Introduction
Transdifferentiation of chondrocytes into bone cells explains most condylar growth during prenatal and early postnatal stages, but the mechanisms regulating chondrocyte transdifferentiation during late postnatal growth remain unknown. This study aimed to quantify the effects of dietary loading on chondrocyte-derived osteogenesis during late postnatal condylar growth.
Methods
Two compound mouse lines were used to trace the fate of chondrocyte lineage in vivo. Twelve 3-week-old male Aggrecan-Cre ERT2 (Acan Lineage ); R26R TdTomato ; 2.3 Col10a1-GFP and twelve 3-week-old male Col10a1-Cre (Col10a1 Lineage ); R26R TdTomato ; 2.3Col1a1-GFP were randomly divided into experimental (soft-food diet, n = 6) and control (hard-food diet, n = 6) groups and kept for 6 weeks. One time, tamoxifen injections were given to Acan Lineage mice at 3 weeks. Radiographic, microcomputed tomographic, and histomorphometric analyses were performed.
Results
Radiologic analysis showed that mice with a soft-food diet had smaller mandible lengths as well as decreased bone volume and density for their condylar process. Histologically, mice with soft diets had reduced activity in chondrocyte proliferation and maturation compared with the controls. Cell lineage tracing results showed the number of Acan Lineage -derived bone cells (293.8 ± 39.8 vs 207.1 ± 44.6; P = 0.005), as well as total bone cells (445.6 ± 31.7 vs 360.7 ± 46.9; P = 0.004), was significantly higher in the hard-diet group than in the soft-diet group, whereas the number of non-Acan Lineage -derived bone cells was not significantly different among groups ( P = 0.938). Col10a1 Lineage mice showed the same trend.
Conclusions
Dietary loading directly affects condyle chondrogenesis and chondrocyte transdifferentiation, which alters the extent of condylar growth and remodeling.
Highlights
- •
Chondrocytes are key players in postnatal condylar bone formation.
- •
Condylar cartilage responses to dietary loading by cell fate change.
- •
Soft diet may cause hyperdivergence by inhibiting chondrocyte transdifferentation.
Orthodontists need to better understand how and why the mandible adapts to environmental changes, which is essential for orthopedic intervention and its treatment effects. A decrease in masticatory strength because of softer diets has been suggested as a major culprit behind phenotypic mandibular variability. Hyperdivergent features observed in human patients, such as increased mandibular plane angles, gonial angles, and anterior face heights, have been directly linked to weaker masticatory muscles. Conversely, when exercises to strengthen the masticatory muscles are completed, the mandible adapts and illustrates phenotypic changes opposite to those of hyperdivergent subjects. These features have also been demonstrated in many animal studies by manipulating their diet. The subsequent changes in quality, size, and shape of cartilage and bone after the alteration of diet have led to the conclusion that dietary loading plays a significant role in the development of the craniofacial complex.
One reason for the adaptability of the mandible is the temporomandibular joint, specifically the mandibular condyle. It is composed of bone and a layer of secondary cartilage on its superior surface. This type of cartilage differs from primary cartilage in that it requires mechanical loading for its growth and reacts to stimuli for its maintenance. There is a clear association between soft diet and condylar growth. The studies on rodents have found decreased cartilage thickness along with reduced length and bone volume of the condylar process in the soft-diet group. However, it is unknown how cellular changes translate to these macroscopic changes and whether the adaptation of condylar cartilage to mechanical stimuli directly links to the reduction of bone in the condylar process.
Temporomandibular joint condyles grow via endochondral bone formation, beginning with mesenchymal cells that proliferate and differentiate into chondrocytes. As the cartilage grows, chondrocytes become entrapped and enter a hypertrophic state. It has been traditionally thought that the hypertrophic chondrocytes undergo apoptosis, followed by an invasion of cells from the underlying bone marrow that erode the calcified cartilage and initiate angiogenesis, bringing in cells that deposit new bone. Using cell lineage tracing, a powerful tool to trace cell fate in vivo, Jing et al found that up to 80% of bone cells in the condylar process arise via direct transdifferentiation of chondrocytes during prenatal and early postnatal development. This is also consistent with the results obtained from fetal and postnatal metaphyseal growth. Together, these studies demonstrated that chondrocytes play a critical role in endochondral bone formation and that chondrogenesis and osteogenesis are 1 continuous process. However, it is still unknown to what extent the condylar chondrocytes contribute to late postnatal condylar growth and whether dietary loading has a direct role in regulating condylar chondrocyte transdifferentiation.
