Total Elbow Arthroplasty Design
April D. Armstrong
Graham J. W. King
Ken Yamaguchi
HISTORICAL BACKGROUND
Prosthetic elbow joint replacement was first described by Mellen and Phalen in 1947 when they replaced the distal portion of the humerus with an acrylic hemiarthroplasty (1). Other hemiarthroplasty designs followed; however, the results of all of these implants were disappointing (Fig. 20-1) (2, 3, 4, 5). Problems with limited motion, instability resulting from inadequate ligamentous support, and early loosening developed. Because of this, resection and interposition arthroplasty persisted as the preferred treatment for complicated intraarticular elbow problems.
Dee in 1972 (6) was the first author to describe a total elbow arthroplasty by implanting a constrained prosthesis with bone cement in 12 rheumatoid patients (Fig. 20-2). The prosthesis was a chrome-cobalt hinged joint that was articulated by the means of an axis pin. The humeral component was designed with a long shank that required a significant amount of distal humeral bone resection, thus destabilizing any surrounding soft tissues (7). The ulnar component consisted of a platform that rested on the olecranon and housed the distal humeral component. With an average follow-up of 14 months, 10 out 12 patients were reported to have excellent results (greater than 90 degrees motion, power 4 or greater, and no pain) (6). Souter in 1973 (7) and Silva in 1976 (8) provided other earlier accounts of their experience with constrained total elbow arthroplasty. Souter (7) showed promising short-term results but cautioned that early loosening had occurred in a significant proportion of his patients and that this procedure was fraught with a significant number of complications. He questioned the longevity of the procedure and cautioned that a much longer follow-up period was required before a thorough assessment could be completed.
Total elbow arthroplasty was beginning to evolve as a viable option for patients with debilitating elbow pain; however, high loosening rates for fixed hinge (constrained) total elbow arthroplasty were being reported (7,9,10). Garrett and colleagues (9) in 1977 reported painful loosening (50% with 3- to 5-year follow-up) as the primary long-term problem following implantation of GSB constrained hinge total elbow prosthesis. Morrey and colleagues (10) in 1981 reported aseptic loosening in 29/73 total elbow arthroplasties for the constrained Mayo and Coonrad prostheses. Ten of these patients required a revision procedure. Loosening of the humeral component was recognized first, followed by the ulnar component.
In the late 1970s, a “loose hinge” strategy for prosthetic articulation took form as new biomechanical studies
indicated that the elbow joint did not function as a true hinge (11, 12, 13, 14). The native ulnohumeral joint was shown to have a small amount of rotational and side-to-side motion throughout the arc of flexion and extension. The high early loosening rate of the fully constrained hinged arthroplasties was attributed to the fact that all of the forces were being absorbed by the prosthesis and its interface, leading to loosening. It was postulated that the surrounding soft tissues with the use of a semi-constrained hinged prosthesis, or more recently referred to as a linked prosthesis, should dissipate some of these forces (Fig. 20-3). Morrey and colleagues (15) in 1992 reported no radiographic evidence of loosening in 58 modified (sloppy hinge) Coonrad elbow implants at a mean follow-up of 3.8 years. The prosthesis allowed approximately 8 degrees of varus-valgus laxity and 8 degrees of internal-external rotation. An anterior extension was subsequently added to the humeral component to resist posterior displacement and axial rotational stresses. The distal part of the humeral component and the proximal part of the ulnar component were also treated with either a titanium plasma spray, beaded surface, or polymethylmethacrylate precoat to improve implantcement fixation. The humeral and ulnar components were coupled together with a pin that was secured with a split ring (16).
indicated that the elbow joint did not function as a true hinge (11, 12, 13, 14). The native ulnohumeral joint was shown to have a small amount of rotational and side-to-side motion throughout the arc of flexion and extension. The high early loosening rate of the fully constrained hinged arthroplasties was attributed to the fact that all of the forces were being absorbed by the prosthesis and its interface, leading to loosening. It was postulated that the surrounding soft tissues with the use of a semi-constrained hinged prosthesis, or more recently referred to as a linked prosthesis, should dissipate some of these forces (Fig. 20-3). Morrey and colleagues (15) in 1992 reported no radiographic evidence of loosening in 58 modified (sloppy hinge) Coonrad elbow implants at a mean follow-up of 3.8 years. The prosthesis allowed approximately 8 degrees of varus-valgus laxity and 8 degrees of internal-external rotation. An anterior extension was subsequently added to the humeral component to resist posterior displacement and axial rotational stresses. The distal part of the humeral component and the proximal part of the ulnar component were also treated with either a titanium plasma spray, beaded surface, or polymethylmethacrylate precoat to improve implantcement fixation. The humeral and ulnar components were coupled together with a pin that was secured with a split ring (16).
