Introduction
The mesially inclined mandibular second molar can be supported upright by the microimplant anchorage. This study established the finite element model to analyze the displacement trend and periodontal ligament (PDL) stress distribution of the uprighting mandibular second molar with the microimplant under different conditions.
Methods
A 3-dimensional model of the mandible and dentition was established. The mesial inclination of the mandibular second molar was 30°, 45°, and 60°. Microimplants were implanted between the buccal side of the second premolar and the first molar and in the distal part of the mandibular second molar, respectively. Six groups were set, each loaded with 0.5 N of force. The second molar initial displacement trend and PDL stress distribution were evaluated.
Results
The PDL stress of mandibular second molars in all groups was within the physiological limit, and the PDL stress of mandibular second molars in the distal implant groups was lower than that of mandibular second molars in the mesial implant groups. PDL stress concentration in the cervical area. Tooth displacement decreased as the mesial inclination angle of the second molars increased. The sagittal displacement of mesial implant groups was larger, and there was a tendency of mesiobuccal torsion when standing uprighting; the vertical displacement of distal implant groups was larger, and there was a tendency of distal lingual torsion when standing uprighting.
Conclusions
Distal microimplant has a better extrusion effect on the mesially inclined second molar, whereas mesial microimplant has a better effect on the distal movement. The optimal orthodontic force for microimplant traction on mesially inclined second molars is 0.5-0.8 N.
Highlights
- •
Finite element analysis of uprighting mesially inclined molar with microimplant.
- •
The insertion position of the microimplant determines the effectiveness of the treatment.
- •
The optimal orthodontic force for uprighting mesially inclined molar is 0.5-0.8 N.
Because of increasingly refined dietary habits, the development of teeth and mandibles are not completely matched, and the incidence of mesially impacted mandibular second molars has been increasing in recent years. Second molar impaction mainly occurs in the mandible, without obvious gender difference. The prevalence of impaction of permanent mandibular second molars was reported to be 0.0%-2.3% globally and 1% in the Chinese population, but it is higher in orthodontic patients, about 2%-3%.
The development of tooth germs of permanent molars starts from the junction of the maxillary tubercle and the mandibular body. The mesial impaction of mandibular second molars is caused by many factors, such as inadequate space for eruption, premature loss of a primary molar leading to the excessive distance between the first and second molars, and a lack of guidance by the distal root of the first molar, and the obstructed eruption because of pathology (eg, odontoma, dentigerous cyst). , , The correct construction of the molar is crucial to the masticatory function. The mesial inclination of the mandibular second molar will lead to dental pulp and periodontal problems for itself and its adjacent mesial teeth and will also affect the height of one third of the surface and the vertical occlusal distance.
Orthodontic uprighting, surgical uprighting, and extraction of the affected teeth are all methods to treat molar inclination. Orthodontic uprighting molars are more acceptable to patients because of their minimal invasive trauma. The common orthodontic methods for uprighting mesially inclined molars can be divided into 2 types: tooth anchorage, including various Nitinol springs and segmental arches, and microimplant bone anchorage. Orthodontists usually insert microimplants in the premolar area or the retromolar area. A distalizing and uprighting movement was produced, which avoided the undesirable movement of the other molars.
Therefore, in this study, microimplants were inserted between the mandibular second premolar and first molar and at the retromolar area to upright different inclination molars. The biomechanical effects of microimplants at different positions on uprighting molars were analyzed to select appropriate methods and orthodontic force for uprighting mesially inclined second molars with different inclinations in clinical practice.
