine Phenylephrine Phenylpropanolamine Pseudoephedrine Terbutaline Theophylline |
Methylxanthines, caffeine, and theophylline are not sympathomimetics per se but may produce many of the same clinical features from their β-adrenergic activity, adenosine antagonism, and phosphodiesterase inhibition. , Although most acute cases of caffeine toxicity would be limited by undesirable effects with increasing dose, the risk for potentially life-threatening caffeine exposures is higher than ever with accessible concentrated caffeine powders (as herbal supplements) and caffeine-laden energy drinks. Methylxanthine toxicity is characterized by protracted emesis, palpitations, chest pain, tachycardia, and tachydysrhythmias—most commonly supraventricular tachycardia. , A wide pulse pressure results from peripheral vasodilation, and hypokalemia occurs via an intracellular potassium shift. Neurologic symptoms range from headache to refractory seizures. Antiemetic therapy with 5-hydroxytryptamine antagonists is recommended, but phenothiazines are contraindicated and may worsen toxicity. Norepinephrine and phenylephrine are vasopressors of choice. Severe hypotension might best be treated with a β-antagonist. Because the offending agent is still present, electrocardioversion for supraventricular tachycardia will be transient, as will adenosine (adenosine antagonism). Judicious use of benzodiazepines is recommended to treat seizures, tachycardia, hypertension, and agitation. Consider a calcium channel or β-receptor antagonist for arrhythmias. , Methylxanthines are dialyzable; in the case of theophylline, multiple dose–activated charcoal is strongly recommended to remove the drug from the system. ,
Designer amphetamines, such as methylenedioxyamphetamine and its derivatives, release serotonin and may precipitate hallucinations as well as the sympathomimetic effects listed earlier. Affected patients are at risk for seizures, dysrhythmias, hyperthermia, rhabdomyolysis, the syndrome of inappropriate secretion of antidiuretic hormone resulting in hyponatremia, and disseminated intravascular coagulation. , , Since the early 2000s, designer amphetamine exposures have been increasing. This group now includes cathinone derivatives, referred to as bath salts (in an attempt to mask their status as illicit substances), which can be consumed, smoked, or injected. Synthetic cannabinoids, referred to as spice , may cause symptoms similar to tetrahydrocannabinol (THC), the active ingredient in marijuana, with mild exposure. , Like THC, synthetic cannabinoids bind cannabinoid receptors. However, they do so at a higher affinity and, because synthetic cannabinoids are usually sprayed on plant material, additional unknown substances may be present. Many cases of synthetic cannabinoid exposure appear sympathomimetic in nature and should be treated as cases involving an amphetamine. Reports exist for both bath salts and spice of significant morbidity and mortality describing severe psychomotor agitation, aggressive psychotic behavior, seizures, tachycardia, hypertension, dysrhythmias, hyperthermia, and myocardial infarction. ,
To reduce CNS catecholamine release and eliminate severe hypertension, tachycardia, agitation, and muscle overactivity, benzodiazepines are considered first (potentially large doses). β-Adrenergic antagonists may paradoxically worsen hypertension from resulting unopposed α-adrenergic receptor stimulation and should be avoided (except in the case of methylxanthines, in which appropriate use may relieve symptoms). A vasodilator can be used for hypertension refractory to benzodiazepines. However, it must be short acting, as cocaine and similar agents may deplete norepinephrine, leading to cardiovascular collapse. , , Benzodiazepines should be used as first-line agents to manage seizures. Phenytoin does not treat toxin-induced seizures; in the case of methylxanthines, it may worsen patient outcome by lowering the seizure threshold.