![]() Figure 20-1 Early hemiarthroplasty elbow replacement design. (Reprinted from Morrey BF, ed. The Elbow and Its Disorders, Third ed. Toronto: W.B. Saunders Company, 2000, with permission.) |
An alternative strategy to improve the problem of implant loosening was the development of improved unconstrained or unlinked prostheses. This type of prosthesis resurfaces the distal humeral and proximal ulnar surfaces with no hinge attachment. Stability is afforded by the geometry of the implant and the surrounding soft tissues. The premise of this prosthetic design was that the forces across the elbow joint would be largely diverted from the bone-implant interface to the surrounding soft tissues. Unfortunately, the trade-off of this design for a decreased loosening rate was a higher instability rate. Ewald and colleagues (17) in 1980 reported on 54 cases of an unlinked prosthesis, the capitellocondylar design, with an average follow-up of 3.5 years (Fig. 20-4). The humeral component was made of cobalt chrome alloy, and the ulnar component was made of high-density polyethylene. The prosthesis was designed so that a minimum amount of bone was resected from each joint surface. They reported elbow dislocation in 9% of cases in this preliminary experience (five elbows). There was no reported loosening of the humeral component. Davis and colleagues (18) in 1982 reported a 13% postoperative subluxation rate in 30 capitellocondylar total elbow arthroplasties. Because the design of the articulating surface of capitellocondylar prosthesis is shallow and less constraining than the native elbow, the stability of this prosthesis was highly dependent on the surrounding soft tissues, particularly the muscles crossing the elbow joint, which act to compress the articular surfaces (19).
More recently, unlinked designs that more closely replicate the normal anatomy of the elbow have been developed. The Souter-Strathclyde (Fig. 20-5) and Sorbie-Questor (Fig. 20-6) are examples. Whereas the Sorbie-Questor closely matches the shape of the native articulation, the Souter-Strathclyde has deeper grooves of articulation (20). The Souter-Strathclyde has been described as a semi-constrained unlinked design. The problem
with this type of implant is that aseptic loosening yet again becomes an issue (20). The more constraining the articular surfaces becomes, the more important it is that the axis of rotation is restored and the soft tissues are balanced. Component malposition will result in an incongruent articulation and implant maltracking (21,22). Theoretically, the surrounding soft tissues would then be eccentrically loaded, leading to their insufficiency and increased forces transmitted to the bone-implant interface. Patients with compromised bone stock, significant malalignment, or inadequate surrounding soft-tissue structures are not good candidates for any unlinked design of total elbow implant.
with this type of implant is that aseptic loosening yet again becomes an issue (20). The more constraining the articular surfaces becomes, the more important it is that the axis of rotation is restored and the soft tissues are balanced. Component malposition will result in an incongruent articulation and implant maltracking (21,22). Theoretically, the surrounding soft tissues would then be eccentrically loaded, leading to their insufficiency and increased forces transmitted to the bone-implant interface. Patients with compromised bone stock, significant malalignment, or inadequate surrounding soft-tissue structures are not good candidates for any unlinked design of total elbow implant.
![]() Figure 20-5 The Souter-Strathclyde total elbow system. (Reprinted from Morrey BF, ed. The Elbow and Its Disorders, Third ed. Toronto: W.B. Saunders Company, 2000, with permission.) |
More recently, the design of total elbow arthroplasty has focused on prostheses that offer the option of either being linked or unlinked. In some designs, additional focus has been placed on the radiocapitellar articulation with the option of replacing the radial head or not. A convertible implant may potentially provide the surgeon with the best of both designs. Revisions of unstable unlinked designs could be easily managed with a simple conversion to a linked design.
ANATOMIC CONCERNS
Restoration of normal elbow kinematics and stability may further improve the longevity of total elbow arthroplasty. More recently, the design of total elbow arthroplasty has focused on replicating the normal anatomic axis of the elbow. The rationale has been that if the normal elbow anatomic axis is restored, then in turn normal kinematics will follow. With normal kinematics, implant longevity may be improved as tension is restored to the surrounding soft tissues, thus minimizing the loads seen at the bone-cement interface and reducing the incidence of implant loosening.
