“Although there is ample evidence that beneficial results can be obtained from the use of mesenchymal stem cells, several questions regarding their use remain to be answered. For many of these questions, preclinical models will be helpful, but the task of evaluating and implementing these findings for orthopaedic patients falls onto the shoulders of clinical researchers. Evaluation of these questions is a daunting, but such a challenge fits the concept of personalized medicine in today’s medicine.”
Key points
- •
Mesenchymal stem cells (MSCs) isolated from different tissues share many characteristics, such as the capability for multilineage differentiation, absence of HLA-DR expression, and possession of the markers CD105, CD73, and CD90, but may vary in expression of other markers, such as CD36 and CD106.
- •
In the United States, unpurified stem cells from bone marrow and other tissues can meet minimally manipulated classification as 361 tissue with exemption from Food and Drug Administration premarket review and regulation; however, enzymatic harvesting and culture expansion of MSCs are exclusive of 361 classification.
- •
Harvesting of MSCs from different anatomic sites yields varying cell numbers and proliferation rates, as exemplified by comparison of MSCs isolated from bone marrow and adipose tissues. Age and sex differences were observed with decreased proliferation and differentiation and increased senescence markers.
- •
Paracrine effects of soluble mediators and cell-to-cell interactions of MSCs affect innate and adaptive immunity and decrease inflammation.
Introduction
Over the past 60 years, evidence has accumulated supporting the existence of a multipotent adult stem cell population in the body that has the potential to differentiate into bone, cartilage, tendon, ligament, adipocytes, dermis, muscle, and connective tissue. These cells are now collectively grouped under the term mesenchymal stem cells (MSCs) or multipotent mesenchymal stromal cells. A large proportion of the studies on MSCs have involved the role of these cells in the development and repair of bone and cartilage, heightening interest in the clinical orthopaedic community. In 1966, intraperitoneal diffusion chambers implanted with mouse bone marrow cells demonstrated that undifferentiated “stem” cells were present and resulted in osteogenic foci of cells producing alkaline phosphatase (AlkP) and fibroblasts while hematopoietic cells were lost. Interestingly, orthopaedic surgeons were already using viable cancellous bone chips containing these cells in fracture repair. In the first edition of Campbell’s Operative Orthopaedics (1939), a recommended treatment of nonunions included a combination of stable fixation and packing of cancellous bone chips from the proximal tibia. Even though the concept of stem cells as we know today was unknown at the time, early orthopaedic surgeons recognized the osteogenic effect of cancellous bone and bone marrow.
Today it is clear that stem cell supplementation offers a valuable tool for correcting some of the clinical challenges in treatment of musculoskeletal diseases and injury. Between 2006 and 2012, there was a threefold increase in the number of MSC product Investigational New Drug (IND) submissions to the Food and Drug Administration (FDA), resulting in clinical trials initiated worldwide (246 trials; source: http://www.clinicaltrials.gov ). Although much of the initial research focused on bone marrow–derived MSCs (bmMSCs) with umbilical or placental sources serving as secondary sources, an increasing trend of adipose-derived MSC-based product INDs has occurred since 2011. Many of these new INDs deal with MSCs destined for allogeneic use, with more than 80% using cryopreservation for storage of the MSC products to facilitate transport to the clinical site where they are used. Cell banking of cultured MSCs (35%) in these endeavors has also been denoted; however, one report showed reduced immunomodulatory function of thawed cryopreserved MSCs immediately after thawing that was recovered after subsequent in vitro culture. The bioactivity of these products in the IND is variably described with molecular markers such as “secreted factors or expression of proteins on the surface of either the MSC or target cells (eg, T cells) that may be related to a given biological activity.”
