Tendon and Ligament Healing
Kenneth M. Lin, MD
Scott A. Rodeo, MD
Dr. Rodeo or an immediate family member serves as a paid consultant to or is an employee of Flexion Therapeutics and has stock or stock options held in Ortho RTI. Neither Dr. Lin nor any immediate family member has received anything of value from or has stock or stock options held in a commercial company or institution related directly or indirectly to the subject of this chapter.
Keywords: anterior cruciate ligament; enthesis; fibrocartilage; ligament; rotator cuff; tendon
INTRODUCTION
Tendon and ligament injuries are common and lead to significant pain, disability, and work hours missed.1,2 These injuries are very diverse in terms of etiology, pathogenesis, and prognosis and affect the general population, regardless of age. The spectrum of disease includes acute traumatic injuries, sports-related injuries, and chronic tendinopathy due to repetitive overuse. Injury and tissue failure may occur at the midsubstance of ligament or tendon or at the junction to bone (enthesis). There are intra- and extra-articular ligaments and tendons, as well as extra-articular but intrasynovial tendons such as the flexor tendons of the hand. It is accepted that the microstructure and composition of tendon and ligament are not reconstituted following injury or rupture, as these tissues heal by formation of scar tissue with material properties that are inferior to native healthy tissue. Various surgical and nonsurgical treatment strategies exist, with recent interest in biologic augmentation of healing. This chapter will review basic factors related to tendon and ligament healing, using rotator cuff repair and anterior cruciate ligament (ACL) reconstruction as clinical examples of each to highlight important concepts. We will conclude by summarizing the evidence for various strategies of biological augmentation of tendon and ligament healing.
BASIC PRINCIPLES OF TENDON HEALING
BIOLOGICAL PATHWAY OF TENDON HEALING
Tendon healing generally occurs through three overlapping biological phases: inflammatory, proliferative, and remodeling.2 The inflammatory phase lasts about a week, during which vascular permeability increases and inflammatory cells (neutrophils, monocytes, lymphocytes, and macrophages) enter the injured area to remove necrotic material. A concomitant increase in the local concentration of cytokines and inflammatory mediators activates tendon fibroblasts, which begin to synthesize type III collagen.
The proliferative phase begins several days after initial injury and lasts for several weeks depending on the tendon involved. Fibroblasts proliferate and initiate new matrix synthesis, beginning with production of type III collagen. Increased proliferative activity correlates with accumulation of growth factors such as transforming growth factor-β (TGF-β), insulinlike growth factor (IGF-1), platelet-derived growth factor (PDGF), vascular endothelial growth factor (VEGF), and basic fibroblast growth factor (bFGF).3 Relative blood flow, VEGF activity, and microvessel density peak at around 1 week and remain elevated for approximately 4 weeks.4 The torn tendon hematoma becomes replaced by granulation tissue followed by immature, poorly organized collagen.
The remodeling phase follows, starting at approximately 6 weeks and lasting for many months. This phase can be divided into the consolidation and maturation stages. During consolidation, repair tissue becomes less cellular and more densely fibrous, as tenocytes and collagen fibers align based on the direction of tensile mechanical load. Collagen synthesis shifts from type III to predominantly type I.5 During
the maturation stage, cellular metabolism and overall tendon vascularity decrease concomitant with progressive matrix remodeling.
the maturation stage, cellular metabolism and overall tendon vascularity decrease concomitant with progressive matrix remodeling.
On a cellular level, there are many important mediators in tendon healing. Tenoblasts and tenocytes are present in both epitenon and endotenon, and it is also known that tendons contain a niche of intrinsic resident progenitor cells.6 Animal studies have shown that tendon healing is initiated by proliferation of cells from the epitenon, followed later by proliferation and matrix synthesis by endotenon cells.6 Intrinsic tenocytes derived from the endotenon secrete larger and more mature collagen fibers compared with epitenon cells and are thought to begin synthesizing collagen later in the repair process. Matrix metalloproteinases (MMPs) play an important role in collagen remodeling. Animal studies have demonstrated that MMP-9 and MMP-13 participate in collagen degradation, whereas MMP-2, 3, and 14 participate in both degradation and remodeling.7 Additionally, chemokines, cytokines, and growth factors, initially secreted by platelets, neutrophils, and monocytes play a role in neovascularization and migration and activation of tenocytes.
