Recent advances in MRI have provided the opportunity to map changes in hemodynamics that correspond to cognitive and sensory operations. These advances in noninvasive, low-risk, imaging environments have extended the traditional role of medical imaging into new domains that include investigations into the interplay between brain anatomy, physiology, and function. This interplay is mandatory for examination of the complex effects of diffuse damage caused by traumatic brain injury. Functional MRI (fMRI) provides relatively high-resolution indirect assessment of neuronal activity. Three main factors interact to affect the quality of fMRI data that is acquired: (1) MRI hardware, (2) the paradigm (or experimental) design, and (3) subject cooperation. This article focuses on paradigm design and subject cooperation.
Recent advances in MRI have provided the opportunity to map changes in hemodynamics that correspond to cognitive and sensory operations . These advances in noninvasive, low-risk, imaging environments have extended the traditional role of medical imaging into new domains that include investigations into the interplay between brain anatomy, physiology, and function. This interplay is mandatory for examination of the complex effects of diffuse damage caused by traumatic brain injury (TBI).
Functional MRI (fMRI) provides relatively high-resolution indirect assessment of neuronal activity. There are three main advantages of fMRI over other types of brain mapping: (1) the in-plane resolution can easily achieve 1.5 × 1.5 mm; (2) the time to acquire data can be short (3–4 minutes); and (3) the study does not require injection of external contrast agents or radioactive isotopes. The most common type of fMRI uses changes in the blood oxygen level dependent signal (BOLD) . The premise of BOLD fMRI lies in local and specific changes in T2 ∗ signal that result from neuronal activity. Following neuronal activity there is an increase in blood flow to the vasculature that is geographically close to that neuronal activity. This increase in blood flow results in a corresponding reduction in deoxyhemoglobin, which is paramagnetic. The reduction of deoxyhemoglobin alters the T2 ∗ signal . This change in the T2 ∗ signal, which occurs as an indirect result of local neuronal firing, is small (between 0.5% and 7% depending on the task design, strength of neuronal response, and magnet strength) and occurs in the presence of high background noise. The source of this change in T2 ∗ signal comes from a combination of extravascular and intravascular contributions. This small signal requires that average signal changes be calculated across multiple trials to increase the signal-to-noise ratio.
Three main factors interact to affect the quality of fMRI data that is acquired : (1) the MRI hardware, (2) the paradigm (or experimental) design, and (3) subject cooperation. A discussion of MRI hardware is outside of the scope of this article. The focus herein is on paradigm design and subject cooperation.
The considerations required for paradigm design include factors such as the choice of a blocked versus event-related (ER) design, the number of volumes required for data analysis, the TR (repetition time), the type of comparisons that will be analyzed, the type of data modeling, the length of time the subject or patient will be left in the scanner, and the difficulty of task.
Two main types of experimental designs are used in fMRI research: blocked and ER. There are advantages and disadvantages for both designs that differ depending on the target population and research question. Blocked designs generally require the subject to complete one task for a period of time (generally 30–45 seconds) and then alternate with one or more other tasks. For example, during a typical functional imaging series evaluating motor function, 100 images are acquired in a 5-minute run. The initial 10 images are baseline (in this case, rest) and are followed by 10 images (30 seconds) during which the patient is asked to tap his or her fingers at a rate of 1 tap/s. This pattern (rest, movement) is then repeated for the remaining 80 images. The beginning and end of each period is cued by a visual or auditory signal and occurs at images 10 and 20 and so on until 100. In this example, the entire motor network involved in finger tapping would be represented in the analysis. If the goal were to evaluate differential activity between right and left hand finger movement, one might eliminate the rest condition and instead alternate movement between the two hands.
These two examples illustrate a major experimental question, that is, what conditions will be compared in the analysis. This decision will affect not only the network that is presented in the analysis but also the statistical power. Generally, comparing an active condition with another active condition will require more statistical power than comparing an active condition with rest. In the two example paradigms described previously, one would expect to see supplementary motor regions when comparing motor with rest but would probably not expect to see this region when comparing two active and equivalent motor conditions.
