Regenerative medicine encompasses all therapeutic approaches to enhance repair processes by replacing or regenerating cells, tissues, or organs to restore health. At its core, outcomes in regenerative medicine are influenced by the genome, the patient, and the cell (Fig. 95–1). Although diverse strategies have been examined, (e.g., stem cells from diverse sources, biomaterials, tissue engineering products, and neo-organogenesis), regenerative medicine is driven by the capacity of stem cells to acquire multiple and discrete fates, a property that can be exploited to repair and/or replace damaged tissue for improved therapeutic and functional outcome.
Figure 95–1
Factors of regenerative medicine: The three-body problem of regenerative medicine. The three factors—cell, genome, and patient—influence each other in complex and sometimes unexpected ways. These three separate scientific foci of regenerative medicine must be developed in the context of one another to have meaningful impact. (Reproduced with permission from Tolar J, Osborn M, Daughters R, Banga A, Wagner J. Regenerative Medicine: Multipotential Cell Therapy for Tissue Repair. In: Kaushansky K, Lichtman MA, Prchal JT, Levi MM, Press OW, Burns LJ, Caligiuri M, eds. Williams Hematology, 9e New York, NY: McGraw-Hill;2016.)

The discovery of cells that exhibited self-renewal and potency was the first identification of a new type of cells: embryonic stem cells.1 However, the earliest conceptual understanding of the “epigenetic landscape” that is the basis for current understanding of stem cell plasticity was suggested in 1957 by CH Waddington.2 Conceptually, Waddington likened embryonic stem cells to a marble sitting at the top of a hill poised to roll down and end up in any number of valleys. From this, it was understood that multiple paths could be taken by an embryonic stem cell to acquire its final phenotype. Although some tissues retain a pool of resident, semi-differentiated stem cells for limited repair and renewal, once an embryonic stem cell is committed to a specific cellular fate, it is considered terminally differentiated and the energy required to push it back up the hill to effect a “reset” is insurmountable (Fig. 95–2).
Figure 95–2
Classic model of differentiation: Development of various formed elements of the blood from bone marrow cells. Cells below the horizontal line are found in normal peripheral blood. The principal sites of action of erythropoietin (erythro) and the various colony-stimulating factors (CSF) that stimulate the differentiation of the components are indicated. Erythro, erythropoietin; G, granulocyte; IL, interleukin; M, macrophage; SCF, stem cell factor; thrombo, thrombopoietin. (Reproduced with permission from Blood as a Circulatory Fluid & the Dynamics of Blood & Lymph Flow. In: Barrett KE, Barman SM, Boitano S, Brooks HL, eds. Ganong’s Review of Medical Physiology, 25e New York, NY: McGraw-Hill;2017.)

This initial framework to explain the concept of pluripotency was dramatically revised with the discovery of the Yamanaka factors,3 which demonstrated that the right combination of transcription factors could restore an embryonic phenotype from a fully differentiated adult fibroblast. This reprogrammed cell possesses an equivalent ability to that of embryonic counterparts, and is able to differentiate into multiple cell types.
This finding paved the way for a new era in developmental studies and disease modeling, as one could now obtain a patient cell and reprogram it to study pathological developmental from a pluripotent origin. In addition, the potential for novel and enhanced therapeutic modalities, such as autologous cellular repair, were now possible.
Several stem cell types have been used in regenerative medicine: embryonic stem cells (ESCs), induced pluripotent stem cells (iPSCs), and endogenous/adult stem cells. The former two of these are pluripotent and can acquire any cell fate given stimulation with the appropriate signaling cues. Endogenous and adult stem cells are more restricted in their potential, that is, they are considered multipotent or totipotent, and have a limited repertoire of fates compared to pluripotent cells. However, with these cell types, their final phenotypes tend to be more robust and the procedure for guiding them to their differentiated state is less complicated than it is for ESCs and iPSCs. Overall, each class of stem cell thus possesses advantages and limitations that may make them more or less suitable depending on application.