Introduction
Over the past 60 years, evidence has accumulated supporting the existence of a multipotent adult stem cell population in the body that has the potential to differentiate into bone, cartilage, tendon, ligament, adipocytes, dermis, muscle, and connective tissue. These cells are now collectively grouped under the term mesenchymal stem cells (MSCs) or multipotent mesenchymal stromal cells. A large proportion of the studies on MSCs have involved the role of these cells in the development and repair of bone and cartilage, heightening interest in the clinical orthopaedic community. In 1966, intraperitoneal diffusion chambers implanted with mouse bone marrow cells demonstrated that undifferentiated “stem” cells were present and resulted in osteogenic foci of cells producing alkaline phosphatase (AlkP) and fibroblasts while hematopoietic cells were lost. Interestingly, orthopaedic surgeons were already using viable cancellous bone chips containing these cells in fracture repair. In the first edition of Campbell’s Operative Orthopaedics (1939), a recommended treatment of nonunions included a combination of stable fixation and packing of cancellous bone chips from the proximal tibia. Even though the concept of stem cells as we know today was unknown at the time, early orthopaedic surgeons recognized the osteogenic effect of cancellous bone and bone marrow.
Today it is clear that stem cell supplementation offers a valuable tool for correcting some of the clinical challenges in treatment of musculoskeletal diseases and injury. Between 2006 and 2012, there was a threefold increase in the number of MSC product Investigational New Drug (IND) submissions to the Food and Drug Administration (FDA), resulting in clinical trials initiated worldwide (246 trials; source: http://www.clinicaltrials.gov ). Although much of the initial research focused on bone marrow–derived MSCs (bmMSCs) with umbilical or placental sources serving as secondary sources, an increasing trend of adipose-derived MSC-based product INDs has occurred since 2011. Many of these new INDs deal with MSCs destined for allogeneic use, with more than 80% using cryopreservation for storage of the MSC products to facilitate transport to the clinical site where they are used. Cell banking of cultured MSCs (35%) in these endeavors has also been denoted; however, one report showed reduced immunomodulatory function of thawed cryopreserved MSCs immediately after thawing that was recovered after subsequent in vitro culture. The bioactivity of these products in the IND is variably described with molecular markers such as “secreted factors or expression of proteins on the surface of either the MSC or target cells (eg, T cells) that may be related to a given biological activity.”
Mesenchymal stem cell definition
What is the definition of this stem cell population and what are the differences between stem cell populations isolated from different tissues (bone marrow, adipose tissues, cord blood, muscle, synovium, dental pulp, muscle, and others)? Caplan has stated that “All or most MSC arise in vivo from perivascular cells (pericytes) that are released from the damaged or inflamed blood vessels at the site of injury.” If this is so, then tissues that have a poor vascular supply would heal poorly or not at all and this is supported by clinical observations of the mid to inner portions of the meniscal and articular cartilages. However, healing by fibroblast proliferation and scar formation and the regrowth of differentiated cells originating from stem cells that are specific to the function of the damage tissue are separate issues. Are stem cells from different tissues equivalent?
Review of the published literature on this question gives evidence to support shared characteristics of MSCs from different tissues, but also supports that variations are present as well. Perivascular MSCs from both bone marrow (BM) and dental pulp (DP) tissues localized immunohistochemically or isolated by immunoselection document that these cells do show expression of STRO1 an early marker of stem cells and CD146 an endothelial marker, but that a 3G5 antigen marker of pericytes was predominantly in the DP population and only in a small portion of the BM cells. Another report observed that the MSC population derived from veins, artery, perivascular cells, or fibroblasts showed similarity of cell morphology and phenotypes established with 22 markers with heterogeneous expression of genes related to angiogenesis. According to criteria set up by the Mesenchymal and Tissue Stem Cell Committee of the International Society for Cellular Therapy, MSCs are adherent cells that have the capacity to differentiate in vitro into osteoblasts, chondrocytes, and adipocytes and must express CD105, CD73, and CD90 and lack expression of CD45, CD34, CD14, CD11b, CD79α, CD19, and HLA-DR surface molecules. A joint statement of the International Federation for Adipose Therapeutics and Science and the International Society for Cellular Therapy, identified CD90 + , CD73 + , CD105 + , and CD44 + as adipose tissue stem cell markers with absence of CD45 − and CD31 − . The adipose-derived MSCs are different from BM MSCs in being positive for CD36 and negative for CD106.