Blocked designs offer better signal to noise than ER designs and generally offer more brain coverage. Because of the better signal-to-noise ratio, fewer volumes are required (translating to less time for data acquisition) than in most ER designs. The main disadvantage of blocked designs is the inability to remove incorrect answers (in memory, language, or attention paradigms) or to investigate separately the different networks that underlie correct versus incorrect responses.
There are two main types of ER designs—a slow ER design or a staggered timing design. In a slow ER design, one trial is presented and followed by an interval that generally ranges from 10 to 15 seconds. This design allows individual modeling of each hemodynamic response for each trial. In a staggered design, a trial is followed by a variable interval of generally 5, 7, or 9 seconds . The analysis then deconvolves the individual responses. ER designs generally provide better temporal resolution than blocked designs but have lower signal to noise and less brain coverage. The main advantage is the ability to model each response. In cognitive tasks versus tasks that target sensory functions, in which behavior is variable, this is a large advantage.
Subject cooperation
Imaging studies targeting patient populations must be concerned with subject cooperation and performance. Patients will generally be more anxious and fatigue more quickly during testing than will healthy controls. In addition, changes in cognitive function will affect cooperation and performance. Although these factors pose a challenge, usually they can be overcome by assimilating the patient to the MR environment, practicing on the tasks, choosing appropriately difficult materials, collecting physiologic data, and evaluating task performance. Additionally, factors such as caffeine consumption, the amount of sleep, and the time of testing will affect performance, although the effects of these factors on fMRI are not well established beyond the acknowledgment that they do affect activation.
Optimal subject performance generally can be obtained with adequate practice before the scan, the use of an MRI simulator (if available), and selection of a challenging paradigm that is not too difficult for the patient. At the author’s center, we require the use of a simulator. The patient practices the task in the simulator until he or she can complete it with minimal head motion over the course of the paradigm (less than one-half of 1 voxel).
Additionally, it is crucial to collect data during image acquisition that can affect the BOLD. At a minimum, behavioral data must be collected (eg, accuracy, latency, grip strength). Additionally, the use of an MR compatible eye tracker (eye movement monitoring equipment) will help assure the investigator that the patient is completing the task as expected. Additionally, the collection of cardiac, respiratory, and oxygen saturation can help in data interpretation and assist in data processing.
Considerations in the use of functional MRI in individuals who have a history of traumatic brain injury
One of the most important considerations in imaging patients with brain injury is establishing and thoroughly understanding the specific fMRI task as applied to normal control subjects. The control subjects should be matched to the patient population of interest in terms of demographics, especially age and years of education. Most often, the control subjects are right-handed individuals who report a negative neurologic history. There has been a recent movement to use a sample of patients with a recent injury, such as an orthopediatric injury but no head injury, as the control subject sample. This practice serves to potentially equalize the confounding emotional impact of a recent trauma on overall functioning. Obtaining adequate control subject data for the selected fMRI task will establish the expected activation network and the individual variation that occurs in the activation pattern. In addition, if the task will be used to determine treatment effectiveness or to gauge recovery, test-retest data should be obtained in a matched control group to establish task reliability across multiple administrations of the task . Fig. 1 presents an activation map of a matched control subject on the reading comprehension task used in a study involving patients with severe TBI. The expected activation pattern in terms of the location and extent of clustered activation voxels is demonstrated on the sentence comprehension task and compared with that in subjects with TBI before and after rehabilitation .