Derived from the inner cell mass of the blastocyst, these naïve cell types are self-renewing and undifferentiated, provided the proper growth factors and conditions are present. Morphologically, ESCs are small round structures that form characteristic stem cell colonies in culture (Fig. 95–3). Ultrastructurally, the most prominent features of ESCs are nuclei that can occupy as much as 95% of cellular volume (Fig. 95–3), and mitochondria that adjust their number and metabolic activity as the cell requires.4
As the nucleus accounts for the largest proportion of ESC structure and function, regulation of chromatin metabolism are heavily weighted in the ESC genome.5,6 DNA replication, recombination, and repair are over represented, as are mechanisms of modification, that is methylation and acetylation, of DNA and histones.6–9 Chromatin accessibility is essential in regulating pluripotency states of ESCs, thus nucleosome dynamics is a key determinant of the ESC ability to respond to stimulatory cues.10,11 This capacity to regulate chromatin readability and accessibility contributes to ESC criticality,12,13 a concept in which the pluripotent genome is maintained within a range of functionality that gives it the potential to form any cell type without sacrificing its capacity to self-renew in an undifferentiated form. In the transition from pluripotent to acquired cell type, these epigenomic mechanisms are tightly controlled, fully securing the committed fate of the embryonic stem cell.
Mitochondrial plasticity also plays a role in cell specialization.14,15 The most salient metabolic characteristic of ESCs is their reliance on glycolysis to meet their energetic requirements. As ESCs begin to differentiate, mitochondria fully mature in support of the underlying metabolic switch from glycolysis to oxidative phosphorylation.4 This transition from a primitive metabolic pathway ensures that the growing energetic needs of the differentiated cell are met, as intricate ultrastructural remodeling, organelle biogenesis, and functional acquisition occurs with phenotype acquisition.
The discovery of cell reprogrammability started a revolution in the stem cell field, as it became possible to recapitulate embryonic pluripotency from adult cells.3 Taking an adult fibroblast cell, Shinya Yamanaka’s group strategically re-expressed transcription factors to define the minimum essential factors required to acquire an embryonic phenotype. They found that the combination of Oct4, Sox2, Klf4, and Myc reverted fibroblasts back to an embryonic-like phenotype. These induced pluripotency stem cells (iPSCs) exhibit hallmarks of an inner cell mass-derived ESC and are capable of differentiating into non-fibroblast cell types.16 This sparked the growth of an entire field of iPSC research with critical ongoing contributions to developmental and disease modeling in the areas of neurophysiology and cardiovascular biology, for example.17–22
The iPSC phenomenon does raise interesting considerations with respect to transcriptional memory.23–26 The notion of transcriptional memory suggests that although the phenotype of iPSCs is comparable to ESCs, there are epigenetic marks that regulate key elements of reprogramming that give rise to iPSCs with varying potency. In combination with varying reprogramming efficiencies, these specificity and yield issues present significant challenges for clinical applications beyond disease modeling to investigate patient-relevant pathological development.27 While demonstration of robust ESC equivalency will be key to translational iPSC utility,28,29 current implementation of human iPSCs as patient surrogates for pharmacological testing and cellular toxicity assays provides immediate demonstration of advantages in using iPSC technology.30–32
The innate capacity for tissue repair is mediated by endogenous pools of totipotent stem cells that exhibit streamlined capacities to serve as progenitors for a limited subset of cell types. For example, hematopoietic stem cells and satellite cells in muscle are recognizable as the historically most characterized endogenous stem cell types,33–35 and it is proposed that stem cell niches exist for each tissue in the body (Fig. 95–4).36–38
Figure 95–4
Progenitor cells: In rapidly growing adult tissues and perhaps in other tissues, there are slowly dividing populations of stem cells. Stem cells divide asymmetrically, producing one cell that remains as a stem cell and another that becomes committed to a differentiative pathway but divides a few more times at a more rapid rate. Such cells have been termed progenitor cells, or “transit amplifying cells,” each of which eventually stops dividing and becomes fully differentiated. (Reproduced with permission from The Nucleus. In: Mescher AL, eds. Junqueira’s Basic Histology, 14e New York, NY: McGraw-Hill;2016.)

Tissue specificities of these potential stem cell pools are limited to fewer cell fates owing to epigenomic variability between adult and embryonic stem cells. This is supported by distinct expression differences in key methyltransferases, demethylases, acetyltransferases, and deacetylases that regulate the methylation and acetylation processes on DNA and histones.39,40 Altered DNA silencing and chromatin accessibility restrict programmatic elements that can be activated in endogenous/adult stem cells, focusing their potential to a subset of phenotypes and reinforcing the notion of transcriptional memory.