Food and Drug Administration regulatory guidelines
The harvesting of BM or adipose tissue from the patient for autologous transplantation results in a heterogeneous mixture of cells including the desired stem cell population. Differential centrifugation and erythrocyte lysis accomplish some concentration of the stem cells, but this population still includes hematopoietic stem cells, endothelial cells, erythrocytes, fibroblasts, lymphocytes, monocyte/macrophages, and pericytes, among other nucleated cells. These relatively unpurified cells from BM can meet in many cases the “minimally manipulated” classification as 361 tissue with exemption from premarket review and regulation by US FDA regulations. For adipose tissue, this stromal vascular fraction has been estimated to contain 15% to 30% stromal cells and 3% to 5% pericytes, with hematopoietic-related and endothelial-related cells making up 20% to 45% and 10% to 20%, respectively, of the remainder. However, enzymatic harvesting or mechanical release of the cells from adipose tissue currently disqualifies them from the 361 classification. Expansion of the adherent stem cells in culture in vitro allows another purification step and also permits differentiation of the cells, but also may be considered disqualification from 361 status dependent on culture conditions.
Harvesting and characterization of mesenchymal stem cells from different tissues
Review of the literature for the use of human MSCs identified 4 major harvest sites from placental, adipose, BM, and umbilical cord tissues. Although other tissues have been used, reports of these tissues are not as common. Comparisons of the yield of MSCs from the major sites were difficult due to the large variations among different techniques, but adipose tissue consistently yielded higher levels of MSCs, ranging from 4.7 × 10 3 to 1.5 × 10 6 cells/mL of tissue compared with BM ranges of 1 to 30 cells/mL to 3.2 × 10 5 cells/mL and umbilical cord tissues yielding 1.0 × 10 4 to 4.7 × 10 6 . The number of MSCs from placental tissues and synovium ranged from 1.0 to 30.0 × 10 3 cells per milliliter. Quantitation of the connective-tissue progenitor yield was conducted in the primary tissue isolate principally by limited dilution fibroblast colony-forming unit (CFU) assay and enumeration of the colonies (ranging from 20–50 cells per colony) forming in vitro. This allows comparison from various studies or of the yields from different tissues, but does not evaluate the capability of these cells to undergo other types of differentiation. Some investigations have reported the findings with respect to the number of phenotypic CFUs when cultured under conditions whereby multilineage differentiation occurs. Information for selective differentiation of cultures is reported as Alizarin Red staining CFUs for osteogenesis, Alcian Blue–positive staining CFUs for chondrogenesis or Oil Red O–staining CFUs for adipogenesis ( Fig. 1 ). These numbers are more relevant to the MSC population than the nucleated cell counts of the primary cells isolated as they make up such a minority of the total cells. It has been estimated that there is a mean of 55 osteoblastic progenitors per 10 6 nucleated cells from the BM, with a cautionary note for risk of variation due to blood contamination.
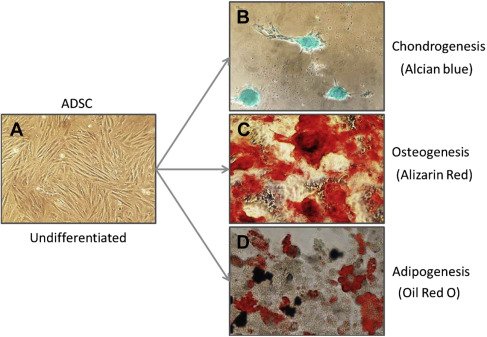
Not only did MSCs vary dependent on the tissue source, the anatomic location was important. The MSCs from subcutaneous fat proliferate in vitro at a higher rate and differentiate better than those from visceral fat. Human MSCs from different adipose anatomic sites showed that the yield was site dependent with higher frequencies of MSCs from the abdomen than the hip or thigh. In this study, abdominal adipose tissue was determined to contain 2.6 to 10.2 × 10 6 MSCs from 100 g of adipose tissue. The anatomic site of BM harvesting also associated with significant variation with up to twofold difference in the yield. Pierini and colleagues analyzed MSC yield from the posterior or anterior iliac crest and the subchondral knee sites and concluded that the posterior iliac crest was preferable, although no differences were found for viability, phenotype, expansion kinetics, or multilineage differentiation. Hernigou and colleagues studied the risk of complications when harvesting from the iliac crest and determined that higher risks, such as trocar contact with the external iliac artery, were observed with aspiration in the 4 most anterior sections with a higher risk in women. Placement of the trocar deeper than 6 cm in the posterior iliac crest was at risk for sciatic nerve and gluteal vessel damage.
