Thoracic and Lumbar Trauma
Chambliss C. Harrod
Devan Moody
Gregory D. Schroeder
Alexander R. Vaccaro
Thoracolumbar fractures are common injuries affecting over 700,000 individuals annually. Fractures can range from low-energy osteoporotic compression fractures to high-energy fracture-dislocations. Approximately, 15,000 major thoracolumbar fractures occur in the United States annually, with approximately one-third of these injuries causing significant neurologic damage. While the term “thoracolumbar” is used with differing meaning in the literature, for the purposes of this chapter, it can be anatomically arranged into the following regions:
Upper (T1–T9)
Thoracolumbar junction (T10–L2)
Lower lumbar (L3–L5)
Sixteen percent of thoracolumbar fractures occur between T1 and T10, 52% occur between T11 and L1, and 32% occur below L1. Plain radiographs often are the first imaging modality used to diagnose fractures. However, computed tomography (CT) is most commonly utilized in polytrauma patients to identify fracture morphology. Magnetic resonance imaging (MRI) provides additional information regarding discoligamentous integrity, neural elements, and presence of hematoma.
Nonoperative treatment is typical for neurologically intact patients with stable fracture patterns. However, newer classification systems have aided in both surgical decision-making and approach selections in patients with unstable injuries to allow adequate stabilization, neural decompression and protection, and deformity realignment or maintenance. Controversy still remains regarding operative versus nonoperative management, timing of surgical intervention, optimal approach selection(s), construct length, complication management, management of osteoporotic vertebral compression fractures, and the utilization of evolving surgical techniques (e.g., minimally invasive surgery and cement augmentation).
Thoracolumbar Anatomy
Normal kyphosis of the thoracic spine is produced by the wedge shape of the vertebral bodies (i.e., they are taller posteriorly than anteriorly), which ranges from 20 to 50 degrees. In contrast, the lordosis of the lumbar spine ranges from 40 to 70 degrees (average 50 degrees) and is principally created by the intervertebral disks, being taller anteriorly than posteriorly. Unique to the thoracic spine is its anatomic continuity with the rib cage and sternum, providing significant additional stiffness. This stiffness contrasts with the more mobile lumbar spine, and thus produces a transition zone at the thoracolumbar junction (T10–L2). This zone is marked by the loss of ribs, and a transition from a small thoracic spinal canal diameter to a larger lumbar canal diameter. Concurrently, the facet joint orientation transitions from coronal (thoracic) to sagittal (lumbar). This portion of the spine is distinctly “straight” from T10–T11 through L1–L2.
Six percent of all fractures involve the spine with 90% involving the thoracic and lumbar regions. Most occur in the T10–L2 transitional area. In the presence of high-energy mechanisms there is a 6:1 ratio of complete/incomplete neurologic injuries. The spinal cord generally terminates around the L1–L2 interspace. Thus, in addition to being the structural transition area, the thoracolumbar spine is also a neurologic transition area. Injuries at the conus medullaris and the cauda equina levels have a more favorable prognosis than at cranial spinal cord areas. This is because nerve roots, which are peripheral nerve structures, are generally more resilient with improved capacity for recovery compared to the spinal cord, which is part of the central nerve system. Because of this and other factors, minimal correlation has been demonstrated between the degree of neurologic injury and the degree of canal compromise.
Initial Treatment and Examination
Initial evaluation should begin with the ABCs (airway, breathing, circulation) following the Advanced Trauma Life Support (ATLS) protocol with early attention to life-threatening airway disorders, ventilation (pneumothorax, etc.), and cardiovascular injuries with appropriate support followed by identification of additional injuries (particularly noncontiguous spine injuries). A cervical collar should be placed and any extremity injuries splinted when the airway is secured.