Anticholinergic agents
The anticholinergic, or antimuscarinic, toxidrome is elicited by agents that possess antimuscarinic properties as their primary effect or as a side effect. Muscarinic receptors are found in the CNS, target organs of the parasympathetic nervous system (PNS), and sweat glands (sympathetic nervous system). This syndrome may have features similar to the sympathomimetic toxidrome. Some anticholinergic agents and features of the anticholinergic toxidrome are listed in Table 126.2 . The anticholinergic patient will exhibit dry skin rather than increased diaphoresis, as seen with sympathomimetic toxicity. Catheter placement may be necessary to address significant urinary retention, and dilated pupils are usually nonreactive as a result of associated cycloplegia (also not seen with sympathomimetic toxicity). ,
Anticholinergic Agents | Anticholinergic Toxidrome Features |
---|---|
Antihistamines (e.g., diphenhydramine, hydroxyzine) Atropine Benztropine mesylate Carbamazepine Cyclic antidepressants Cyclobenzaprine Hyoscyamine Jimsonweed Oxybutynin Phenothiazines Scopolamine Trihexyphenidyl | Agitation Delirium Coma Mydriasis Dry mouth Warm, dry, flushed skin Tachycardia Hypertension Fever Urinary retention Decreased bowel sounds |
It is critical to monitor core temperature and actively cool hyperthermic patients because sweating is inhibited in the face of anticholinergic toxicity. Consider benzodiazepines first to treat agitation and prevent heat production from excessive muscle activity. Although physostigmine is a cholinesterase inhibitor that may reverse central and peripheral manifestations of anticholinergic toxicity, case reports describing convulsions or asystole preclude its use for the anticholinergic manifestations of tricyclic antidepressant overdose—it is contraindicated in this setting. , , Consultation with a medical toxicologist is warranted to determine the right patient and right time for physostigmine, when clinical benefit outweighs the risk of morbidity and mortality.
Cholinergic agents
Cholinergic agents are best divided into the following three categories: muscarinic agents, nicotinic agents, and cholinesterase inhibitors. Muscarinic agents act in the CNS, at postganglionic parasympathetic nerve endings, and in the sweat glands. Nicotinic agents act in the CNS, in the autonomic ganglia (both sympathetic and parasympathetic), and at the neuromuscular junction. Cholinesterase inhibitors increase acetylcholine in the cholinergic synapse to present a combination of symptoms resulting from action at both nicotinic and muscarinic receptors. , Agents with cholinergic activity and signs and symptoms of cholinergic excess are listed in Boxes 126.2 and 126.3 .
Inhibitors of acetylcholinesterase
Organophosphate insecticides (malathion, parathion, diazinon)
Carbamate insecticides (aldicarb, carbaryl, propoxur)
Nerve agents (e.g., soman, sarin, tabun, Vx, cyclosarin)
Drugs used for myasthenia gravis or reversal of neuromuscular blockade (e.g., physostigmine, pyridostigmine, neostigmine, edrophonium)
Direct muscarinic agonists
Bethanechol
Carbachol
Methacholine
Pilocarpine
Muscarinic mushrooms (e.g., Clitocybe spp., Inocybe spp.)
Nicotinic agents
Nicotine
Water hemlock
Nicotine produces salivation, nausea, and vomiting, but significant exposure results in hypotension, bradycardia, and respiratory depression, often preceded by tachycardia, hypertension, and tachypnea. Central features include initial stimulation followed by seizures, lethargy, coma, and neuromuscular blockade. Management of nicotine poisoning is entirely supportive, with consideration of atropine for bradycardia, close monitoring, and aggressive respiratory support. Children are at increased risk of nicotine poisoning from ingestion of cigarettes or chewing tobacco; exposure to smoking cessation products (gums, lozenges, patches, and inhalers); and, more recently, exposure to concentrated liquid nicotine associated with electronic cigarettes (e-cigarettes). Because liquid nicotine intended for vaporization and inhalation is in a highly concentrated form when used with e-cigarette cartridges or in vials for refillable e-cigarettes (10 to >24 mg/mL), very small exposures may cause toxicity, usually seen at doses greater than 30 mg. These concentrates are easily absorbed through the skin and GI tract, rendering most decontamination useless. Symptoms usually appear within 15 to 30 minutes, although patients without significant respiratory symptoms at 4 hours are unlikely to show symptoms beyond this time frame. There is no antidote for nicotine toxicity. , ,
Direct-acting muscarinic agents produce excessive parasympathetic activity resulting in salivation, lacrimation, urination, diuresis, GI upset (including diarrhea), and emesis (often referred to as SLUDGE ). Cholinesterase inhibitors, organophosphorus pesticides, and nerve agents produce a mixed picture of toxicity by binding and inactivating acetylcholinesterase to prevent normal termination of cholinergic stimulation at postsynaptic receptors. In the PNS and CNS, the result is excessive nicotinic and muscarinic activity and, at the neuromuscular junction, depolarizing neuromuscular blockade. Over time the acetylcholinesterase becomes phosphorylated and weak hydrogen bonds change to covalent bonds, a process referred to as aging . Not all organophosphorus agents age at the same rate. Some nerve agents age within a few minutes, whereas some pesticides take as long as 72 hours to permanently inactivate the cholinesterase—activity is then restored only by synthesis of new enzyme. , Conversely, carbamates reversibly bind acetylcholinesterase. This enzyme/chemical complex undergoes spontaneous hydrolysis, generally restoring cholinesterase function within hours. Furthermore, carbamates do not penetrate the CNS well; thus, central manifestations are usually less severe. Because both organophosphates and carbamates cause bronchorrhea, bronchospasm, decreased respiratory drive, and paralysis of the muscles involved in breathing, death results from respiratory failure. , With dermal exposure, initial local hyperhidrosis is followed by systemic effects once the agent is absorbed. However, with inhalation, upper airway manifestations and respiratory distress occur rapidly. Following ingestion, drooling and vomiting are the early signs of toxicity.