The tendon healing process relies on a complex interplay of intrinsic basic cell biologic activity (cell proliferation, matrix synthesis, and remodeling), tissue vascularity, the inflammatory response to injury, and exogenous factors (cytokines and biomechanical loads).
ANATOMIC CONSIDERATIONS IN TENDON HEALING
There are several important distinctions related to tendon and ligament healing. Tendons can be intrasynovial or extrasynovial. Intrasynovial tendons (such as the flexor tendons of the hand) have a synovial sheath which allows for decreased friction, abrasion, and greater excursion; the presence of the synovial sheath also has biological implications for healing and repair strategies. All tendon healing occurs through two general pathways: Intrinsic healing occurs through proliferation of cells in the tissue itself, such as epitenon and endotenon tenocytes, while extrinsic healing occurs via invasion of cells, generally from the surrounding sheath or synovium.8 Intrinsic tendon healing results in improved biomechanics and fewer complications, most notably better gliding mechanics, and is generally the goal of treatment; extrinsic healing tends to lead to scar formation and is the predominant mode of healing in tendon ruptures seen clinically.
Another important distinction is that between intra- and extra-articular tendons and ligaments. In general, intra-articular tendons and ligaments have poor intrinsic healing capacity and usually require surgical repair in the event of complete rupture, such as the rotator cuff and ACL. It is hypothesized that the presence of synovial fluid, matrix-degrading proteases, and other intra-articular mediators have an adverse effect on healing of intra-articular tissues. One fundamental reason for poor healing of intra-articular issues is the failure of a fibrin clot to form and serve as a provisional scaffold for healing. The presence of fibrinolysates in the injured joint prevents formation of a stable fibrin clot. Furthermore, the levels of inflammatory cytokines needed for effective healing, including PDGF, TGF-β, and FGF, may be inadequate following intra-articular ligament rupture.9 In contrast, some extra-articular tendons and ligaments, such as the Achilles tendon and the medial collateral ligament (MCL) have been shown to heal with good outcomes following nonsurgical management, although there are exceptions such as the patellar tendon, which is almost universally treated with surgical repair. In all cases, the healed tendon tissue is composed of scar tissue, with a greater content of collagen III, smaller diameter collagen fibrils, and abnormal collagen cross-links in comparison to native tendon tissue.
MIDSUBSTANCE VERSUS TENDON-BONE HEALING
Due to material, mechanical, and biological properties of bone and the bone-tendon interface, bone-to-tendon healing differs from midsubstance tendon-to-tendon healing. Direct insertions, such as the rotator cuff (Figure 1) and ACL (Figure 2) insertions to bone, have a tendon-bone interface mediated by fibrocartilage, through four zones: tendon, unmineralized fibrocartilage, mineralized fibrocartilage, and bone. In contrast to bone-to-bone healing, spontaneous healing with restoration of the microstructure and composition of the native enthesis does not typically occur at the bone-tendon junction after injury. Failure of healing may be due to numerous factors, including the relative avascularity of the fibrocartilage region, pathological changes in the bone, the complex mechanical loading environment, and gap formation between tendon and bone at the site of injury. Animal models and limited analysis of clinical specimens have shown that tendon-bone healing occurs through fibrovascular scar tissue rather than regeneration of the native fibrocartilaginous transition zone.1 Even at late time points following tendon rupture and healing, the structural and mechanical properties of a healed tendon to its bony insertion do not return to normal. In contrast, in midsubstance tendon or ligament healing, generation of well-aligned collagen fibers can lead to good return of function.