A focused history with attention to trauma mechanism, location of pain, presence of incontinence, history or presence of spondyloarthropathy (e.g., diffuse idiopathic skeletal hyperostosis [DISH] or ankylosing spondylitis [AS]), prior spine surgery, and the presence of pacemakers or other metallic foreign body contraindicating the use of MRI should be identified. Some nonspine injuries, such as calcaneal fracture, frequently occur concomitantly with thoracolumbar burst fractures and thus should raise suspicion of spinal injury. Flexion moments potentiated by wearing lap seatbelt may injure abdominal viscera in addition to causing a spine injury (e.g., Chance-type fracture). Patients with stiff spines (e.g., patients with DISH or AS) often have minimally or nondisplaced fractures as a result of an extension distraction mechanism that may nonetheless be unstable injuries. These fractures are often missed on initial clinical and radiographic evaluations and can result in neurologic decline due to instability, neurologic compression, and symptomatic epidural hematoma, the incidence of which is especially increased in coagulopathic patients.
Physical examination begins with complete exposure of the patient with logroll and examination of the dorsal surfaces of the spine looking for ecchymosis, induration, or other indicators of significant spine instability. Complete neurologic examination including motor, sensory, and reflexes with careful rectal assessment (voluntary rectal tone, bulbocavernosus reflex, anal wink reflex, etc.) to identify sacral nerve root function is performed and should be calculated according to the American Spinal Injury Association (ASIA) scale (Fig. 5.1). Total
absence of motor (Frankel system grades 0 to 5) and sensory function below the anatomic injury level denotes complete neurologic injury whereas incomplete injuries (Table 5.1) maintain residual cord or root function below the injury level. The ASIA has devised standards for describing spinal cord injuries based on motor and sensory levels in addition to the presence or absence of sacral sparing. Complete disruption of neurologic function in the torso and extremities causes flaccid paralysis after spinal cord injury and is often referred to as spinal shock. Spinal shock ends with the return of the bulbocavernosus reflex, which indicates that the arc between the pelvic afferent nerves and sacral cord efferent nerves is once again intact. Hemodynamic status should be carefully assessed to detect neurogenic or hypovolemic shock to allow appropriate treatment with volume resuscitation with vasopressors and chronotropic agents, as necessary, to treat neurogenic shock and maintain mean arterial pressures above 85 mm Hg.
absence of motor (Frankel system grades 0 to 5) and sensory function below the anatomic injury level denotes complete neurologic injury whereas incomplete injuries (Table 5.1) maintain residual cord or root function below the injury level. The ASIA has devised standards for describing spinal cord injuries based on motor and sensory levels in addition to the presence or absence of sacral sparing. Complete disruption of neurologic function in the torso and extremities causes flaccid paralysis after spinal cord injury and is often referred to as spinal shock. Spinal shock ends with the return of the bulbocavernosus reflex, which indicates that the arc between the pelvic afferent nerves and sacral cord efferent nerves is once again intact. Hemodynamic status should be carefully assessed to detect neurogenic or hypovolemic shock to allow appropriate treatment with volume resuscitation with vasopressors and chronotropic agents, as necessary, to treat neurogenic shock and maintain mean arterial pressures above 85 mm Hg.
Complete spinal cord injury patients have a worse prognosis for neurologic recovery than those with incomplete injuries. A recent study reported that 44% of patients with complete neurologic injury and 73% of patients with incomplete injury recovered at least one motor grade. The most important predictor of a favorable neurologic outcome is retention of sacral (S4–S5) sensation 72 hours to 1 week after the injury. Sacral sparing with intact pinprick sensation may suggest a favorable prognosis for recovery of bladder function whereas priapism suggests a complete spinal cord injury via loss of sympathetic tone with unregulated parasympathetic input.
Deep venous thrombosis prophylaxis is paramount. Use of intermittent external pneumatic compression devices, static compression stockings, and, in select patients, subcutaneous (5,000 U subcutaneously every 12 hours) or intravenous low–molecular-weight heparin helps to minimize potentially fatal pulmonary emboli.