Pittenger and colleagues showed only 0.001% to 0.01% of the cells in the iliac crest aspirate of normal adults exhibited the capacity for CFUs in vitro. This low number mandates larger collection volumes or culture expansion. Although the iliac crest has been the traditional site of harvest for BM, the intramedullary cavities of the long bone offers another resource, with high numbers of stem cells present in the BM. The number of stem cells that can be obtained has been enormously increased by the adaptation of a Reamer-Irrigator-Aspirator (RIA) (Synthes, Westchester, PA), consisting of a cutting head on a drive shaft with an attached irrigating and aspirating system. Comparisons were made for MSCs harvested using a traditional 10-mL iliac crest BM aspiration (ICBMA) and MSCs collected during the reaming of the femur to remove 1.5 mm at the isthmus. The material obtained during the reaming was divided into liquid (RIA liquid) and that which was strained out of the liquid through a cell strainer (RIA solid) and further digested with collagenase. Manual white cell count and in vitro culture of the cells under conditions of differentiation showed that colony formation was equivalent in ICBMA and RIA liquid fractions, although the volume of the RIA liquid was 70 times that of the ICBMA. The RIA solid had almost 4 times more colonies than the ICBMA sample and had approximately 2.5 times the volume. The ability to collect such high amounts of these stem cells suggests that this methodology could provide 50,000 MSCs in a BM concentrate, a number that has been effective in nonunions, without culture expansion.
Effects of age and gender on mesenchymal stem cells
Other variables to be considered for autologous stem cell supplementation concern the age and gender of the patient. What is the impact of age on stem cell number in an individual? A decrease in stem cell number with aging could contribute to delayed or incomplete repair in older individuals and would be a factor for consideration of supplementing with allogeneic versus autologous MSC. The data are somewhat controversial for an age-related change in the number of MSCs in BM, but the bulk of the evidence supports that aging decreases MSC numbers in the BM and increases markers of senescence. In studies of healthy volunteers, both the number and the proliferative capacity of bmMSCs from aged donors (>40 years) decreased compared with young or adult individuals. Cells from older individuals showed increased levels of radical oxygen species and senescence markers, such as p53 and p21, while AlkP-positive CFUs were reduced. These data agree with a similar study in which comparisons of MSCs harvested from young (18 years) and older (59–75 years) donors showed that the number of AlkP-positive colonies were decreased in older individuals as well as their proliferation. The decreased proliferation of the older cells was associated with a shorter telomere restriction fragment. Telomere length of a cell positively correlates with replicative ability.
A study of MSCs (STRO-1 + cells) taken from BM from discarded femoral tissues from 57 subjects, ages ranging from 17 to 90 years old, undergoing hip arthroplasty for osteoarthritis (OA) showed that the number of STRO-1 + cells in the marrow did not change with age. However, significant age-associated effects were observed, such as delayed proliferation due to prolongation of the duration of cell cycling, increased apoptosis, a fourfold greater senescence-associated β-galactosidase (SA-β-gal) in the group 55 years or older, and decreased osteoblast differentiation in terms of alkaline phosphatase activity and in the number of AlkP-positive cells. The levels of p53, a negative regulator of osteoblast differentiation, showed an age-related increase in p53. In contrast, an earlier study of BM aspirates in orthopedic patients ranging in age from 13 to 83 years showed a significant decline of nucleated cells with age, but only the female patients showed a significant decline in AlkP-positive CFUs with age, possibly relevant to osteoporosis in older women.