Mesenchymal stem cells (MSCs) have emerged as one of the most promising types of endogenous/adult stem cells due to a favorable ethical profile and better safety. They possess self-renewal ability and can differentiate into cells of multiple lineages, including mesoderm lineages, such as chondrocytes, osteocytes, and adipocytes, and ectodermic and endodermic cells. MSCs can be isolated from the bone marrow, adipose tissue, and umbilical cord, and are easily expanded in vitro. Experimental and early clinical data suggest that (MSCs) may be more effective than other cell types for diseases that are difficult to treat or untreatable (Fig. 95–5).
Figure 95–5
Mesenchymal stem cell differentiation into adipocytes: Mesenchymal stem cells differentiate as progenitor cells for all types of connective tissue, including preadipocytes. These are initially of at least two types. Preadipocytes developing within the lateral mesoderm of the embryo produce large number of white adipocytes (forming white adipose tissue) and a smaller number of so-called beige adipocytes with cytological features and gene expression patterns of both white and brown adipocytes. White adipocytes are unilocular, with one large lipid droplet occupying most of the cytoplasm. The white adipocyte is usually much larger than that shown here in relation to the other cell types. (Reproduced with permission from Adipose Tissue. In: Mescher AL, eds. Junqueira’s Basic Histology: Text and Atlas, 15e New York, NY: McGraw-Hill;2018.)

Brown adipocytes differentiate from another population of preadipocytes located in paraxial embryonic mesoderm and remain multilocular (having many small lipid droplets) with numerous mitochondria (not shown here). Mitochondrial metabolism of lipid in brown adipocytes releases heat rather than ATP. Cells functioning as brown adipocytes can also develop from beige adipocytes during adaptation to cold temperatures.
In the context of tissue repair and rehabilitation, translational applications can exploit the unique properties of the various stem cell types for multiple physiological systems. Based on data available in the clinical trials registry website from the National Institutes of Health (https://clinicaltrials.gov), at the time of this writing, there are around 1,000 active clinical trials associated with stem cell-based therapies in Phases 1 to 3. The majority of these clinical studies are based on endogenous/adult stem cells, with a number of therapy trials associated with active cardiovascular and neurological studies (Table 95–1). Notably, a majority of these trials utilize mesenchymal and hematopoietic stem cells. A few open trials are associated with pluripotent-based treatments, specifically human ESC (hESC). Of these, seven are associated with macular degeneration, one for Parkinson’s disease, and one for ischemic heart disease (Table 95–2). Transplantation of MSCs in animal models with spinal cord injury, have shown to promote repair, therefore, there is much interest in the clinical use of these cells in this condition as well.