Radiologic Evaluation
While plain radiographs are no longer the initial imaging of acute trauma victims in many large centers, they remain an important surveillance tool. Plain radiographic evaluation begins with anteroposterior (AP) and lateral views combined with swimmer’s views to visualize the cervicothoracic junction tend to define vertebral anatomy. The lateral view allows assessment of vertebral, facet, spinous process, and intervertebral foramina alignment. Loss of vertebral body height and cortical
margins denote compressive spinal injury patterns. Lateral radiographs also allow Cobb measurements of sagittal kyphotic deformities, translation anteriorly or posteriorly (>2.5 mm). Particular attention should be noted to the posterior vertebral line or angle as this can differentiate burst from compression fractures. AP views can demonstrate lateral vertebral body compression, interspinous process, and interpedicular widening. Close attention to the endplates on the AP view will also identify subtle injuries missed on lateral views. As indicated above, plain films are becoming increasingly less popular due to low sensitivities and the utility of screening CT scans for chest, abdomen, and pelvis. Regardless of the screening tool, plain, upright radiographs continue to have an important role in assessing the alignment of stable fractures treated nonoperatively in an orthosis once the patient is mobilized.
margins denote compressive spinal injury patterns. Lateral radiographs also allow Cobb measurements of sagittal kyphotic deformities, translation anteriorly or posteriorly (>2.5 mm). Particular attention should be noted to the posterior vertebral line or angle as this can differentiate burst from compression fractures. AP views can demonstrate lateral vertebral body compression, interspinous process, and interpedicular widening. Close attention to the endplates on the AP view will also identify subtle injuries missed on lateral views. As indicated above, plain films are becoming increasingly less popular due to low sensitivities and the utility of screening CT scans for chest, abdomen, and pelvis. Regardless of the screening tool, plain, upright radiographs continue to have an important role in assessing the alignment of stable fractures treated nonoperatively in an orthosis once the patient is mobilized.
TABLE 5.1 INCOMPLETE SPINAL CORD INJURY SYNDROMES | ||||||||||||||||||
---|---|---|---|---|---|---|---|---|---|---|---|---|---|---|---|---|---|---|
|
CT provides optimal characterization of bony fracture patterns with sagittal, coronal, and 3D reconstructions. In polytrauma evaluations, screening CT scans serve well for both visceral and bony injury. Inaba and coworkers demonstrated superior sensitivity and interobserver variability of reformatted CT scans compared with plain radiographs for localizing, classifying, and delineating the thoracolumbar spinal injuries (25% injuries are missed on radiograph alone with gross underestimation of burst spinal canal compromise). Abdominal CT is recommended when a flexion-distraction injury (FDI) is suspected to evaluate for intra-abdominal injury. The greatest disadvantage of CT is its limited sensitivity in showing consistently specific soft tissue injuries (disk herniation, epidural hematoma, ligamentous disruption, or spinal cord injury).
MRI is highly sensitive in detecting injury to the anterior discoligamentous structures and posterior ligament complex (PLC), epidural hematoma, pathologic fractures, or paraspinal masses. MRI has over 90% sensitivity and specificity for identifying supraspinous and interspinous ligament injury (Fig. 5.2). Once one spinal injury is detected, it is imperative to image the entire spine fully to identify noncontiguous injuries. A recent study demonstrated a 10-fold higher incidence of neurologic injury associated with missed TL fractures than those recognized at initial assessment. Extension distraction fracture dislocations in the patient with DISH or AS are particularly important to diagnose and prevent catastrophic neurologic decline due to significant instability or development of delayed epidural hematoma. Spinal cord edema is often noted as increased signal intensity on T2-weighted images. Hematoma is characterized by decreased signal intensity on T2-weighted images acutely and often is surrounded by a halo of T2-weighted enhancement from adjacent edema. Edema extending more than two vertebral levels and the presence of hematoma within the spinal cord are considered poor prognostic signs for potential functional motor recovery.