Atropine is used to reverse the muscarinic effects of cholinergic toxicity, whereas an oxime is provided to reverse neuromuscular blockade, and benzodiazepines are used to treat seizures. Extremely large doses of atropine are provided to resolve bronchorrhea; tachycardia and pupil size are not indicative of an end point of atropine therapy. , Tachycardia during therapy is limited and not life-threatening, and even patients with initial tachycardia should receive atropine to address pulmonary secretions. With some agents, repeated doses or a constant infusion should be considered. , Oximes prevent cholinesterase aging. Pralidoxime is the oxime available and indicated for organophosphate poisoning in North America. The use of an oxime in conjunction with atropine is “atropine-sparing”—reactivation of affected cholinesterases decreases the amount of atropine necessary to combat life-threatening symptoms. Pralidoxime is generally not indicated in carbamate overdose because of its more limited toxicity. Benzodiazepines should be considered for seizure prophylaxis. Cholinesterase levels are not available in real time and should not be used to guide therapy when they will delay treatment.
Methemoglobinemia
In hemoglobin, oxidization of iron from the ferrous (2+) to the ferric (3+) state results in the formation of methemoglobin, an abnormal hemoglobin unable to transport oxygen. By shifting the oxygen saturation curve to the left, it decreases off-loading of any bound oxygen at the tissue level. Box 126.4 lists xenobiotics that may cause oxidative stress and result in methemoglobinemia. Methemoglobin maintains a darker hue than deoxyhemoglobin, causing the patient to look cyanotic in color. However, it does not improve with oxygen administration—the blood may have a chocolate color even after exposure to oxygen. Multiple-wavelength co-oximetry must be used to diagnose methemoglobin because standard pulse oximetry, used to measure oxyhemoglobin and deoxyhemoglobin, cannot reliably assess the degree of methemoglobinemia. Rather, standard pulse oximetry may overestimate or underestimate true oxygen saturation, generally reading in the 75% to 80% range as the methemoglobin level increases. , Methemoglobinemia should be suspected in a patient who appears cyanotic but has a normal partial pressure of oxygen (PO 2 ).
Benzocaine
Dapsone
Inhaled nitric oxide
Lidocaine
Naphthalene (found in certain mothballs)
Nitrates
Nitrites
Nitroprusside
Phenazopyridine
Prilocaine
Sulfonamides
Patients may appear blue with methemoglobin concentrations as low as 15 g/L, but a decision to treat is based on clinical symptoms, not the level. Treatment is not always necessary. Anemic patients have less hemoglobin to convert to methemoglobin, limiting their cyanotic appearance. However, because this is usually a larger percentage of their functioning hemoglobin, they are more likely to express symptoms of hypoxia at lower methemoglobin levels. Treatment begins with the administration of 100% oxygen (to maximize oxygen-carrying capacity by ensuring that unaffected hemoglobin is fully saturated). If necessary, this is followed by intravenous methylene blue at a dose of 1 mg/kg. Methylene blue accelerates reduction of the oxidized heme iron of methemoglobin to its normal state via nicotinamide adenine dinucleotide phosphate–dependent methemoglobin reductase. Response is rapid, generally within 30 minutes. Depending on the inciting agent, as in the case of dapsone, recrudescent methemoglobinemia may require repeated doses of methylene blue. The total dose should not exceed 7 mg/kg because methylene blue is itself oxidizing and may cause additional methemoglobinemia or precipitate hemolysis. Methylene blue may be ineffective in patients with glucose-6-phosphate dehydrogenase (G6PD) deficiency and may increase the risk of hemolysis or methemoglobinemia in this population. In patients not responding to methylene blue, G6PD deficiency should be suspected. In nonresponding patients who are severely ill, exchange transfusion should be considered. ,
Xenobiotic-induced hyperthermia
In addition to the previously described syndromes, sympathomimetic and anticholinergic, three distinct xenobiotic-induced hyperthermic syndromes deserve attention. Distinctions of malignant hyperthermia, serotonin syndrome, and neuroleptic malignant syndrome are provided in Table 126.3 . Although differences exist, there is also potential for overlap; diagnosis may be difficult when no previous patient history is available. In all cases of severe hyperthermia, other causes—such as sepsis—should be ruled out. Because hyperpyrexia in these scenarios is not hypothalamic in origin, antipyretics are ineffective.