This study aimed to determine (1) the role and contribution of chondrocyte-derived osteogenesis occurring during late postnatal condylar growth and (2) whether dietary loading affects the condylar bone by regulating the chondrocyte transdifferentiation. It was hypothesized that a consistent decrease in dietary loading would reduce chondrocyte differentiation and transdifferentiation, resulting in mandibular growth changes. Two Cre lines were used to trace the fate of chondrocytes: Aggrecan-Cre ERT2 was used to trace all chondrocytes and their descendants after tamoxifen administration at 3 weeks; Col10a1-Cre was used to trace hypertrophic chondrocytes and their descendants constitutively once its expression began around E14.5. The expression of 2.3Col10a1-GFP (a marker for osteoblasts and osteocytes ) was colocalized with cell lineage tracing to study chondrocyte transdifferentiation. If chondrocytes are revealed to be key players in condylar bone formation during late postnatal growth and directly respond to dietary loading, the focus of future studies should be shifted away from the bone marrow and instead be directed toward developing novel methods to control chondrocyte transdifferentiation. This could open new methods of research targeting condylar growth therapeutics and mandibular orthopedic therapies.
Material and methods
The experimental protocol was approved by the Institutional Animal Care and Use Committee of Texas A&M School of Dentistry (IACUC 2022-0124-CD; reference no. 116605). Twelve 3-week-old male Aggrecan-Cre ERT2 (Acan Lineage ); R26R TdTomato ; 2.3Col10a1-GFP and twelve 3-week-old male Col10a1-Cre (Col10a1 Lineage ); R26R TdTomato ; 2.3Col10a1-GFP were randomly divided into 2 groups. Each group (n = 6) maintained normal mice chow (hard diet, the control group) or gel food (soft diet, the experimental group, ClearH20 76A, ME) for 6 weeks ( Fig 1 , A ). Three-week-old mice are equivalent to prepubescent humans aged approximately 6-8 years.

One time tamoxifen (75 mg/kg) injections were given to Aggrecan-Cre ERT2 ; R26R TdTomato ; 2.3Col1a1-GFP mice at 3 weeks. All mice were killed when aged 9 weeks with EdU (10 μg/mL, A10044; Invitrogen, Carlsbad, Calif) administered 3 hours before harvest. The sample size was based on published estimates assuming a power of 0.95 and α of 0.05, with a 10% difference and effect size of 2.4.
Mandibles were fixated in 4% paraformaldehyde, dissected, and separated in half along the midsymphysis. Microcomputed tomography (μCT) scans (μCT 35 scanner; Scanco Medical AG, Bassersdorf, Switzerland) were taken to quantify the bone quality of the condylar process beyond the mandible foramen ( Fig 1 , B ). , The μCT 3-dimensional (3D) reconstruction of the hemimandibles was oriented consistently (to visualize all cusps of the first molar equally from the lingual view), and 2-dimensional (2D) images were captured of that orientation to measure total mandibular length, mandibular base length, ramus height, and condylar length with ImageJ (National Institutes of Health, Md) software by 1 examiner (A.J.) ( Fig 1 , C ; Table I ). , ,
Measurements | Units | Soft diet | Hard diet | P value |
---|---|---|---|---|
Total mandibular length (Co-Me) | mm | 9.53 ± 0.11 | 9.83 ± 0.16 | 0.004 |
Mandibular base length (Me-Go) | mm | 8.65 ± 0.26 | 8.92 ± 0.21 | 0.006 |
Ramus height (Co-GoT) | mm | 4.24 ± 0.15 | 4.36 ± 0.10 | 0.182 |
Condylar length (Co- ⊥Ic-Rd) | mm | 2.61 ± 0.07 | 2.63 ± 0.08 | 0.725 |
The condylar process was decalcified in 10% ethylenediaminetetraacetic acid, dehydrated in 30% sucrose, and sectioned along the frontal plane parallel with the axis of the ramus. The slides from the middle third portion were used for the study. The sections for analysis were selected by 1 examiner in which the mediolateral width of the condyle was the largest. The sections were used for Toluidine blue, Safranin O staining, or immunohistochemistry (IHC) with the following antibodies: Aggrecan (the major proteoglycan in the articular cartilage, 1:400; Abcam, Cambridge, United Kingdom), Col10a1 (Collagen type 10a1 expressed by hypertrophic chondrocytes, 1:400; Abcam), and Sox9 (a key transcriptional factor for chondrocyte differentiation, , 1:100; Abcam). Click-iT Imaging Kit with Alexa Flour 647-azide (A10277; Invitrogen) was used to detect EdU signal in cryosections for cell proliferation analysis. Toluidine blue and Safranin O images were captured by a light microscope, and fluorescent images were captured using an SP5 Leica confocal microscope (Leica, Germany) at wavelengths ranging from 488 ( green ) to 561 ( red ) nm.