The capitellum and trochlea make up the distal articular surface of the humerus (Fig. 20-7A). The articular surface is angulated 30 degrees anteriorly and tilts in 6 to 8 degrees of valgus with respect to the long axis of the humerus. In the transverse plane, the distal humeral articulation is rotated internally 5 to 7 degrees, referenced to a line bisecting the medial and lateral epicondyles. With the elbow extended and supinated, the angle formed by the humerus and the forearm is called the carrying angle. This is on average 10 degrees in males and 13 degrees in females (23). During flexion, the elbow moves from a valgus to varus alignment (23).
The ulnar articulation closely approximates the deep groove of the trochlea (Fig. 20-7C). The proximal aspect of the ulna consists of a greater sigmoid notch that articulates with the trochlea. Together, the proximal ulna and trochlea form the ulnohumeral articulation of the elbow. This articulation is responsible for flexion and extension motion of the elbow joint. The opening of the greater sigmoid notch
angles 30 degrees posterior to the long axis of the ulna, matching the 30-degree anterior tilt of the distal humerus (24). In the coronal plane, the ulna is angled in 1 to 6 degrees of valgus, contributing to the normal valgus carrying angle of the elbow (24). In earlier designs of total elbow arthroplasty, the normal valgus angulation of the ulna was not taken into consideration and was thought to be one of the reasons why these implants failed or became unstable (17,25).
angles 30 degrees posterior to the long axis of the ulna, matching the 30-degree anterior tilt of the distal humerus (24). In the coronal plane, the ulna is angled in 1 to 6 degrees of valgus, contributing to the normal valgus carrying angle of the elbow (24). In earlier designs of total elbow arthroplasty, the normal valgus angulation of the ulna was not taken into consideration and was thought to be one of the reasons why these implants failed or became unstable (17,25).
The radial head articulates with the capitellum and forms the radiocapitellar articulation of the elbow (Fig. 20-7B). The radial head also articulates with the proximal ulna at the lesser sigmoid notch forming the proximal radioulnar joint. Rotatory motion of the forearm, pronation and supination, occurs through these articulations. A line that bisects the center of the radial head and the fovea of the distal ulna forms the axis of rotation (26,27). The radius rotates around the ulna. If this articulation is not matched anatomically with a total elbow implant, then limitation of pronation and supination may result.
Replication of the radial head anatomy is difficult. For this reason, many total elbow implants have simply excised the radial head and ignored the radiocapitellar and proximal radioulnar articulations. The radial head is angulated 15 degrees with respect to the radial neck and shaft (24,26). King and colleagues (28) have shown that the normal radial head is not circular but also does not have a consistent elliptical shape. They also showed that the radial head was offset from the long axis of the radial neck. Radial head implants with a circular design, therefore, may not restore normal kinematics and potentially alter joint contact patterns. It was suggested that head asymmetry might be compensated for by not rigidly fixating the intramedullary stem of the prosthesis. The radial head
implant would then not be completely conforming, thus allowing for some toggle of the radial head to cope with the mismatch in head shape. Alternatively, a bipolar radial head has been developed. Both strategies may result in better contact of the radial head to the capitellum and lesser sigmoid notch. King and colleagues (28), in addition, concluded that a poor correlation was found for radial head size and canal diameter size. Modularity of the head and stem components was suggested. These same considerations for an isolated radial head hemiarthroplasty must also be taken into consideration when designing a radial head component for total elbow arthroplasty.
implant would then not be completely conforming, thus allowing for some toggle of the radial head to cope with the mismatch in head shape. Alternatively, a bipolar radial head has been developed. Both strategies may result in better contact of the radial head to the capitellum and lesser sigmoid notch. King and colleagues (28), in addition, concluded that a poor correlation was found for radial head size and canal diameter size. Modularity of the head and stem components was suggested. These same considerations for an isolated radial head hemiarthroplasty must also be taken into consideration when designing a radial head component for total elbow arthroplasty.