Tissue-dependent effects were obtained comparing the MSCs of old and young rabbits. The bone marrow–derived stem cells from old rabbits showed reduced proliferation and chondrogenic response whereas muscle-derived stem and adipose-derived stem cells exhibited no negative effects although the yield from all tissues were lower in the older animals. Although bmMSCs show detrimental changes with aging, this may not be the case for adipose-derived MSCs. The number of MSCs derived from adipose tissue or their differentiation capacity did not correlate with age of the donor in several studies, although the androgenic status of the pre-adipocytes at different locations influences the proliferation and differentiation of these cells. Another factor that has been shown to affect the stem cell population is previous treatment with steroids. Corticosteroid treatment results in decreased stem cells in the BM of the iliac crest.
Mode of action of mesenchymal stem cell supplementation
Although much of the initial enthusiasm for the use of MSCs pertained to their function as a precursor population for a more differentiated phenotype and their plasticity and multipotency in giving rise to different reparative cell types, it is apparent that these cells can also function to impact local resident cells and to decrease the immune response of both innate and adaptive immune cells and reduce inflammation. The MSCs that are present in an initial injury inhibit the immune system early in the reparative process possibly as an attempt to forestall autoimmune reactions to components of the damaged tissue. In addition, they also produce molecules that stimulate replication of progenitor cells, stimulate formation of new blood vessels, and inhibit the apoptosis of cells due to ischemia. Building on this hypothesis, investigations of the downregulation of the immune response by MSCs have been undertaken in a variety of autoimmune diseases. This immunomodulatory influence of MSCs has been used in the treatment of lupus erythematosus, multiple