Material and methods
A 21-year-old female subject was selected for our study. Inclusion criteria were (1) Angle Class Ⅰ molar and canine relationships, (2) Standard tooth anatomic morphology, (3) normal development of tooth root morphology and length, and (4) no periodontal disease. Written informed consent has been signed by the subject. This study was approved by the ethics committee of Stomatology Hospital of China Medical University. This study selected the posterior border of the right mandible to the mesial of the mandibular right first premolar as the research scope. The head helical cone-beam computed tomography was performed using the iCAT cone-beam computed tomography system (KaVo 3D eXam; KaVo Dental, Bismarckring, Germany) at these settings: 37.1 mA, 120 kVp, the scan time of 17.8 seconds, voxel size of 0.3 mm, axial slice thickness of 0.3 mm, and field of view of 23×17 cm, with a resolution of 768 × 768 pixels. The digital imaging and communications in medicine data were imported into the Mimics software (version 20.0; Materialise, Leuven, Belgium), and the models of the mandible, mandibular first premolar, first molar, and second molar were reconstructed according to the threshold settings. These initial models were imported into the reverse engineering software Geomagic Studio (3D System, Rock Hill, SC) for surface optimization.
Subsequently, we adjusted the matching relationship of each part in NX software (NX 12; Siemens, Nuremberg, Germany) and established six 3-dimensional (3D) models ( Fig 1 , C ). In groups A, B, and C, the mandibular second molars inclined mesially by 30°, 45°, and 60° in turn, and microimplants were inserted at an angle of 20° with the alveolar bone surface at a distance of about 5 mm from the alveolar crest top on the buccal side of the second premolar and the first molar. The mandibular second molars in groups D, E, and F inclined mesially by 30°, 45°, and 60° in turn, and microimplants were inserted in the distal 5 mm of the second molars perpendicular to the bone surface. The parameters of the microimplant in all groups were 10.0 mm in length and 1.5 mm in diameter.

All 3D models were imported into the Ansys software (version 19.2; Ansys, Canonsburg, Pa). Isotropic, homogeneous, and continuous linear elastic material properties with small deformation conditions as defined in this study. Material parameters were derived from previous investigations and listed in Table I . Meshing each model using 10-node tetrahedral elements. Each model consisted of about 30,000 elements through the convergence test in our study. All models were established with 328,367, 328,002, 328,178, 328,148, 328,002, and 328,178 elements sequentially ( Fig 1 , D ).
Material | Young’s modulus (Mpa) | Poisson’s ratio |
---|---|---|
Cortical bone | 13,700 | 0.26 |
Cancellous bone | 1370 | 0.30 |
Tooth | 20,000 | 0.30 |
PDL | 0.68 | 0.49 |
Buccal tube | 210,000 | 0.30 |
Miniscrew | 103,000 | 0.33 |
The degree of freedom of the inferior margin of the mandible was assumed to be 0 in all 3 directions of x, y, and z, which was considered fixed. There was a bonded contact constraint between teeth, brackets, buccal canals, periodontal membranes, roots, alveolar bones, and microimplants. The first premolar, second premolar, and first molar of the mandible have a bonded contact relationship without relative sliding. A three-dimensional coordinate system was constructed in which the x-axis corresponds to the mesial-distal direction, the y-axis to the buccolingual direction, and the z-axis to the crown-root direction. The positive x value was defined as the mesial direction, positive y as the lingual direction, and positive z as the crown direction. All groups set the loading force to 0.5 N with a loading force duration of 1 second under all working conditions. , The origin of force also differed, as uprighting was achieved by (A, B, C) using an elastomeric chain or (D, E, F) using an open coil spring and a 0.016-in stainless steel uprighting spring between the implant and the molar ( Fig 2 ). Von Mises stress and displacement of the mandibular second molar on each model were analyzed.