The elbow joint is commonly referred to as a hinge joint. However, a better descriptor to characterize elbow motion is a “loose hinge” (14,29). Morrey and Chao (14) have shown that out-of-plane motion occurs during elbow flexion; the forearm rotates internally during the early part of flexion and externally during the later part. A tight locus of instant centers of rotation measuring 2 to 3 mm was defined (Fig. 20-8). Thus the motion of the elbow joint during flexion was not truly uniaxial. The approximate anatomic axis of rotation was described to pass through the center of the arcs of the trochlea and capitellum, in the plane of the anterior border of the humerus (24). The anterior-inferior aspect of the medial epicondyle may be used as a surface landmark to identify the center of the arc of the trochlea (30). Bottlang and colleagues (29) more recently described an average screw displacement axis that penetrated the anterior-inferior aspect of the medial epicondyle, the center of the trochlea, and the center of the capitellum. The screw displacement axis varied 2.6 to 5.7 degrees in orientation and 1.4 to 2.0 mm in translation. It is likely that restoration of the normal anatomic axis of rotation for the elbow should normalize the length-tension relationships of the surrounding soft-tissue structures. The original fully constrained hinge design of total elbow arthroplasty failed to take into consideration this normal pattern of elbow motion, resulting in excessive force transmission to the bone-cement interface and early aseptic failure (10,31,32). Latter “loose hinge” designs improved aseptic loosening rates (15). O’Driscoll and colleagues (33) have shown that a “loose hinged” elbow prosthesis behaved as a semi-constrained joint under physiologic conditions, allowing the muscles to absorb some of the forces and moments that would have normally been transferred to the bone-cement interface in a fully constrained implant.
![]() Figure 20-8 The motion of the elbow joint is not truly uniaxial. (Reprinted from Morrey BF, Chao EY. Passive motion of the elbow joint. J Bone Joint Surg Am 1976;58:501-508, with permission.) |
Normal stability of the elbow is provided by both the bony articulations and the surrounding soft-tissue structures. Morrey and An (34) have shown that the osseous ulnohumeral articulation provided 31% and 33% of the resistance to valgus stress at full elbow extension and 90 degrees elbow flexion, respectively. In resisting varus stress, more support was provided by the bony articulation: 55% with the elbow extended and 75% with the elbow at 90 degrees of flexion. An and colleagues (35) have shown that varus and valgus stability of the elbow progressively decreased with increasing loss of the proximal ulna. If the normal articular anatomy of the elbow is restored with an anatomic unlinked total elbow arthroplasty, one-third or more of the inherent stability of the implant may be achieved. Implants with a more shallow articular design lose this advantage. It has been shown that the compressive forces of muscle activation and the surrounding soft tissues are more important for stability in implants with a less-constraining articulating surface (19). However, the disadvantage of a more conforming implant, with exact replication of the anatomy, was that precise surgical technique was critical to ensure that the implants were properly aligned. If a more conforming implant design was malpositioned, this could result in an incongruent articulation and maltracking, leading to increased polyethylene wear, eccentric loading of the soft tissues, and resultant accelerated aseptic loosening or instability.
The soft-tissue stabilizers of the elbow can be divided into static and dynamic stabilizers. The static stabilizers include the capsule and the medial and lateral collateral ligament complexes. The dynamic stabilizers include all of the surrounding musculature. The anterior capsule is more important in providing stability to the elbow only when in full extension (34). Additionally, the capsule cannot impart initial stability with a total elbow arthroplasty because it is compromised usually with the surgical approach or is incompetent secondary to an associated inflammatory condition. The lateral ulnar collateral ligament of lateral ligamentous complex is the major stabilizer to resist elbow varus and posterolateral rotation (Fig. 20-9) (21,36, 37, 38, 39, 40). The radial collateral ligament also plays an
important role in posterolateral rotatory instability of the elbow by virtue of its attachment to the annular ligament (40,41). The lateral collateral ligament complex (lateral ulnar collateral ligament and radial collateral ligament) is considered isometric and inserts on the lateral epicondyle, which coincides with the anatomic axis of rotation (27).
important role in posterolateral rotatory instability of the elbow by virtue of its attachment to the annular ligament (40,41). The lateral collateral ligament complex (lateral ulnar collateral ligament and radial collateral ligament) is considered isometric and inserts on the lateral epicondyle, which coincides with the anatomic axis of rotation (27).
The anterior bundle of the medial collateral ligament (MCL) complex has been shown to be the most important valgus stabilizer of the elbow (Fig. 20-10) (34,42, 43, 44, 45, 46, 47, 48, 49, 50, 51, 52, 53). The anterior bundle tightens in reciprocal fashion so that the anterior part is tight in extension and the posterior part is tight in flexion, indicating that it is not isometric (27,45). The attachment of the anterior bundle of the MCL at the sublime tubercle can be injured during preparation for insertion of the ulnar component (21,54). The ideal total elbow arthroplasty design should aim to duplicate the normal length-tension relationship of the collateral ligaments. The instrumentation for implantation of the prosthesis should also spare these ligament attachments or allow for anatomic reattachment.