While tissue at a repaired enthesis is biologically inferior to native insertion site tissue, a further challenge to adequate healing is pathological change at the bone attachment site. Bone loss and focal reduction in bone mineral density has been reported after tendon rupture and repair. In a canine model of flexor tendon repair as well as a rat model of rotator cuff repair, decreased bone mineral density is seen at the insertion site even at late times following repair, suggesting that bone resorption may contribute to the clinically observed delay in tendon-bone healing, such as the inferior healing rates seen in rotator cuff repairs treated with early or excessive mechanical loading.5
CHRONIC TENDINOPATHY VERSUS ACUTE INJURY
The exact underlying cellular and molecular mechanism(s) that predispose to tendon injury are unknown, and thought to be multifactorial.9 Many intrinsic and extrinsic factors may predispose to tendon injury, such as age, sex, disease comorbidities (diabetes, rheumatoid arthritis), medication use, sports activity, and limb alignment.10 When categorizing tendon injuries into acute versus chronic types, acute ruptures are thought of as occurring in relatively normal tissue, although it has been demonstrated that some degree of preexisting tendinopathy is usually present for a tendon to spontaneously rupture.11 In chronic tendinopathy, however, there is accumulation of microscopic matrix damage that occurs over time, weakening the tendon and altering the innate biological healing capacity. Degeneration is hypothesized to involve hypoxia, ischemic damage, oxidative stress, nonuniform mechanical loading, and production of inflammatory cytokines.10 It has become increasingly clear that inflammatory mediators play an important role in the initiation of the pathological tendon changes. For example, it has been shown that administration of the inflammatory mediators prostaglandin E2 and IL-6 induce histologic changes consistent with tendinopathy.12
Acute injuries have a better prognosis for healing compared with chronic injuries,13 as treatment with surgical repair is relatively straightforward. In chronic injuries, there is an inferior innate biological healing capacity, resulting in the high failure rates reported in surgical repairs of ruptured tendinopathic tendons, particularly in large rotator cuff tears.14 Given these biological limitations, research efforts have focused on strategies to augment the biology of tendon healing, especially in chronic tendinopathy.3
CLINICAL ASSESSMENT OF TENDON AND LIGAMENT HEALING
In the clinical setting, objective evaluation of the quality of tendon or ligament healing is difficult. While various clinical scores and physical examination maneuvers allow assessment
of the patient’s function and the overall status of the repaired or reconstructed tendon (such as a positive or negative anterior drawer test for the ACL), there are no standardized scores for assessment of tendon healing in the postoperative patient. As second-look arthroscopy and tissue sampling is only possible in patients indicated for a subsequent procedure, direct assessment of the tissue quality of the graft is limited to MRI or ultrasonography imaging. In the setting of ACL reconstruction, oblique coronal and sagittal MRI have been used for postoperative evaluation of graft healing, and several authors have proposed scores for quantification.15,16 When assessing graft maturity of autografts and allografts at 2 years, Li et al found that allografts had a significantly higher signal to noise quotient, suggesting a lower rate of revascularization and integration.17 However, clinical outcomes were similar between both groups, and the lack of association between graft maturity on MRI with clinical scores has been reported from other studies as well,18,19 suggesting that graft maturity and incorporation assessed by current noninvasive imaging techniques should not be an objective criteria for determining timing of return to sport.
of the patient’s function and the overall status of the repaired or reconstructed tendon (such as a positive or negative anterior drawer test for the ACL), there are no standardized scores for assessment of tendon healing in the postoperative patient. As second-look arthroscopy and tissue sampling is only possible in patients indicated for a subsequent procedure, direct assessment of the tissue quality of the graft is limited to MRI or ultrasonography imaging. In the setting of ACL reconstruction, oblique coronal and sagittal MRI have been used for postoperative evaluation of graft healing, and several authors have proposed scores for quantification.15,16 When assessing graft maturity of autografts and allografts at 2 years, Li et al found that allografts had a significantly higher signal to noise quotient, suggesting a lower rate of revascularization and integration.17 However, clinical outcomes were similar between both groups, and the lack of association between graft maturity on MRI with clinical scores has been reported from other studies as well,18,19 suggesting that graft maturity and incorporation assessed by current noninvasive imaging techniques should not be an objective criteria for determining timing of return to sport.