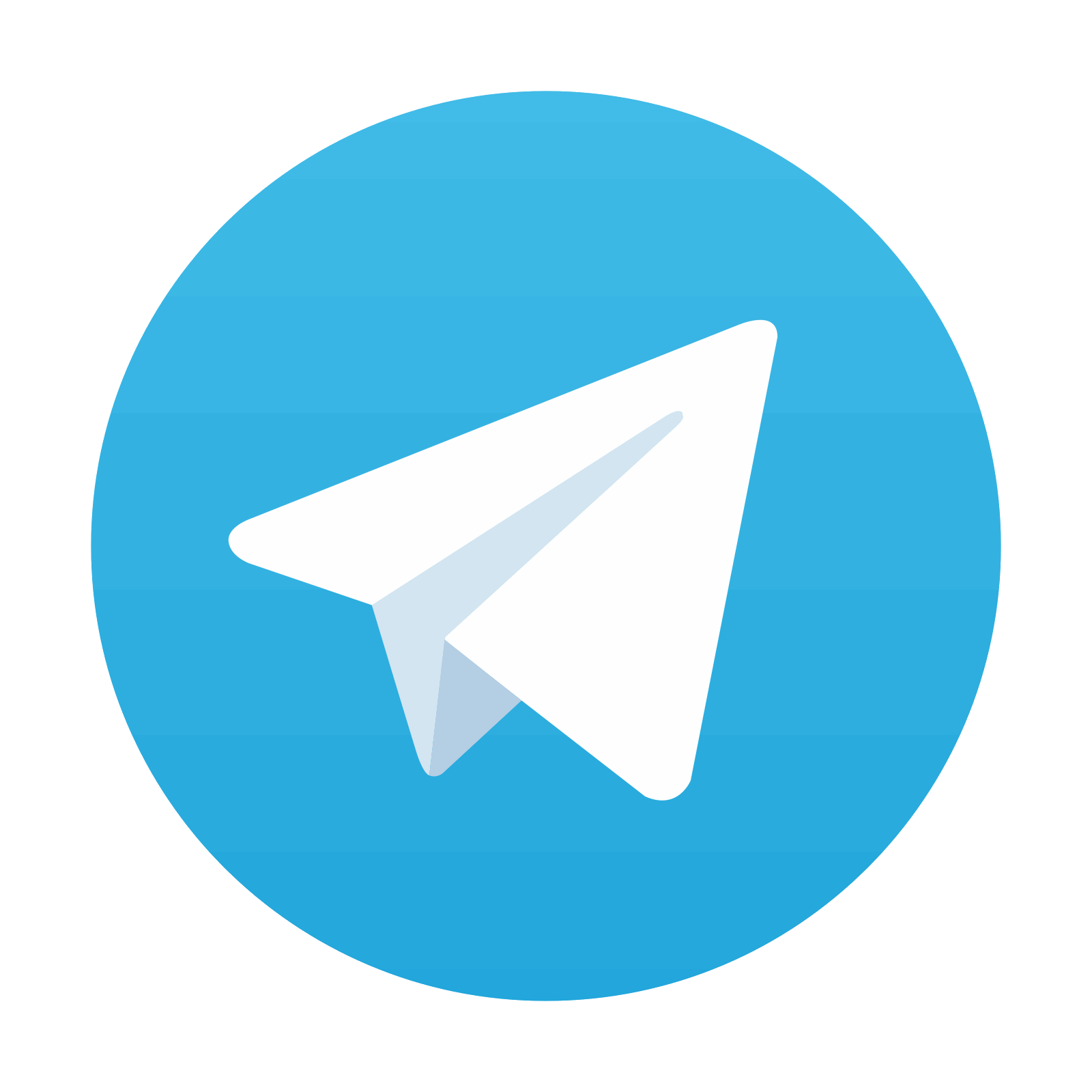
Stay updated, free articles. Join our Telegram channel

Full access? Get Clinical Tree
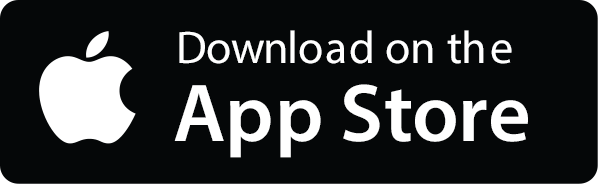
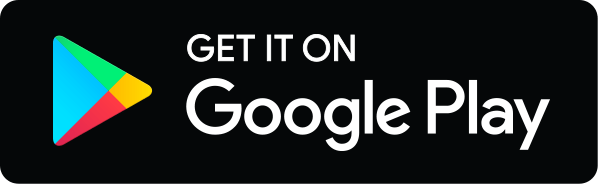