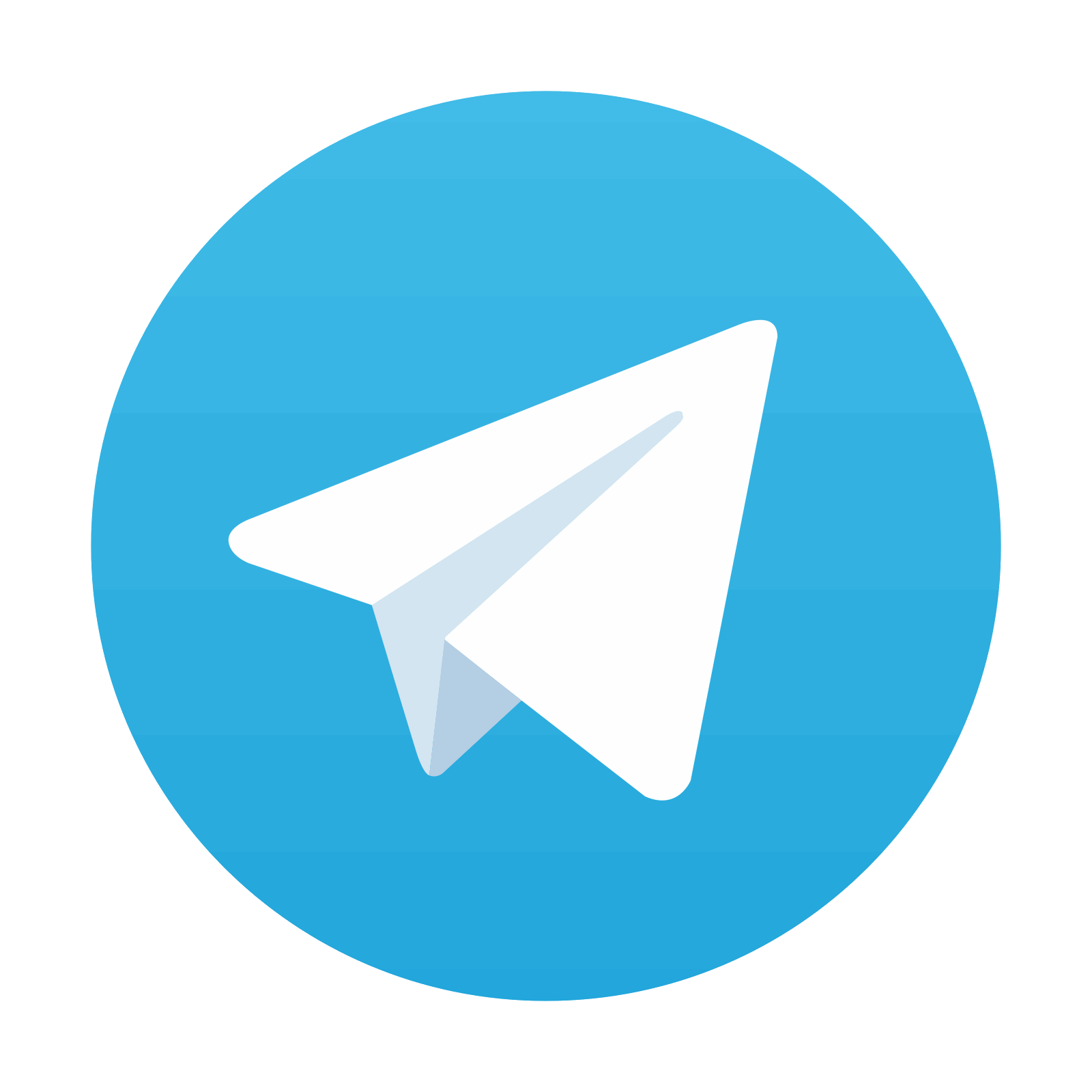
Stay updated, free articles. Join our Telegram channel

Full access? Get Clinical Tree
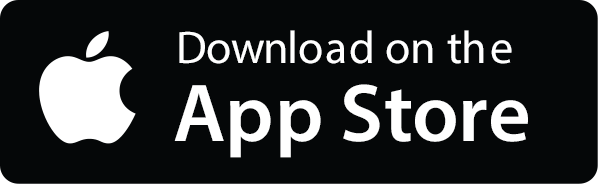
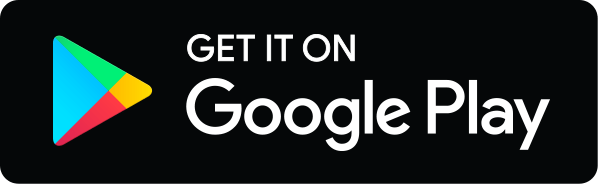