CLINICAL EXAMPLE: ROTATOR CUFF REPAIR
Rotator cuff pathology is common, with symptomatic rotator cuff injuries affecting up to 30% of the population20; among the aging population, the prevalence of full-thickness tears is estimated to be as high as 30%.21 Depending on injury factors, activity level, functional status, and occupation, surgical repair or reconstruction is generally the treatment of choice for injuries of the rotator cuff. The rotator cuff presents special challenges for tendon healing: its tendons are intra-articular and thus intrasynovial, there is a complex mechanical loading environment, the native fibrocartilaginous insertion to the greater tuberosity is not reconstituted, and there is almost always an element of chronic tendinopathy leading to impaired biological healing capacity on both the tendon and bone side. Factors known to be associated with failure of rotator cuff repair include muscle degeneration (fatty infiltration and atrophy), tear size, chronicity, advanced age, and other environmental factors.22
TREATMENT TECHNIQUES
Treatment of rotator cuff tears begins with nonsurgical measures such as activity modification, physical therapy, nonsteroidal anti-inflammatory medications, and corticosteroid injection. Oftentimes, however, patients will require surgical management. When tears are irreparable, or the patient has concomitant advanced glenohumeral arthrosis, alternative options such as arthroplasty can be recommended. When repair is indicated, many approaches and techniques have been described, including traditional open repairs, mini-open deltoid split approaches, and arthroscopic repair. The current standard of care is arthroscopic repair, although the superiority of arthroscopic versus open or mini-open repair is still somewhat controversial. Arthroscopic repair has been shown to have similar outcomes and failure rates, with decreased short-term pain and more rapid return to activity.23
The rotator cuff tends to fail at or near the tendon-bone junction, and the goal of repair is to restore the tendon to its anatomic footprint to encourage healing.23 Historically, transosseous tunnels were used in open rotator cuff repair, with sutures placed and tied through bone tunnels from the cuff footprint to the lateral aspect of the tuberosity. Arthroscopically, repair is performed with suture anchors in various configurations—single-row, double-row, and transosseous-equivalent repair technique. Controlled laboratory studies have generally shown superiority of double-row techniques over single-row in terms of initial and ultimate failure strength, decreased gap formation, decreased strain and suture cut-through, and improved vascularity in transosseous-equivalent double-row repair.24
BIOLOGY OF ROTATOR CUFF FOOTPRINT HEALING
It is well-established that the anatomic structure of the rotator cuff insertion site is not regenerated after tendon-to-bone repair.25 Repair of the rotator cuff leads to formation of a fibrovascular scar at the healing tendon-bone interface that never attains the gross, histological, or mechanical properties of native tendon1 (Figure 3). Clinically, it has been shown that repair failure occurs at the tendon attachment site, with the tendon pulling through the sutures.26 This suggests that the tendon-bone interface is slow to heal due to the formation of scar tissue with inferior material properties at the attachment site. Biologic factors that adversely affect healing include poor vascularity, the presence of excessive inflammatory mediators and matrix metalloproteinases, and the presence of chronic tendinopathic change. Furthermore, the concentration of biomechanical load on the suture-tendon interface may further impair healing. The presence of chronic tendinopathic change is almost ubiquitous in patients requiring rotator cuff repair and is associated with poor outcomes; in a controlled laboratory study in rats, repair of rotator cuff tendon with chronic degenerative change led to inferior healing, with decreased bone mineral density at the footprint, abnormal trabecular structure, and weaker biomechanical strength at the repair site.27
Clinical and research emphasis has been placed on footprint and tendon edge preparation in an effort to understand and manipulate healing potential. Studies in rat models comparing various techniques of footprint preparation have shown that leaving the footprint untreated (and preserving what is left of native fibrocartilage) or microfracture leads to greater mean load to failure, thicker collagen bundles, and greater fibrocartilage formation than radiofrequency ablation of the footprint or excavation of the footprint down to cancellous bone.28 Overall bone health has also been hypothesized to affect footprint healing, as vitamin D deficiency has been shown to significantly decrease load to failure in a rat model of rotator cuff repair, with histological analysis showing less bone formation and inferior collagen fiber organization.29