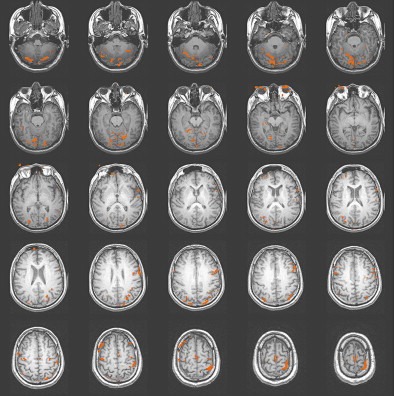
Selection of an fMRI task that is within the capabilities of individuals with TBI is essential. When the patient with TBI cannot accuracy and efficiently complete the task, interpretation of the activation data can be impossible. It is well known that subjects with brain injury generally have reduced performance relative to controls on neuropsychological tests. To examine the activation pattern elicited by a similar cognitive probe or fMRI task in the MR scanner, the subjects will need to demonstrate near-normal performance on similar neuropsychologic tests. Without this criterion, it will be difficult to characterize what mental activity is actually being performed during the imaging session in response to the cognitive challenge presented during the task. In addition, frustration with the task, especially when it is beyond the patient’s abilities, can result in aberrant activation patterns and movement. It is advisable to obtain the results of neuropsychologic testing from all potential subjects before imaging to determine the subject’s specific level of impairment in the cognitive domain to be addressed in the chosen fMRI task. For example, in the study mentioned previously, to ensure that the patients with severe TBI could read with sufficient fluency for the reading comprehension fMRI task, a reading fluency test from a standardized academic battery was administered before the imaging .
Another way to ensure that the subject with TBI can complete the specific fMRI task is to provide a training session using a similar task but with non-duplicated stimuli before imaging. Pretraining the subject on the task can reduce patient anxiety during the scanning and will most likely result in more predictable results. In general, control subjects will be able to tolerate cognitive tasks that are more difficult than those within the capabilities of patients with TBI. The aim of the neuropsychologic testing and pretraining on the activation task is to ensure that the performance accuracy in the patient and control subject pools is statistically equal. With equal accuracy, there is some assurance that the two groups can be compared with each other and that the resulting activation pattern is related to the effect of the brain injury and not different cognitive processes employed during imaging. Even when accuracy is equated, subjects with TBI often have slower processing speed. When the fMRI task stimuli is presented sufficiently slowly to allow patients with TBI to process the stimuli, control subjects will have extra time during the task that can result in noise in the data. This noise results from unwanted cognitive activities that are not task directed. Allowing the task to be self-paced, as an alterative strategy, generally results in a variable number of stimuli, again making comparisons between samples difficult. Furthermore, tolerance of extended time in the scanner will most likely be shorter for patients who have a TBI than for control subjects who have been selected for adequate attention and motivation. Excess time in the scanner will often result in unwanted head movement within the scanner, which can invalidate the fMRI results.
Patients with a history of moderate-to-severe brain injury often have abnormalities that will influence co-registration of their brain on an available standardized structural atlas. Normalization of the brain is essential in determining the location of the activation clusters and in comparing the patients with TBI with normal controls. When imaging patients with severe brain injury, Schiff and colleagues chose not to employ a normalized brain because of the significant morphologic changes and instead used landmarks such as the anteroposterior commissural line to assign anatomic labels. In addition, some researchers have attempted to mask-out or ignore parts of the brain with lesions or have selected regions of interest (ROI) within the brain that are outside the area of the lesions. Establishing a specific neuroanatomically defined ROI to examine may confound the comparison with normal controls because a change in the location of the cluster of activation may occur as an adaptation to the brain injury. As an alternative, the patient with a chronic brain injury can be used as their own control in the longitudinal study, and changes in activation pattern can be located on the patient’s co-registered Talairached structural image for each study. When using this technique, the patient should be within the chronic stage of recovery so that acute changes in the areas of brain injury would not be expected.
Studies of patients with TBI have determined that alternations in the vascular system can exist after TBI. Some researchers have reported a shut down of cerebral autoregulation following mild head injury , and a slowing of cerebral circulation was demonstrated by Taylor and Bell . Other researchers have demonstrated an increase in cerebral blood volume and an increase in arterial blood pressure . Given that fMRI studies are dependent on the change in blood flow to vasculature near the activation, alterations in the vascular system can influence the obtained activation network in terms of signal strength. In their study of patients with mild TBI, Chen and colleagues found a weaker BOLD signal within the frontal region but an increase in activation in the posterior cerebral regions in a majority of their subjects. In Fig. 2 , a subject 2 months following moderate TBI is shown initially to demonstrate enhanced posterior activation. At 4 months post TBI, a more even distribution of activation was demonstrated on an identical fMRI task .