Classifications
Historically, thoracolumbar injuries were first classified by mechanism and injury severity. Most early classification systems focused on fracture mechanism and/or
morphology without accounting for ligamentous or neurologic injury. Holdsworth believed that the PLC ultimately determined stability at each segment and conceptualized the spine being composed of two columns (anterior/posterior). All posterior column injuries were hence unstable.
morphology without accounting for ligamentous or neurologic injury. Holdsworth believed that the PLC ultimately determined stability at each segment and conceptualized the spine being composed of two columns (anterior/posterior). All posterior column injuries were hence unstable.
![]() Figure 5.2 Midsagittal MRI T2-weighted image demonstrating T12 burst fracture with fragment retropulsion with distal cord edema and hemorrhage. |
TABLE 5.2 THE FERGUSON AND ALLEN CLASSIFICATION | ||||||||||||||||||||||||||||||||||||||||||||||||||||||||||||
---|---|---|---|---|---|---|---|---|---|---|---|---|---|---|---|---|---|---|---|---|---|---|---|---|---|---|---|---|---|---|---|---|---|---|---|---|---|---|---|---|---|---|---|---|---|---|---|---|---|---|---|---|---|---|---|---|---|---|---|---|
|
Ferguson and Allen presented a mechanistic classification of thoracolumbar injuries with seven injury patterns: compressive-flexion, distractive-flexion, lateral-flexion, translational, vertical-compression, and distractive-extension injuries (Table 5.2). The Denis classification hinges on the importance of the middle column of the spine (midvertebral body to posterior vertebral cortex) to overall spinal stability with the four types being:
Compression fracture
Burst fracture
Seatbelt fractures (Chance flexion-distraction injuries)
Fracture-dislocation
McAfee’s classification (wedge-compression, stable and unstable burst, Chance, flexion-distraction, and translational) arose in response to criticisms of Denis’ classification by utilizing CT to describe the mode of failure of the middle column. Thus, the classification emphasizes various injuries as stable/unstable with emphasis on the importance of the PLC. The “stable burst fracture” was coined by McAfee and involves the anterior and middle columns but has an intact PLC whereas unstable burst fractures involve disruption of the PLC. Chance fractures consist of a horizontal vertebral avulsion injury with the axis of rotation classically anterior to the ALL (though this can vary). The other two modes include flexion-distraction and translational injuries.
The Magerl classification is based on the three primary forces applied to the spine. Type A injuries are those caused by compressive loads, type B injuries are distractive injuries, and type C injuries are rotational and multidirectional. Each fracture type is divided into three subtypes depending on the severity of load applied and structure(s) compromised (bone vs. soft tissue). The classification provides rationale for determining treatment and prognosis but is limited due to its complex scheme yielding low interobserver reliability.
The Thoracolumbar Injury Classification System (TLICS) categorizes injuries by morphology (rather than by injury mechanism), posterior ligamentous injury (Fig. 5.3), and neurologic injury and has been found to have high interobserver reliability (Table 5.3). Points are assigned to each category. Morphology (based primarily on CT axial and reconstructed imaging) includes compression (1 point), burst (1 additional point), translation-rotation (3 points), and distraction (flexion or extension, 4 points) injuries. Ligamentous (PLC) disruption is assigned 0 (none), 2 (indeterminate), or 3 (definite) points while neurologic injury is assigned 0 (intact), 2 (complete), or 3 (incomplete, cauda equine injury) points. Patients with <4 points typically are treated nonoperatively (exceptions: AS, DISH, neurologic deficit), whereas, those with >5 points are operatively managed.