Regarding tendon edge preparation, a clinical study using biopsy samples of cuff tissue at various distances from the
edge of the tear from patients during arthroscopic rotator cuff repair showed increased expression of proapoptotic mediators, including caspases 3, 8, and 9, BAX (Bcl-2 associated X protein), and TNF-α closer to the tendon edge compared with a more proximal biopsy site.30 Moving distal to proximal from the torn tendon edge, there was an increased expression of antiapoptotic Bcl-2 and anti-inflammatory IL-10. A separate analysis of the same specimens showed decreased expression of type I collagen and increasing expression of proinflammatory IL-1β and interferon gamma moving from proximal to distal, closer to the torn tendon edge.31 The exact role of the inflammatory response remains unclear, but it is known that inflammatory mediator expression is associated with increased expression and activity of matrix metalloproteinases, and it is hypothesized that a balance must be achieved between pro- and anti-inflammatory signals.32 In a rat model of rotator cuff repair, use of both indomethacin, a nonselective NSAID, and celecoxib, a COX-2-specific inhibitor, had negative effects on healing, with significantly lower load to failure and inferior collagen organization compared with controls in both groups.33
edge of the tear from patients during arthroscopic rotator cuff repair showed increased expression of proapoptotic mediators, including caspases 3, 8, and 9, BAX (Bcl-2 associated X protein), and TNF-α closer to the tendon edge compared with a more proximal biopsy site.30 Moving distal to proximal from the torn tendon edge, there was an increased expression of antiapoptotic Bcl-2 and anti-inflammatory IL-10. A separate analysis of the same specimens showed decreased expression of type I collagen and increasing expression of proinflammatory IL-1β and interferon gamma moving from proximal to distal, closer to the torn tendon edge.31 The exact role of the inflammatory response remains unclear, but it is known that inflammatory mediator expression is associated with increased expression and activity of matrix metalloproteinases, and it is hypothesized that a balance must be achieved between pro- and anti-inflammatory signals.32 In a rat model of rotator cuff repair, use of both indomethacin, a nonselective NSAID, and celecoxib, a COX-2-specific inhibitor, had negative effects on healing, with significantly lower load to failure and inferior collagen organization compared with controls in both groups.33
EFFECT OF BIOMECHANICAL LOAD
Mechanical loads at the healing tendon-to-bone attachment site have a critical effect on healing. Cells in both tendon and bone respond to mechanical loads, and stress shielding can lead to adverse changes in these tissues.34 However, excessive mechanical load in the early postoperative period impairs healing,35 which may be due in part to failure of resolution of the postoperative inflammatory response. Proper tendon healing requires re-establishment of collagen fiber continuity between tendon and bone, and collagen fibrils become organized and aligned in response to biomechanical load. Biomechanical load on the healing tendon attachment site also leads to enhanced cell proliferation, and selective enhanced expression of tenocyte-related genes such as tenomodulin, but not genes related to other cell lineages such as chondrocytes or osteocytes.36
Despite the physiologic role of load in tendon development and homeostasis, loads applied too early to a repaired tendon can inhibit healing. Biomechanical studies in animal models of tendon healing have shown that after repair, a period of initial immobilization results in a stronger tendon-bone complex with improved collagen organization with less scar formation than immediate loading.37 It should be noted, however, that prolonged immobilization, in both repaired and native tendons, has been shown to significantly weaken the bone-tendon complex, with a corresponding increase in MMP-13 activity.35 A certain level of biomechanical stress is required for tendon homeostasis and repair, and this is reflected in laboratory studies of tendon development: injection of botulinum toxin A at birth into the supraspinatus showed reduction in muscle volume, fibrocartilage development, and bone mineralization, showing that a baseline level of muscle loading is required for normal development, and likely healing as well.38 Beyond simply overstressing the repair mechanically, there are direct biological consequences of changing load on a repaired rotator cuff, such as activation of various MMPs. Further research is required to elucidate the cellular pathways involved in mechanotransduction.