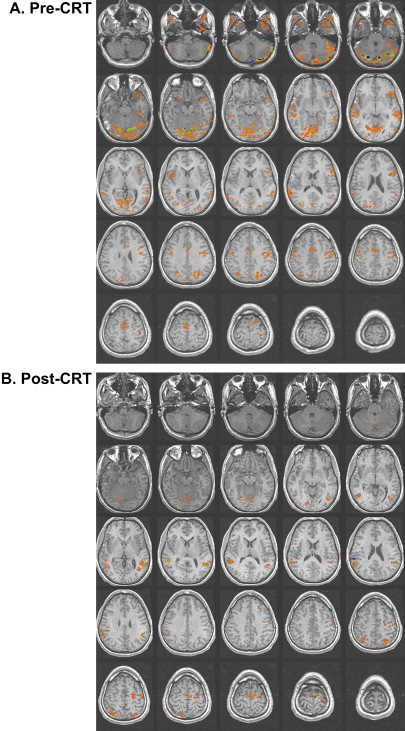
As well understood in neuropsychologic testing, depression and anxiety are common symptoms following TBI and can influence attention, memory, and the speed of processing. Many studies have demonstrated the relationship between emotional status and performance on neuropsychologic tests. Because fMRI tasks often involve a cognitive task, the absence of acute depression and anxiety will help to optimize performance and consistency in response to the task. High anxiety can cause head movement and distractability during the imaging, whereas depression may influence the patient’s ability to attend to the task stimuli. As part of a pre-scan evaluation, a measure of anxiety and depression is recommended to help the clinician understand the patient’s emotional state. In addition, the patient’s current medication should be closely examined. Medications that are known to enhance or depress cerebral blood flow should be avoided if possible. In a longitudinal rehabilitation study, consistency in medication treatment is an appropriate goal.
Repeating fMRI studies over time during periods of intervening rehabilitation or drug treatment is potentially useful in understanding recovery from all types of brain injury. Nevertheless, even when the patient with TBI is their own control, there are significant difficulties in determining whether longitudinal changes seen on the fMRI activation pattern are due to changes in the activation network used to complete the task, an increased familiarity with the task, changes in the strategy employed during the task, or a change in effort as a general response to rehabilitation. Using control subjects and repeating the imaging task longitudinally with the control subjects can help determine whether changes in activation are related to recovery or treatment effects and not just repetition of the fMRI task. Alternatively, the patient can be imaged multiple times before treatment as in a double-baseline research study.
Considerations in the use of functional MRI in individuals who have a history of traumatic brain injury
One of the most important considerations in imaging patients with brain injury is establishing and thoroughly understanding the specific fMRI task as applied to normal control subjects. The control subjects should be matched to the patient population of interest in terms of demographics, especially age and years of education. Most often, the control subjects are right-handed individuals who report a negative neurologic history. There has been a recent movement to use a sample of patients with a recent injury, such as an orthopediatric injury but no head injury, as the control subject sample. This practice serves to potentially equalize the confounding emotional impact of a recent trauma on overall functioning. Obtaining adequate control subject data for the selected fMRI task will establish the expected activation network and the individual variation that occurs in the activation pattern. In addition, if the task will be used to determine treatment effectiveness or to gauge recovery, test-retest data should be obtained in a matched control group to establish task reliability across multiple administrations of the task . Fig. 1 presents an activation map of a matched control subject on the reading comprehension task used in a study involving patients with severe TBI. The expected activation pattern in terms of the location and extent of clustered activation voxels is demonstrated on the sentence comprehension task and compared with that in subjects with TBI before and after rehabilitation .