For chondrogenic activity, the EdU + chondrocytes (EdU + cells/area), the expression levels of Aggrecan, Col10a1 (marker + /area), and Sox9 in cartilage (Sox9 + cells/area) were quantified using ImageJ software. , Chondrocyte transdifferentiation was evaluated in the superior and inferior portions of the subchondral bone separately ( Fig 1 , D ). The superior portion included the subchondral bone above the widest portion of the condyle, whereas the inferior portion was 250 μm thick, immediately below the widest portion of the condyle as viewed in the frontal orientation. The following parameters were quantified in the superior and inferior regions, as well as the combination of both areas: the numbers of Acan Lineage or Col10a1 Lineage -derived bone cells ( yellow and red ), the number of nonchondrocyte-derived bone cells ( green and blue ), and the ratio of Acan Lineage or Col10a1 Lineage -derived bone cells over all subchondral bone cells to represent the percentage of chondrocyte-derived osteogenesis.
Statistical analysis
All statistical analyses were conducted using SPSS (version 28.0; IBM, Armonk, NY). Based on the skewness and kurtosis statistics, the data were normally distributed. For quantitative analysis on the histologic level, 4 sections were used as an average for each animal. Parametric means and standard deviations were used to describe central tendency and dispersion. Between-group comparisons for condylar size were made using independent t tests. For 2D measurement, 3D μCT, IHC staining, and cell lineage tracing data, between-group comparisons were made using analysis of covariance, controlling for final mouse weight differences. The intraobserver error was assessed using intraclass correlation coefficients on repeated measures, with all analyses having an intraclass correlation >0.99.
Results
There was no statistical significance between-group differences in weight before diet modification. After 1 week, the soft-food diet mice were significantly lighter (2-3 g) than the hard-food diet mice. This difference was maintained throughout the study ( Table II ).
Week | Soft diet, g | Hard diet, g | P value |
---|---|---|---|
0 | 12.55 ± 1.12 | 11.88 ± 0.87 | 0.276 |
1 | 15.30 ± 2.00 | 17.70 ± 1.08 | 0.034 |
2 | 19.05 ± 1.22 | 21.89 ± 0.83 | 0.001 |
3 | 23.03 ± 1.58 | 25.24 ± 1.19 | 0.021 |
4 | 24.42 ± 2.23 | 27.74 ± 1.26 | 0.010 |
5 | 25.18 ± 2.31 | 28.68 ± 1.33 | 0.009 |
6 | 25.72 ± 2.36 | 29.55 ± 1.56 | 0.008 |
The total mandibular length (Co-Me) and mandibular base length (Go-Me) were significantly shorter in the soft-food diet group than in the hard-food diet group ( Table I ). The difference in ramus height (Co-GoT) approached statistical significance, favoring the hard food group. The 3D μCT results showed no significant differences in the bone quality of the condylar process between groups, although the soft-diet condylar process displayed lower bone volume, total volume, and bone volume fraction, with the difference approaching statistical significance ( Table III ). In summary, the radiologic analysis revealed shorter mandibles with reduced bone quality among mice fed softer diets.