Syndrome | Causative Agent | Timing of Onset | Treatment |
---|---|---|---|
Malignant hyperthermia | Depolarizing neuromuscular blockers or inhalational anesthetics | Minutes | Dantrolene |
Serotonin syndrome | Coadministration of two or more serotonergic agents | Hours | Supportive care, cyproheptadine |
Neuroleptic malignant syndrome | Antipsychotic drugs | Days | Supportive care, bromocriptine |
Malignant hyperthermia, a genetically determined condition, results in rigidity and muscle damage following exposure to depolarizing neuromuscular blocking agents (succinylcholine) or inhalational anesthetic agents. This life-threatening condition of ryanodine receptor dysfunction results in excessive intracellular calcium in somatic cells and therefore persistent muscle rigidity. Along with aggressive cooling, dantrolene should be administered without delay. Dantrolene allows muscle relaxation through blockade of calcium release from the sarcoplasmic reticulum.
Serotonin syndrome is a constellation of features resulting from excessive serotonergic activity in the CNS, most commonly associated with therapeutic regimens of two or more drugs that increase CNS serotonin transmission by different mechanisms. In addition to hyperpyrexia, hallmark features are altered mental status, excessive muscle activity, and autonomic instability. Suggested diagnostic criteria include a history of exposure to a serotonergic agent(s) and the presence of three of the following: mental status change, agitation, myoclonus, hyperreflexia, diaphoresis, shivering, tremor, diarrhea, incoordination, or fever. As opposed to other hyperthermic syndromes, it is distinguished by lower limb rigidity and hyperreflexia. , A simple algorithm for the diagnosis of serotonin syndrome can be found in Box 126.5 . Symptoms, starting within hours of exposure, are short lived, and mild cases of serotonin toxicity are self-limiting. However, patients with severe toxicity may develop extreme hyperthermia and rhabdomyolysis with renal failure and cardiovascular collapse. Supportive treatment includes withdrawal of all serotonergic agents, benzodiazepines for muscle overactivity, and aggressive cooling. The use of cyproheptadine has been proposed as a serotonin antagonist at doses from 16 to 32 mg divided up to four times daily. In cases of severe hyperthermia, cyproheptadine will do little to prevent further symptoms and should be abandoned for more aggressive therapy with cooling in addition to benzodiazepines or barbiturates and muscle paralysis with a nondepolarizing paralytic.