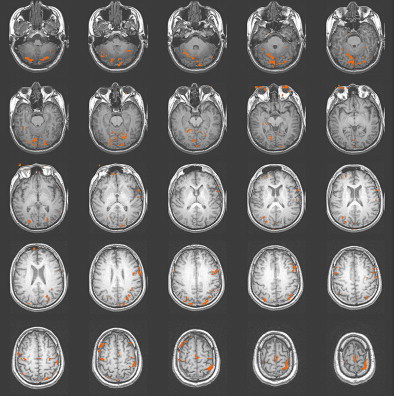
Selection of an fMRI task that is within the capabilities of individuals with TBI is essential. When the patient with TBI cannot accuracy and efficiently complete the task, interpretation of the activation data can be impossible. It is well known that subjects with brain injury generally have reduced performance relative to controls on neuropsychological tests. To examine the activation pattern elicited by a similar cognitive probe or fMRI task in the MR scanner, the subjects will need to demonstrate near-normal performance on similar neuropsychologic tests. Without this criterion, it will be difficult to characterize what mental activity is actually being performed during the imaging session in response to the cognitive challenge presented during the task. In addition, frustration with the task, especially when it is beyond the patient’s abilities, can result in aberrant activation patterns and movement. It is advisable to obtain the results of neuropsychologic testing from all potential subjects before imaging to determine the subject’s specific level of impairment in the cognitive domain to be addressed in the chosen fMRI task. For example, in the study mentioned previously, to ensure that the patients with severe TBI could read with sufficient fluency for the reading comprehension fMRI task, a reading fluency test from a standardized academic battery was administered before the imaging .
Another way to ensure that the subject with TBI can complete the specific fMRI task is to provide a training session using a similar task but with non-duplicated stimuli before imaging. Pretraining the subject on the task can reduce patient anxiety during the scanning and will most likely result in more predictable results. In general, control subjects will be able to tolerate cognitive tasks that are more difficult than those within the capabilities of patients with TBI. The aim of the neuropsychologic testing and pretraining on the activation task is to ensure that the performance accuracy in the patient and control subject pools is statistically equal. With equal accuracy, there is some assurance that the two groups can be compared with each other and that the resulting activation pattern is related to the effect of the brain injury and not different cognitive processes employed during imaging. Even when accuracy is equated, subjects with TBI often have slower processing speed. When the fMRI task stimuli is presented sufficiently slowly to allow patients with TBI to process the stimuli, control subjects will have extra time during the task that can result in noise in the data. This noise results from unwanted cognitive activities that are not task directed. Allowing the task to be self-paced, as an alterative strategy, generally results in a variable number of stimuli, again making comparisons between samples difficult. Furthermore, tolerance of extended time in the scanner will most likely be shorter for patients who have a TBI than for control subjects who have been selected for adequate attention and motivation. Excess time in the scanner will often result in unwanted head movement within the scanner, which can invalidate the fMRI results.
Patients with a history of moderate-to-severe brain injury often have abnormalities that will influence co-registration of their brain on an available standardized structural atlas. Normalization of the brain is essential in determining the location of the activation clusters and in comparing the patients with TBI with normal controls. When imaging patients with severe brain injury, Schiff and colleagues chose not to employ a normalized brain because of the significant morphologic changes and instead used landmarks such as the anteroposterior commissural line to assign anatomic labels. In addition, some researchers have attempted to mask-out or ignore parts of the brain with lesions or have selected regions of interest (ROI) within the brain that are outside the area of the lesions. Establishing a specific neuroanatomically defined ROI to examine may confound the comparison with normal controls because a change in the location of the cluster of activation may occur as an adaptation to the brain injury. As an alternative, the patient with a chronic brain injury can be used as their own control in the longitudinal study, and changes in activation pattern can be located on the patient’s co-registered Talairached structural image for each study. When using this technique, the patient should be within the chronic stage of recovery so that acute changes in the areas of brain injury would not be expected.
Studies of patients with TBI have determined that alternations in the vascular system can exist after TBI. Some researchers have reported a shut down of cerebral autoregulation following mild head injury , and a slowing of cerebral circulation was demonstrated by Taylor and Bell . Other researchers have demonstrated an increase in cerebral blood volume and an increase in arterial blood pressure . Given that fMRI studies are dependent on the change in blood flow to vasculature near the activation, alterations in the vascular system can influence the obtained activation network in terms of signal strength. In their study of patients with mild TBI, Chen and colleagues found a weaker BOLD signal within the frontal region but an increase in activation in the posterior cerebral regions in a majority of their subjects. In Fig. 2 , a subject 2 months following moderate TBI is shown initially to demonstrate enhanced posterior activation. At 4 months post TBI, a more even distribution of activation was demonstrated on an identical fMRI task .