Measurements | Units | Soft diet | Hard diet | P value |
---|---|---|---|---|
Trabecular no. | 1/mm | 10.01 ± 0.70 | 9.44 ± 0.44 | 0.082 |
Trabecular thickness | mm | 0.14 ± 0.01 | 0.14 ± 0.01 | 0.649 |
Trabecular spacing | mm | 0.07 ± 0.00 | 0.06 ± 0.01 | 0.474 |
Total volume (TV) | mm | 1.09 ± 0.18 | 1.37 ± 0.21 | 0.178 |
Bone volume (BV) | mm | 0.78 ± 0.15 | 1.03 ± 0.15 | 0.113 |
BV fraction | NA | 0.72 ± 0.03 | 0.75 ± 0.02 | 0.122 |
Mean/density of TV (apparent) | mg HA/ccm | 707.76 ± 24.18 | 729.84 ± 24.73 | 0.332 |
Mean/density of BV (material) | mg HA/ccm | 865.58 ± 18.05 | 871.31 ± 22.92 | 0.920 |
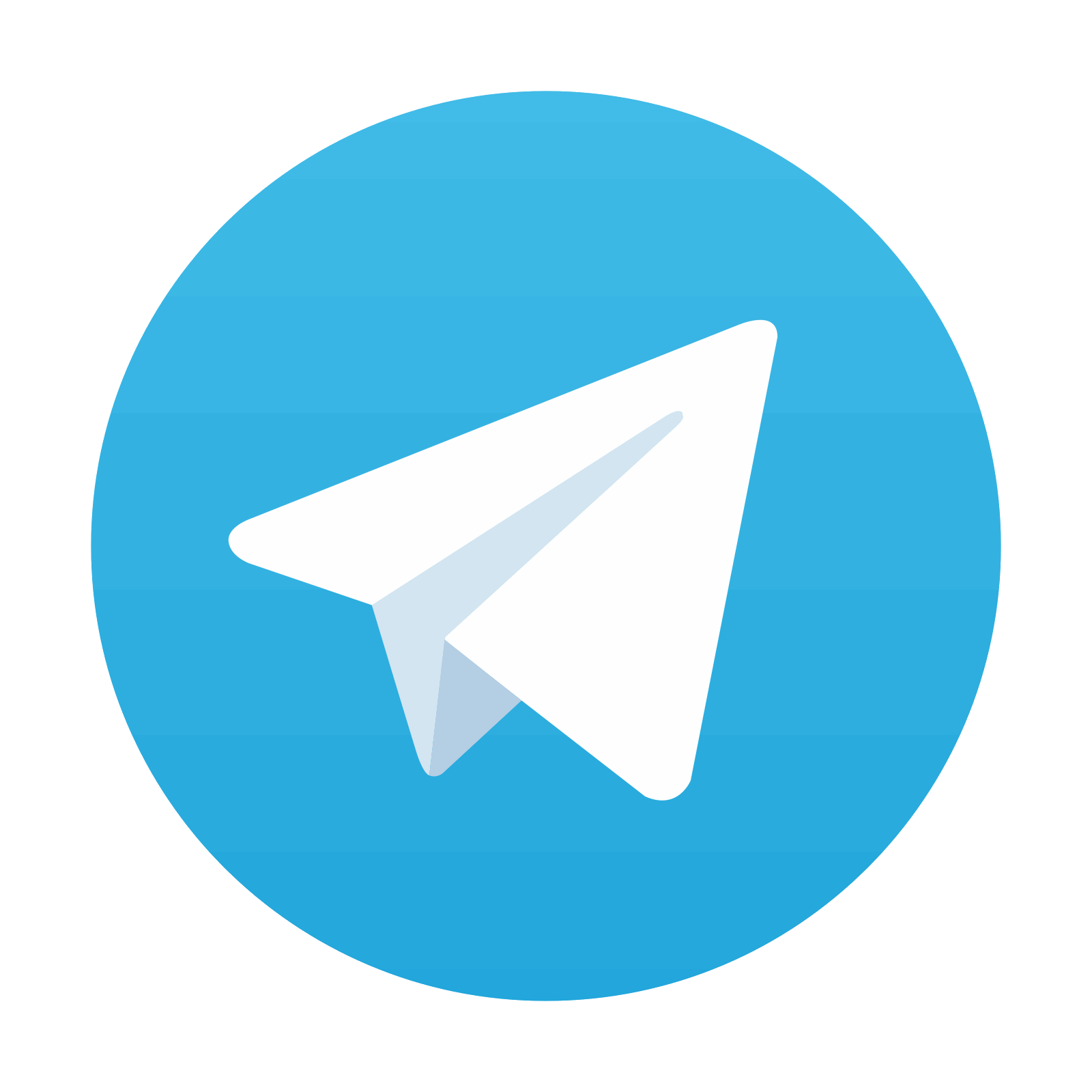
Stay updated, free articles. Join our Telegram channel

Full access? Get Clinical Tree
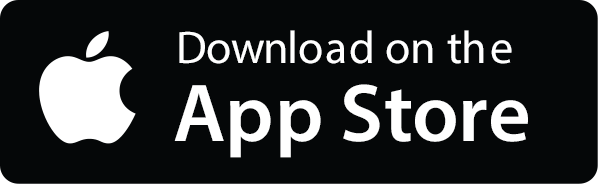
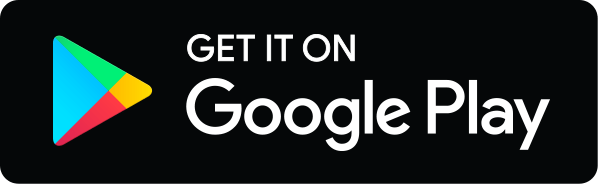