In the presence of a serotonergic agent
IF (spontaneous clonus = yes) THEN serotonin toxicity
ELSE IF (inducible clonus = yes) AND (agitation = yes) OR (diaphoresis = yes) THEN serotonin toxicity = YES
ELSE IF (ocular clonus = yes) AND (agitation = yes) OR (diaphoresis = yes) THEN serotonin toxicity = YES
ELSE IF (tremor = yes) AND (hyperreflexia = yes) THEN serotonin toxicity = YES
ELSE IF (hypertonic = yes) AND temperature >38°C) AND (ocular clonus = yes) OR (inducible clonus = yes) THEN serotonin toxicity = YES
ELSE serotonin toxicity = NO
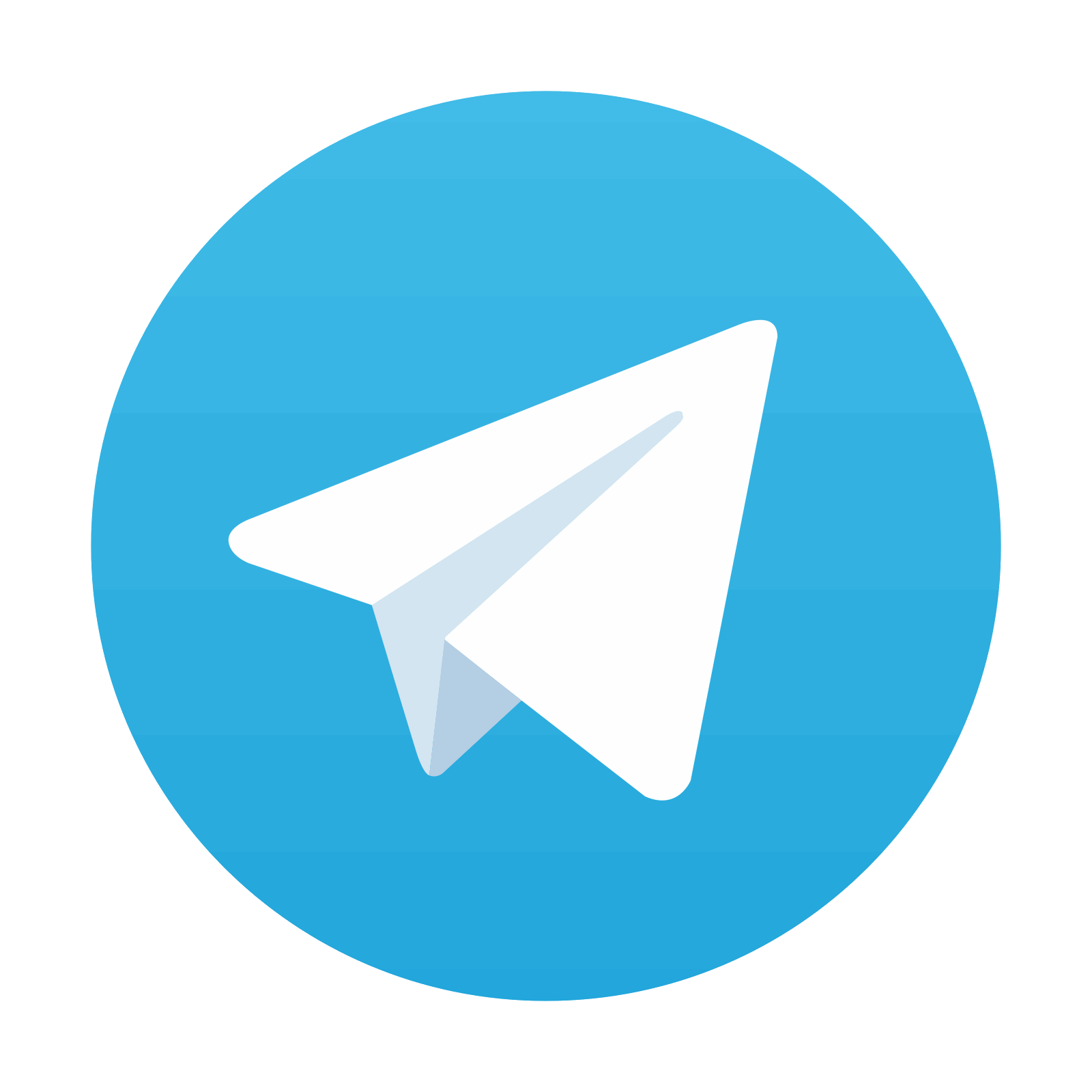
Stay updated, free articles. Join our Telegram channel

Full access? Get Clinical Tree
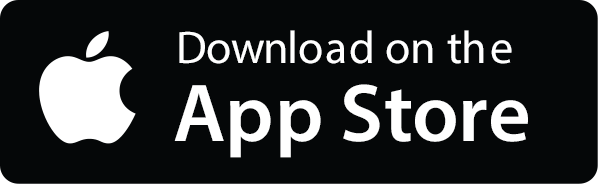
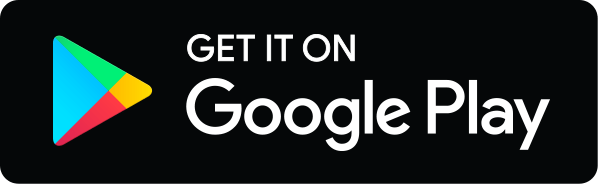