sclerosis, systemic sclerosis, and graft-versus-host disease. Many of the beneficial effects on autoimmunity and the reparative function are elicited by production of soluble mediators and through cell-to-cell interaction ( Table 1 ). Cocultures of MSCs suppress proliferation of stimulated mononuclear cells or T-lymphocytes and Fas ligand–mediated apoptosis of T cells. The interaction of T1 or T17 lymphocytes upregulates adenosine production by MSCs that suppresses these lymphocyte populations and leads to new blood vessel formation through stimulation of vascular endothelial growth factor. The interaction of MSCs and monocytes results in their production of interleukin-10 and an anti-inflammatory phenotype.
Source | Factor | Action | Reference |
---|---|---|---|
Mouse bmMSC | IL-1ra | Decreased inflammatory response to bleomycin in the lung | |
Human bmMSC | Soluble TNF receptor −1 | Attenuated systemic inflammatory response to intraperitoneal injection of lipopolysaccharide | |
Human bmMSC | TNF-α stimulated gene/protein 6 | Reduced early inflammatory response to permanent ligation of the anterior descending coronary artery and size of the myocardial infarcts | |
Human MSC | TNF-α stimulated gene/protein 6 | Reduced inflammation in a model of sterile cornea injury | |
Human bmMSC | TNF-α stimulated gene/protein 6 | Reduction of neutrophils and macrophages in model of zymosan-induced peritonitis | |
Mouse bmMSC | PGE 2 | Mouse model of sepsis induced by cecal ligation and puncture | |
Human bmMSC | PGE 2 | Increased numbers of anti-inflammatory M2 phenotype in human macrophages in vitro cocultures | |
Mouse MSC | Adenosine | Suppression of T-cell proliferation | |
Human bmMSC | Adenosine | Suppresses immune responses of Th17 cells | |
Human bmMSC | IL-6 | Skew monocytes to an IL-10 producing phenotype |
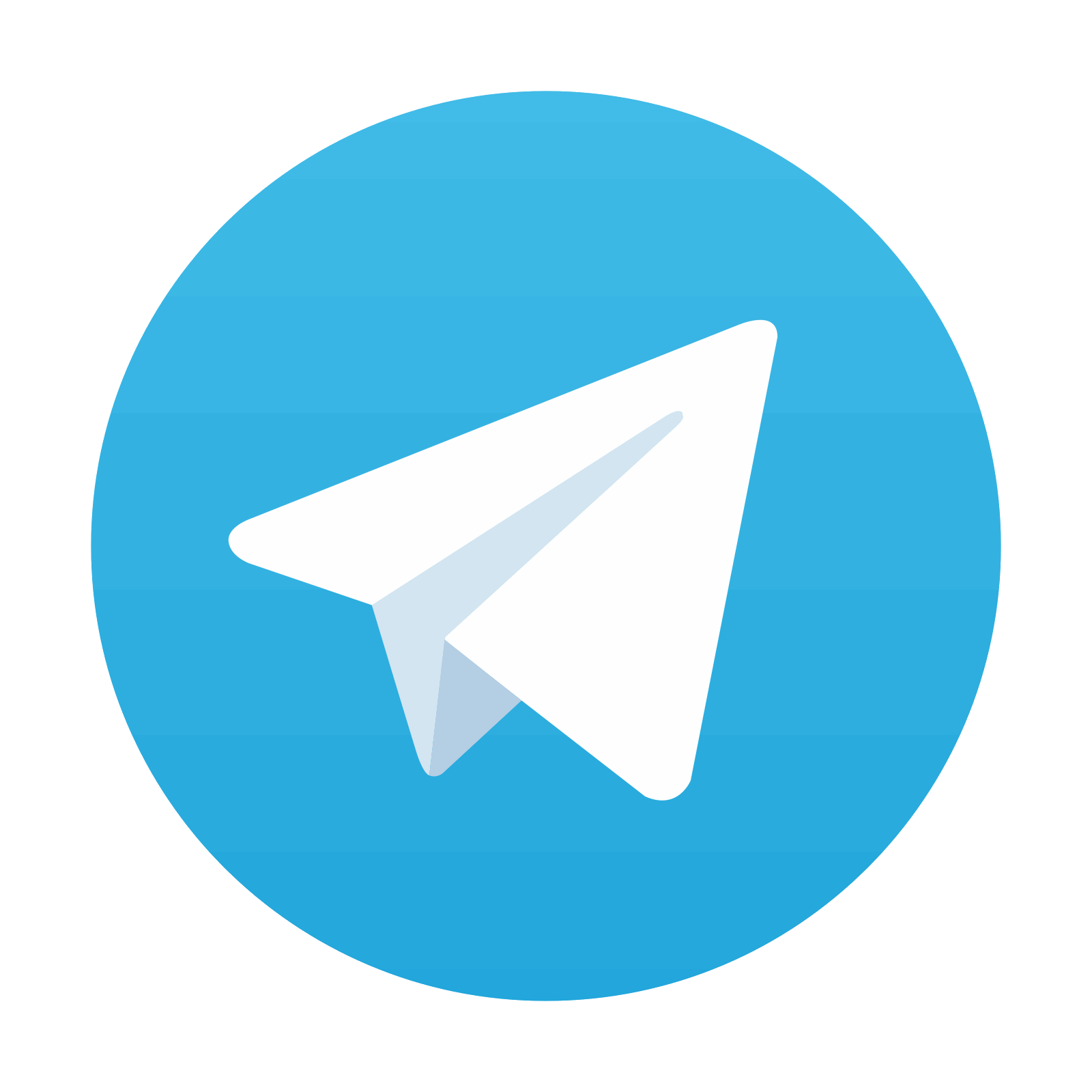
Stay updated, free articles. Join our Telegram channel

Full access? Get Clinical Tree
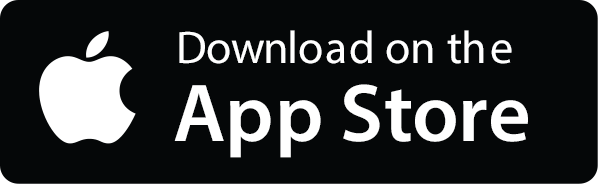
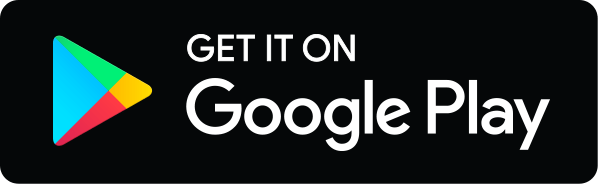