TLICS can also be used to suggest a surgical approach (anterior, posterior, or combined) primarily depending on presence of neurologic compromise (anterior decompression) and PLC disruption (posterior tension band
restoration). A recent retrospective review lent external validity to the TLICS system, which was tested outside of the founding institutions. TLICS correctly governed appropriate treatment (i.e., actual treatment) 98% of the time. This paper lends further credence to the TLICS as an easy and useful assessment scale in management of thoracolumbar trauma patients. Future efforts are being made to develop classification systems that reflect long-term assessment of patient centered outcomes following nonoperative versus operative treatment as well as reflective of cultural sensitivities of the treating physician and patient.
restoration). A recent retrospective review lent external validity to the TLICS system, which was tested outside of the founding institutions. TLICS correctly governed appropriate treatment (i.e., actual treatment) 98% of the time. This paper lends further credence to the TLICS as an easy and useful assessment scale in management of thoracolumbar trauma patients. Future efforts are being made to develop classification systems that reflect long-term assessment of patient centered outcomes following nonoperative versus operative treatment as well as reflective of cultural sensitivities of the treating physician and patient.
![]() Figure 5.3 Intraoperative dorsal photograph of patient in Figure 5.2 demonstrating disruption of posterior ligamentous complex. |
The newest classification is the AOSpine Thoracolumbar Injury Classification System. This system combines the advantages of the Magerl system with those of TLICS in an effort to develop a classification that can establish a globally accepted treatment algorithm. It divides fractures into three major types:
A—compression type injury
B—tension band injury
C—translational injuries
