General Principles
Definition
Stress fractures are fatigue-failure injuries of the bone affecting physically active people, including military recruits, track and field athletes, and ballet dancers. With the increased role of exercise for elderly people and patients with chronic disease, stress fractures should not be overlooked in nontraditional populations.
Etiology
- •
Stress fractures are overuse injuries that present over a continuum of fatigue failure of the bone, from microfracture to complete structural failure. Excessive repetitive stress alters the balance of bone remodeling. Bone formation (osteoblastic activity) lags behind resorption (osteoclastic activity). Stress can be compressive, tensile, or rotational.
- •
Initially, increased osteoclastic activity at a site of stress leads to a more porous, weakened cortex. Above a certain threshold, additional stress cycles lead to the development of microscopic cracks, further decreasing bone strength. Continued repetitive stress leads to microfracture propagation and coalescence, resulting in a stress fracture. With further stress, frank fracture and displacement can occur ( Fig. 59.1 ).
Figure 59.1
Etiology of Stress Fracture.
Site specificity of stress fractures in track and field athletes is determined by the anatomic stress demanded by the sport.
Epidemiology
- •
Individual sports place stress on specific anatomic sites, which are at increased risk of stress fracture. Site specificity is determined by the athletic population ( Table 59.1 ).
TABLE 59.1
Stress Fracture Sites Associated With Selected Sports
Track
Football
Basketball
Gymnastics
Softball (Fast-Pitch)
Dance
Running
Navicular
Tibia
First rib
Pars interarticularis
Rib
Metatarsals
Tibia
Tibia
Pars
Tibia
Radius
Ulna
Fibula
Fibula
Metatarsals
5th metatarsal
5th metatarsal
Midfoot
Humerus
Pars interarticularis
Metatarsals
Fibula
Tarsal
Navicular
Tibia
- •
Certain sports are more commonly associated with stress fractures: running ( 69% ), fitness class (8%), racket sports (5%), basketball (4%), and other sports (14%).
- •
Female military recruits have 10 times the fracture risk of males; female athletes have 3.5 times the fracture risk of males.
- •
Risk is activity dependent: higher risk for female runners and gymnasts.
- •
Females’ higher risk may be related to underlying menstrual irregularities and associated decreases in bone density (e.g., with female athlete triad) or anatomic and biomechanical factors specific to females.
- •
- •
The most common bones injured, reported as percentage of all stress fractures: tibia (49.1%), tarsals (25.3%), metatarsals (8.8%), femur (7.2%), fibula (6.6%), pelvis (1.6%), sesamoids (0.9%), and spine (0.6%); bilateral injuries in 16.6% of cases
- •
Age relationship: Femoral and tarsal fractures more common in older patients; tibial, fibular, and upper extremity fractures more common in younger patients
Protective and Risk Factors
See Table 59.2 .
Intrinsic Factors | Extrinsic Factors |
---|---|
Alignment abnormalities | Overt |
Femoral neck anteversion | Continued self-abuse |
Pronation/supination | Inappropriate training |
Tibial torsion | Inappropriate technique |
Leg length discrepancy | Inappropriate equipment |
Muscle imbalance | Harsh environment |
Muscle weakness | Covert |
Flexibility | Joint instability |
Genetic predisposition | Extrinsic pressure |
Aging/hormonal | Biomechanical fault |
Bone Characteristics
- •
Composition: Mineral deposition around collagen matrix; the bone resists compression; the collagen matrix (connective tissue) resists tension
- •
Bone remodels in response to stress (Wolff’s law).
- •
Allows protective increases in cortical thickness, density, and diaphyseal diameter ( Fig. 59.2 )
Figure 59.2
Bone architecture in relation to physical stress.
- •
Remodeling is a function of number, frequency, and duration of loading cycles. It is influenced by strain volume, application rate, and duration per cycle.
- •
Osteoclasts activated by stress results in increased activity over 30–45 days, resulting in increased bone porosity.
- •
Osteoblasts migrate into porous areas and build matrix. This process begins approximately 30 days after stress and culminates with new bone formation over approximately 180 days.
- •
If a weakened bone is subject to additional stress, osteoclastic activity can overwhelm osteoblastic activity, resulting in further weakened bone and microfractures. Each load cycle may propagate microfractures until symptomatic stress fracture results. Increased microfractures have been demonstrated in areas of bone porosity.
- •
Bone geometry may determine the risk.
- •
Larger and higher-density bones more resistant to fatigue fractures
- •
Long bones with increased diameter resist bending in response to load. During basic training, recruits with tibial stress fractures had significantly smaller tibial widths and cross-sectional areas than those without stress fracture.
- •
Soft Tissues (Intrinsic Factors)
- •
Good muscle strength is protective. Muscle contractions attenuate ground reaction forces developed at impact during running and jumping.
- •
Bone strain increases as muscles fatigue.
- •
Contraction of strong opposing muscle groups on a single bone may produce tension across the bone and promote stress fracture.
- •
No relationship between stress fracture and muscle size or flexibility
- •
General fitness is protective. Military recruits with higher activity levels before enlistment developed fewer stress fractures during basic training.