NCT Number | Disease/Conditions | Cell Type | Country | Trial Phase | Registry Date |
Cardiovascular | |||||
NCT01337011 | Heart failure | Autologous bone marrow-derived SCs | Germany | Phase 1, 2 | April 14, 2011 |
NCT01569178 | Myocardial infarction | Bone marrow-derived mononuclear cells | England | Phase 3 | March 30, 2012 |
NCT01652209 | Acute myocardial infarction | Autologous bone marrow-derived MSCs | South Korea | Phase 3 | July 25, 2012 |
NCT01957826 | Primary idiopathic dilated cardiomyopathy | Autologous bone marrow-derived MSCs | Spain | Phase 1, 2 | December 21, 2012 |
NCT01758406 | Heart failure | Autologous cardiac SCs | Iran | Phase 2 | December 24, 2012 |
NCT02032004 | Chronic heart failure | Allogeneic mesenchymal precursor cells | United States | Phase 3 | January 8, 2014 |
NCT02033278 | Idiopathic dilated cardiomyopathy | Autologous mononuclear bone marrow-derived SCs | Spain | Phase 2, 3 | January 9, 2014 |
NCT02248532 | Dilated cardiomyopathy; heart failure | CD34+ bone marrow-derived SCs | Slovenia | Phase 2, 3 | September 16, 2014 |
NCT02460770 | Chronic myocardial ischemia | Autologous bone marrow-derived MSCs | France | Phase 1 | January 22, 2015 |
NCT02408432 | Cardiomyopathy | Allogenic bone marrow-derived MSCs | United States | Phase 1 | March 31, 2015 |
NCT02503280 | Chronic ischemic left ventricular dysfunction | Autologous bone marrow-derived MSCs | United States | Phase 1, 2 | May 4, 2015 |
NCT02462330 | Chronic myocardial ischemia | Autologous bone marrow-derived MSCs | France | Phase 2 | May 27, 2015 |
NCT02504437 | Myocardial infarction | Autologous bone marrow-derived MSCs | China | Phase 1, 2 | July 14, 2015 |
NCT02501811 | Ischemic cardiomyopathy | Autologous bone marrow-derived MSCs | United States | Phase 2 | July 15, 2015 |
NCT03092284 | Heart failure | Allogenic adipose-derived SCs | Denmark | Phase 2 | July 20, 2015 |
NCT02509156 | Cardiomyopathy due to anthracyclines | Allogenic bone marrow-derived MSCs | United States | Phase 1 | July 23, 2015 |
NCT02673164 | Heart failure | Allogenic adipose-derived SCs | Denmark | Phase 2 | February 1, 2016 |
NCT02781922 | Hypoplastic left heart syndrome | Autologous cardiac SCs (JRM-001) | Japan | Phase 3 | May 19, 2016 |
NCT03043742 | Chronic ischemic heart disease | CD133+ autologous bone marrow-derived SCs | United States | Phase 1 | June 17, 2016 |