Type A injuries are further subdivided into five subtypes: A0—clinically insignificant fracture such as a transverse process fracture; A1—compression fracture involving one endplate, but not the posterior vertebral wall; A2—compression fracture or coronal split fracture involving both endplates, but not the posterior vertebral wall; A3—incomplete burst fracture involving one endplate and the posterior vertebral wall; A4—complete burst fracture involving both endplates and the posterior vertebral wall. B type injuries are further divided into three subtypes: B1—a boney injury to the tension band (i.e., boney Chance fracture); B2—a posterior tension band injury including the ligamentous structures; B3—an anterior tension band injury. Additionally, the patient’s neurologic status is graded, and patient-specific modifiers can be applied (Table 5.4). After almost ten studies were performed to help understand the controversial portions of the new classification, the Thoracolumbar AOSpine Injury Score (TL AOSIS) was developed (Table 5.5), as well as a surgical algorithm. The TL AOSIS assigns an integer value to each component of the injury. Injuries with
a score of three or less should undergo nonoperative care, whereas injuries with a score of more than five should be treated surgically. The ideal treatment for injuries with a score of four or five is unclear, and so this is left to the discretion of the surgeon and patient.
a score of three or less should undergo nonoperative care, whereas injuries with a score of more than five should be treated surgically. The ideal treatment for injuries with a score of four or five is unclear, and so this is left to the discretion of the surgeon and patient.
TABLE 5.3 THE THORACOLUMBAR INJURY CLASSIFICATION AND SEVERITY SCORE | ||||||||||||||||||||||||||||||||||||||||||||||
---|---|---|---|---|---|---|---|---|---|---|---|---|---|---|---|---|---|---|---|---|---|---|---|---|---|---|---|---|---|---|---|---|---|---|---|---|---|---|---|---|---|---|---|---|---|---|
|
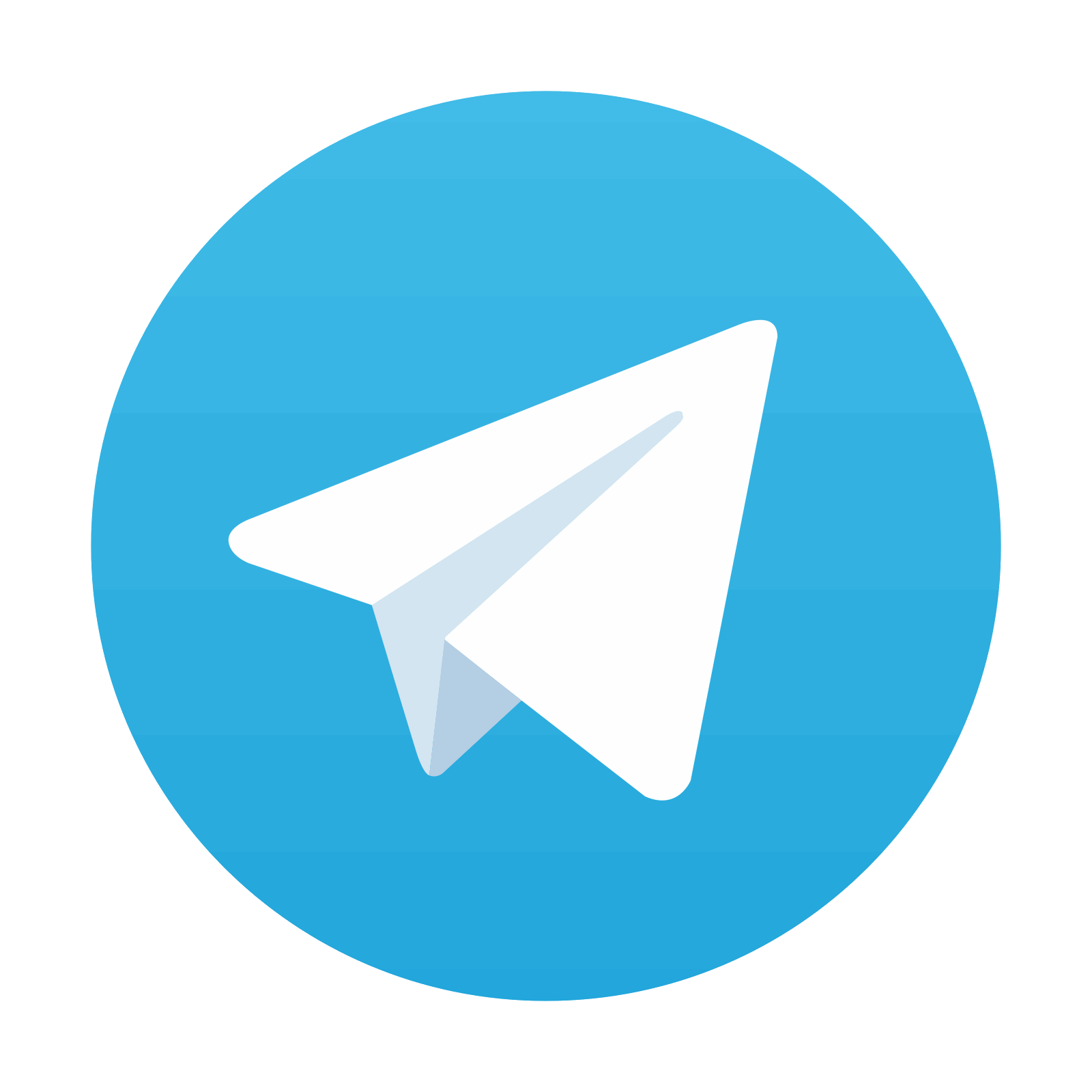
Stay updated, free articles. Join our Telegram channel

Full access? Get Clinical Tree
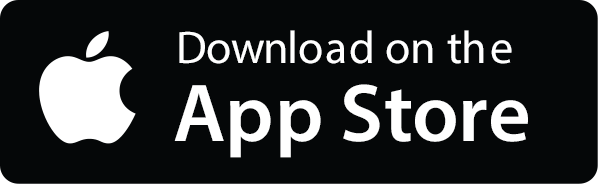
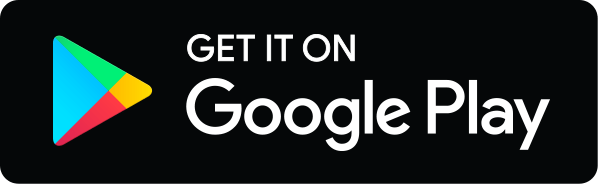