Endocrine
- •
Low testosterone or estrogen levels can increase the risk of stress fractures.
- •
Oligomenorrheic or amenorrheic female athletes are at a risk secondary to decreased estrogen levels and increased osteoclastic activity.
- •
High-intensity training may suppress menses.
- •
Delayed menarche, often observed in athletes, may result in decreased bone density but probably does not increase the risk of stress fractures.
- •
Cigarette smoking lowers estrogen levels in a dose-dependent manner, resulting in higher osteoclastic activity.
Nutritional
- •
Stress fractures are associated with a lower fat intake, a lower caloric intake, eating disorders, and weight <75% of the ideal body weight.
- •
Calcium intake correlates with bone density; low calcium intake does not correlate with stress fractures.
Training Intensity
- •
Training errors include rapidly escalating frequency, duration, and intensity of training; 60% of running injuries are associated with training errors.
- •
Stress fractures are increased during the initial 2 weeks after increased training intensity, with increases >30% in duration or intensity over a single season, and in freshman runners adjusting to collegiate training demands.
- •
Multiple factors compound the risk
- •
Independent of risk associated with menstrual dysfunction, athletes training >5 hours/day have a 6-fold increased risk of stress fractures.
- •
A dancer who is both amenorrheic for >6 months and training >5 hours/day is 93 times more likely to develop a stress fracture than an eumenorrheic counterpart who trains less.
- •
Extrinsic Factors
- •
Surface
- •
Hard running surfaces increase impact forces.
- •
Unidirectional running on cambered track surfaces may predispose to injury.
- •
Large epidemiologic studies reveal no association between stress injury and running surface.
- •
- •
Environment (e.g., hills or uneven terrain)
- •
Technical faults in training or performance— may predispose to stress injury
Diagnosis
Time to Diagnosis
- •
It takes an average of 5–16 weeks to diagnose a stress fracture. Delayed diagnosis can be detrimental, particularly with high-risk stress fractures.
History
- •
Maintain a high index of suspicion.
- •
Certain affected anatomic areas are particularly challenging to diagnose.
- •
Recall of specific detailed historical facts may be challenging for athletes.
- •
Recent change in activity level over the past 2 months and preseason training
- •
Recent change in equipment or playing surface
- •
Activities added to already demanding physical performance
- •
Insidious onset of pain, usually initially after activity; with fracture progression, pain moves earlier into activity and may eventually occur with minimal activity (e.g., walking) or even at rest.
- •
Vague pain in affected region, occasionally localized
- •
Pain relief with rest and/or reduced activity
- •
Prior stress fractures
- •
Females: Menstrual irregularities, recent weight changes, eating disorder history or behaviors, and nutrition
- •
Older athletes, particularly females: osteoporosis risk factors (e.g., smoking, family history, corticosteroid use, body weight, Caucasian race, menopause, and hormone replacement)
Physical Examination
- •
Point tenderness, edema, warmth, and palpable callus in certain cases ( Fig. 59.3 ); in areas where bone not easily palpated, such as femoral neck, gentle range of motion (ROM) may elicit pain
Figure 59.3
Physical examination of stress fracture.
- •
Tenderness with percussion or with sound waves (tuning fork or ultrasound)
- •
Specific tests:
- •
Stork test or one-legged standing hyperextension test: Back extension with resultant hip flexion while standing on one leg increases pain in pars interarticularis stress fractures.
- •
Fulcrum test: Performed by placing fist or arm of examiner under suspected fracture site and applying “bending” force to distal extremity while proximal extremity is kept relatively immobilized by anatomic restraints; positive test results in pain over the fracture site; commonly used to evaluate femoral shaft stress fractures but may be used in other “long bone” areas
- •
Hop test: Hopping on affected leg reproduces pain at the fracture site, usually with femoral shaft stress fractures.
- •
Tuning fork test: Vibrating tuning fork over fracture site results in pain at the fracture site; high rate of false positives
- •
Radiologic Studies
- •
Plain radiographs usually negative in the early phase, particularly during initial 2–3 weeks; two-thirds of initial radiographs are negative, but half ultimately prove positive; radiographs are specific but not sensitive.
- •
Ultrasound: Not reliable for diagnosis; several studies in progress
- •
Bone scan: Approximately 100% sensitive but not specific; not helpful for guiding return to play; uptake on bone scan normalizes over 12–18 months, often lagging behind resolution of clinical symptoms; particularly useful in tarsal, femur, pelvis, and tibial plateau fractures; helps differentiate stress fractures from periostitis (shin splints). Stress fractures are usually positive in all phases of triple-phase technetium bone scan (angiogram, blood pool, delayed). Periostitis is often negative in the angiogram and blood-pool phase and positive in the delayed image phase.