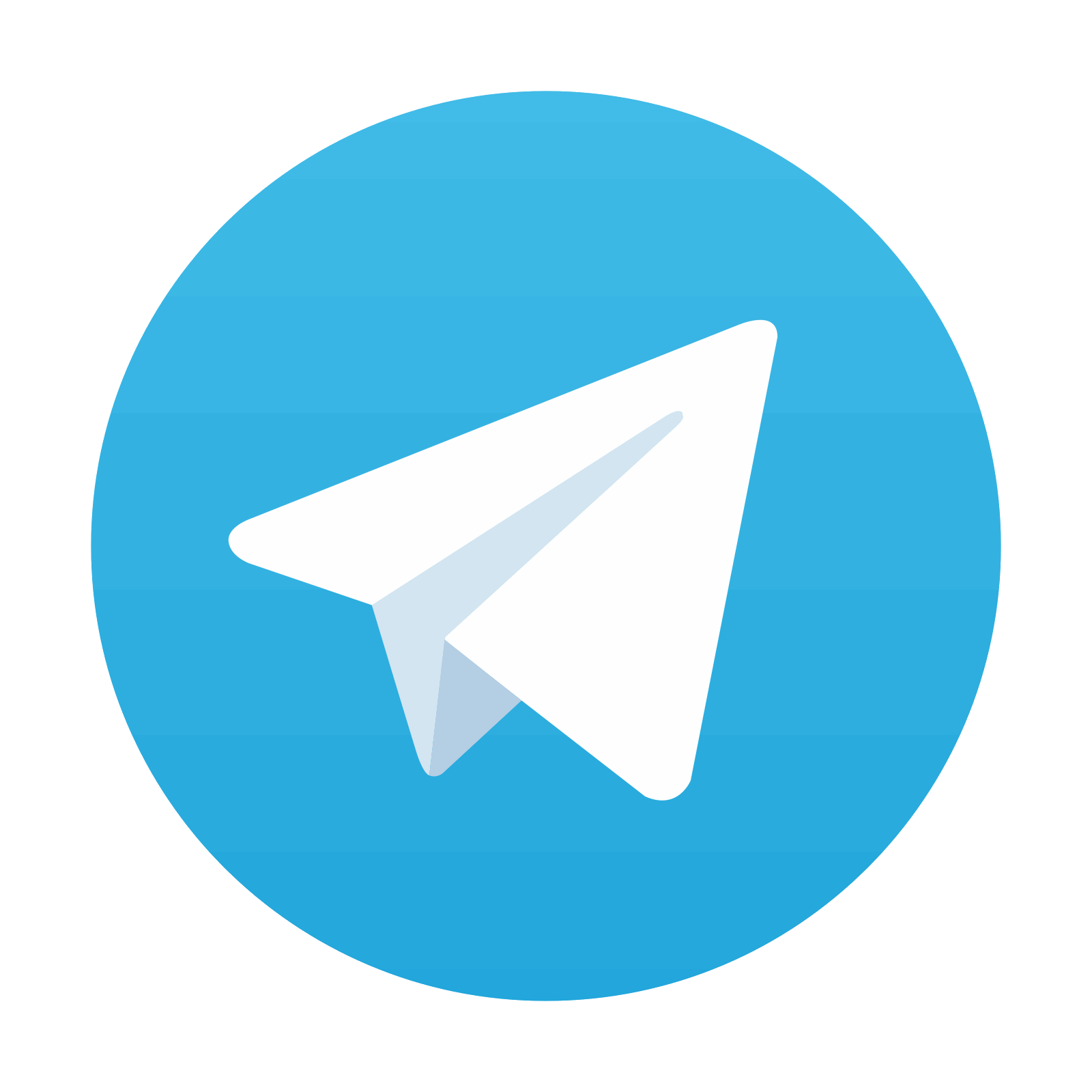
Stay updated, free articles. Join our Telegram channel

Full access? Get Clinical Tree
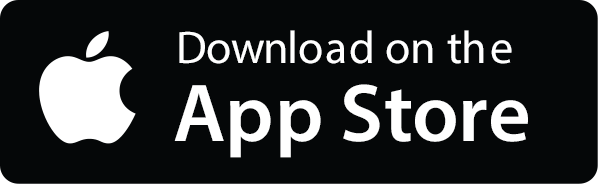
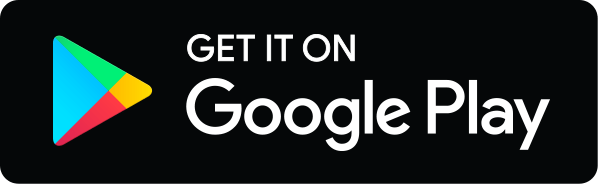