NCT02962661 | Other forms of heart disease | Allogenic bone marrow-derived MSCs | United States | Phase 1 | November 8, 2016 |
NCT03047772 | Myocardial infarction | Autologous bone marrow-derived MSCs | China | Phase 2 | February 7, 2017 |
Neurology | |||||
NCT00716066 | Autoimmune neurological disease | Autologous hematopoietic SCs | United States | Phase 2 | July 15, 2008 |
NCT01896102 | Cerebral adrenoleukodystrophy (CALD) | Hematopoietic SCs | United States | Phase 2, 3 | March 22, 2013 |
NCT02245776 | Incurable neurological disorders | Autologous bone marrow mononuclear cell | India | Phase 1 | September 12, 2014 |
NCT02241395 | Cerebral palsy | Autologous bone marrow mononuclear cell | India | Phase 1 | September 12, 2014 |
NCT02338271 | Low back pain | Autologous adipose-derived MSCs | South Korea | Phase 1 | January 7, 2015 |
NCT02559830 | Metachromatic leukodystrophy | Autologous hematopoietic SCs | China | Phase 1, 2 | August 12, 2015 |
NCT02540655 | Cerebellar ataxia | Allogenic adipose-derived SCs | Taiwan | Phase 2 | August 26, 2015 |
NCT02574923 | Cerebral palsy | Autologous bone marrow mononuclear cell | Vietnam | Phase 2 | October 9 2015 |
NCT02855112 | Infantile spinal muscular atrophy, Type I | Allogenic adipose-derived MSCs | Iran | Phase 1, 2 | July 22, 2016 |
NCT Number | Disease/Conditions | Cell Type | Country | Trial Phase | Registry Date |
NCT02057900 | Ischemic heart disease | hESC-derived CD15+ Isl-1+ progenitors | France | Phase 1 | September 17, 2013 |
NCT02286089 | Age-related macular degeneration | hESC-derived retinal pigment epithelial cells | Israel | Phase 1, 2 | November 2, 2014 |
NCT02590692 | Dry macular degeneration; geographic atrophy | hESC-derived retinal pigment epithelial cells | United States | Phase 1, 2 | October 23, 2015 |
NCT02749734 | Macular degeneration | hESC-derived retinal pigment epithelium | China | Phase 1 | January 7, 2016 |
NCT02903576 | Age-related macular degeneration | hESC-derived retinal pigment epithelial cells | Brazil | Phase 1, 2 | March 7, 2016 |
NCT02755428 | Dry age-related macular degeneration | hESC-derived retinal pigment epithelium | China | Early Phase 1 | March 26, 2016 |
NCT03046407 | Dry age-related macular degeneration | hESC-derived retinal pigment epithelium | China | Early Phase 1 | February 2, 2017 |
NCT03119636 | Parkinson’s disease | hESC-derived neural precursor cells | China | Phase 1, 2 | April 6, 2017 |
NCT03167203 | Macular degenerative disease | hESC-derived retinal pigment epithelial cells | United States | Phase 1, 2 | May 24, 2017 |
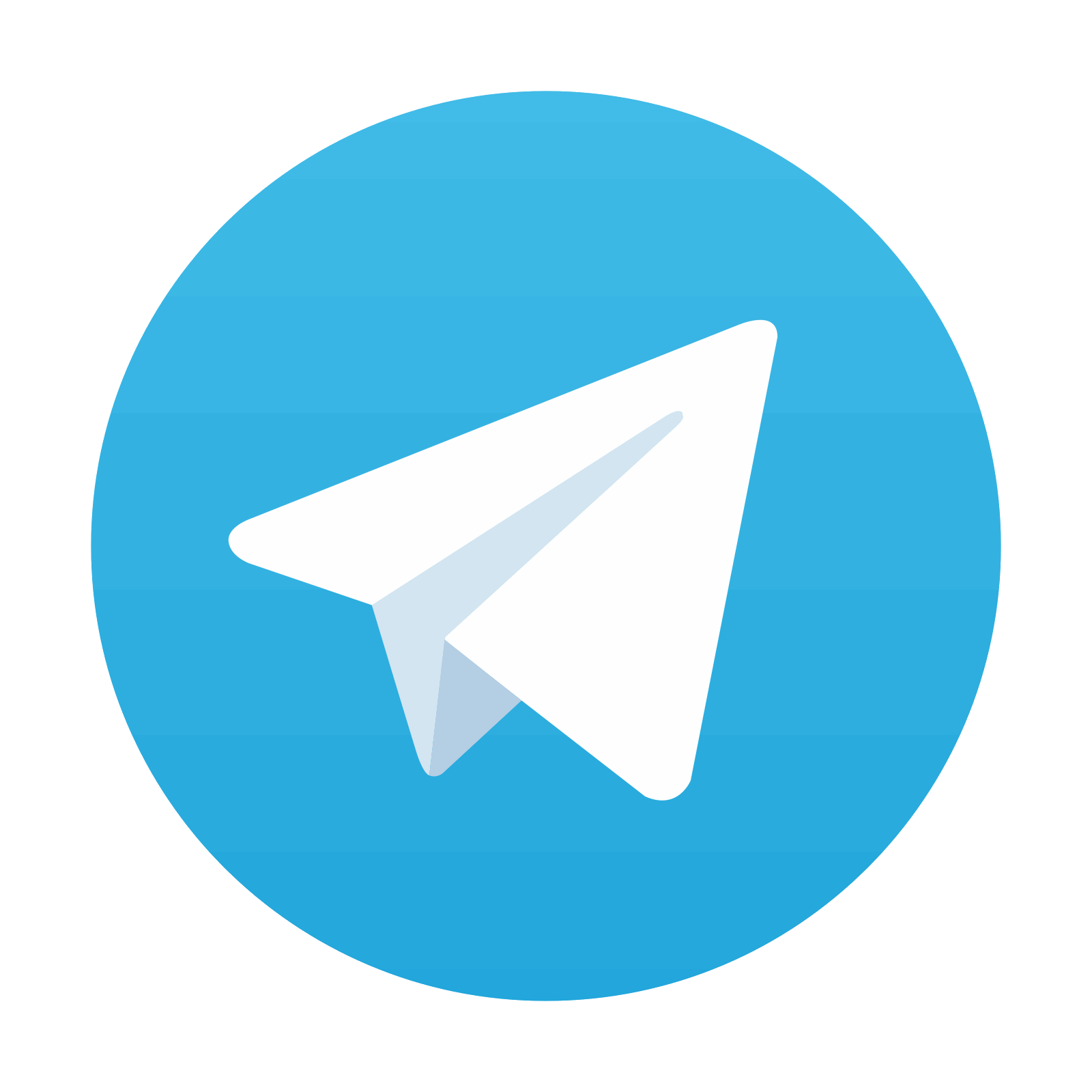
Stay updated, free articles. Join our Telegram channel

Full access? Get Clinical Tree
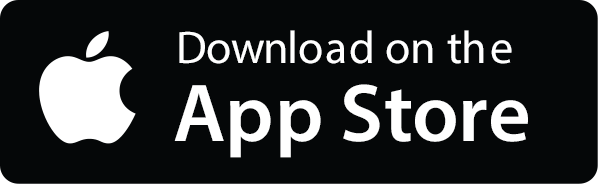
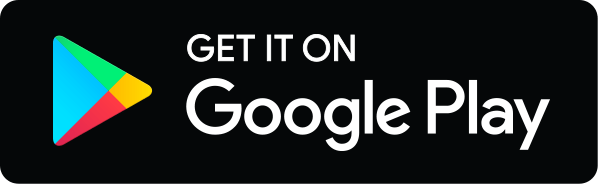