- •
Single-photon emission computed tomography (SPECT): More specific than planar bone scan; particularly helpful in detecting stress fractures of the pars interarticularis, pelvis, and femoral neck
- •
Computed tomography (CT): Delineates bone well, useful when diagnosis is difficult (e.g., tarsal navicular stress fractures); helpful with spinal or linear stress fractures
- •
Magnetic resonance imaging (MRI): Superior sensitivity and specificity over bone scan and CT for soft tissue abnormalities and edema; may delineate early injury. Findings include periosteal or marrow edema. MRI may be highly useful with certain stress fractures (such as hip and pelvis).
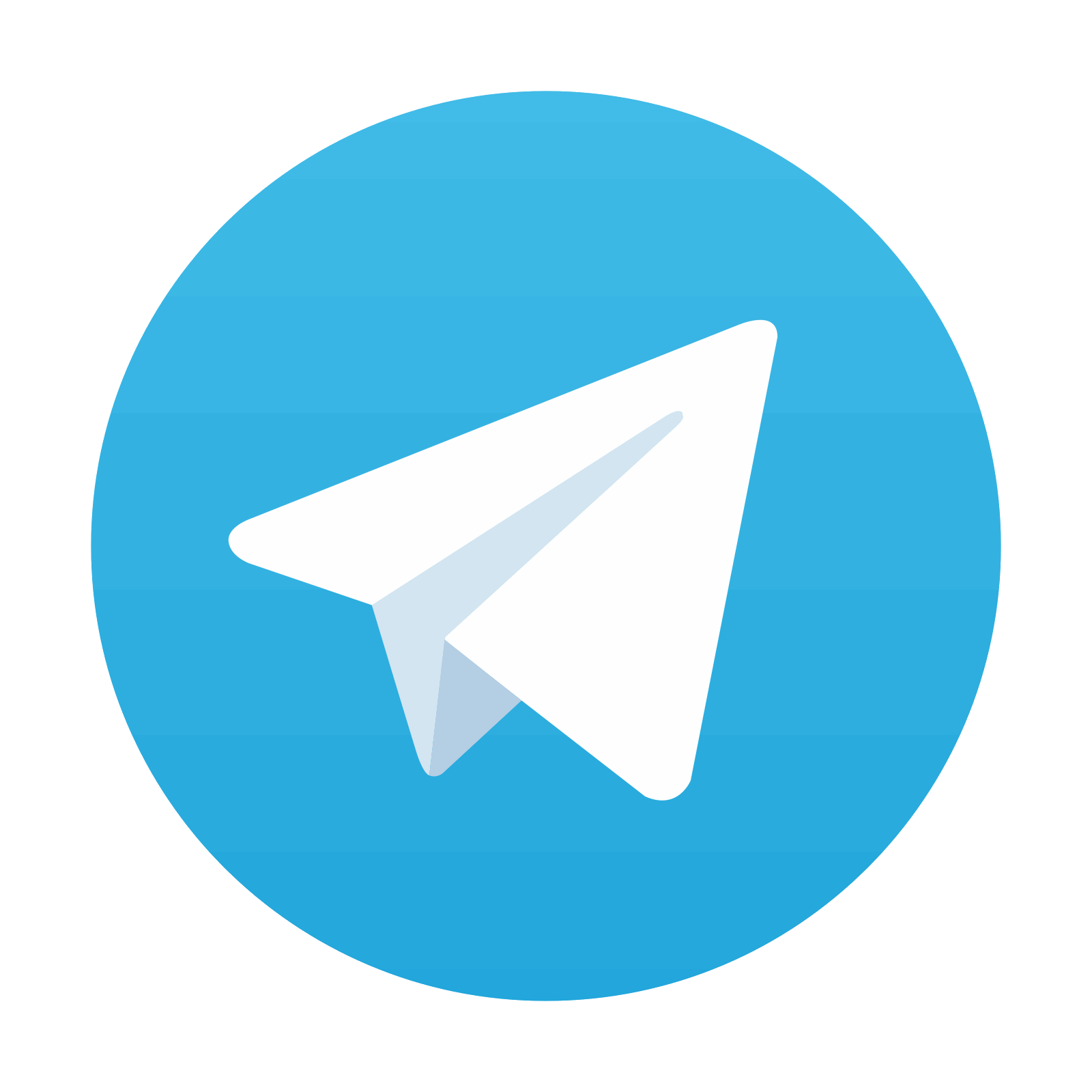
Stay updated, free articles. Join our Telegram channel

Full access? Get Clinical Tree
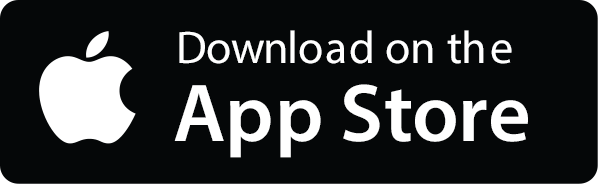
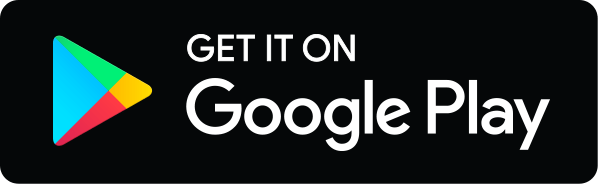