Physiatrists are uniquely qualified to serve as sports medicine physicians and care for the recreational and competitive athlete as well as those who exercise for health-related benefits. Physiatrists are trained extensively in musculoskeletal medicine and injury, functional rehabilitation, and coordinating and leading a team of professionals to optimize care of patients. The sports medicine team includes the athlete, his or her family, specialty and primary care physicians, athletic trainers, physical and massage therapists, chiropractors, dieticians, psychologists, and coaches. Physiatrists are also skilled in prehabilitation, or preventive rehabilitation, which is an important aspect of care for any athlete involved in routine physical training.
Exercise physiology, emergency medical care, and more routine medical care are also important to any well-rounded sports medicine physician. This chapter covers the breadth of sports medicine for the physiatrist, including the general role and medicolegal aspects of being a team physician, sporting event administration with a particular focus on emergency preparedness, athletic conditioning and training principles, injury prevention and functional rehabilitation, biomechanics of sports, pharmacology in sports, emergency assessment and care of the athlete, common medical and neurologic conditions in athletes, and a review of specific populations of athletes and their common ailments, including a special focus on athletes with disabilities and adaptive sports medicine. This chapter is not intended to be a musculoskeletal medicine chapter because other chapters in this text cover in-depth musculoskeletal issues that overlap with the musculoskeletal injuries seen in athletes.
Role of the Team Physician
The team physician has multiple, overlapping roles within the framework of the team itself, the individual athletes and their families, the school or overseeing organization, and the community. The primary responsibility is caring for the health and well-being of the individual athlete. The chief duties are to determine initial medical eligibility, provide care for the injured athlete, facilitate and determine return to play, create and maintain emergency preparedness, oversee the healthfulness of the team’s overall training program, supervise the personnel providing health care for the team, and protect against institutional and personal liability. The role of intermediary for each of these groups is both challenging and rewarding. The team physician is responsible for conveying information to other medical specialists, allied health professionals, athletic trainers, and coaches, as well as for creating and coordinating health care plans for the athletes.
These multiple roles can at times be in conflict, and it is essential to recognize that the primary obligation is always to the athlete as patient. The specific details of confidentiality and the flow of information are defined by the Health Information Privacy and Portability Act. Specifically, the components of consent for treatment and the authorization to release information are most pertinent to the team physician. It is prudent to review this document and the applicable state law as well as to discuss these matters with all involved parties (e.g., athletes, coaches, families) before issues arise.
Beyond the medical obligation to the athlete, the team physician has an assumed medicolegal responsibility. Physicians receiving financial or other obvious remuneration for services are bound by normal medicolegal responsibilities. The physician might be held legally liable if injury occurs as a result of negligence or treatment below the professional standard of care. Those physicians who volunteer their services may not be covered by Good Samaritan laws, particularly if they are the “designated” medical provider for an event, regardless of whether or not they are remunerated for their services. Preparticipation physical evaluations (PPEs) are not covered under Good Samaritan laws. In some cases, athletes might be legally allowed to participate despite a disqualifying PPE.
The team physician is also responsible for the final decision regarding clearance to play or return to play (RTP) after injury. These issues can be complex, and several fundamentals must be met before the athlete should be cleared. When recovering from an injury, the athlete must have completed appropriate rehabilitation; a health care professional must document the athlete’s recovery; and the risks of return must be discussed and documented. The team physician must obtain the appropriate consultant opinions when dealing with conditions or injuries outside the scope of his or her practice. Although some states have determined that athletic participation implies assumed risk, this is not universal. Team physicians have been held liable for injury occurring after full RTP has been granted. In cases of differing opinions of the team physician and a consultant, the final responsibility typically lies with the team physician.
The traveling team physician presents a unique issue given that licensure and malpractice coverage are state-specific. There is currently a bill (Sports Medicine Licensure Clarity Act of 2015) before the 114th Congress that could protect sports medicine physicians who travel across state lines and provide care for athletes in states in which they are not licensed.
Event Administration
The two most common competition venues where the sports medicine physician practices are on the sidelines of a sporting event (e.g., football, soccer, gymnastics) and at mass participation endurance events (e.g., marathons, road races, triathlons). These are unique venues that require appropriate planning, organization, rehearsal, and communication to provide optimal medical care.
Both require significant preseason or preevent planning. From an administrative perspective, it is essential that the team physician or chief medical officer develops a chain of command that defines the responsibilities of the medical providers, emergency medical services, event officials, and other parties involved. Establishing and regularly rehearsing an emergency action plan (EAP) are critical. Reviewing the medical equipment that will be available at the event as well as the protocols for attending to an injured athlete are standard components of preparation. The team physician must also assess environmental concerns and playing conditions and have a policy to modify or suspend practice or competition if adverse conditions exist.
Administering care at a mass participation endurance event such as a marathon requires unique planning given the sheer number of participants, the unique medical illnesses encountered, the length of the course, the number of medical sites needed, and the large medical team required to staff such an event. Given its size, the potential impact of the event on the community and its resources needs to be accounted for in planning and implementation. These large mass participation events require the coordination of public, private, and medical agencies, as well as effective communication among them during race planning and race day.
Although any marathon has a known number of people requiring medical assistance (2% to 20% of participants, depending on event size, duration, and weather conditions), if these numbers should increase unexpectedly, there is a potential for the community resources to become overwhelmed if not allocated appropriately. For this reason, communication among the medical team, race officials, emergency medical services, local hospitals, and participants is crucial to help prevent and respond to incidents during the race. It is the role of the chief medical officer to coordinate these effective lines of communication.
Medical staff need to be familiar with the more common ailments that runners can present with during or after the race and to have the appropriate equipment available in advance. Exercise-associated collapse, a common medical entity seen in marathon medical tents, has a broad differential diagnosis, which includes fairly benign to potentially life-threatening disorders (see later). Appropriate medical equipment for a marathon includes devices for rapid electrolyte blood testing, cardiac monitoring capabilities, automated external defibrillators (AEDs), rectal thermometers, rapid warming and cooling protocols and equipment, and personnel and equipment for starting intravenous therapy.
Prevention of medical tent encounters is an even better way to enhance safety. Preventing race day maladies in marathoners requires educating racers and properly coordinating appropriate resources (aid and fluid replacement stations) along the course. Educate the runners in the months, weeks, and even the day before and day of the event to “listen to their bodies,” train adequately, determine/estimate fluid needs before the race and stick to a plan during the race, and adjust race/time expectations if the weather conditions are adverse. Education is an important strategy to lessen morbidity (and potentially mortality) during and after the race.
Principles of Conditioning and Training
Effective training programs require a fundamental understanding of strength, flexibility, and endurance development, as well as the basic principles of specificity, individuality, periodization, overload, and tapering. Please refer to Chapter 15 for an overview of strength, flexibility, and endurance training.
The body’s adaptation to physical training is metabolically and neuromuscularly specific to the exercise performed. The principle of sport specificity highlights the importance of training in the same sport the athlete will be competing in. For example, although cycling and cross-country skiing are valuable forms of aerobic exercise, an athlete who is training to run a marathon needs to focus on running as the major component of training to ultimately maximize running performance. It must be realized, however, that individuals respond differently to the same training stimulus. For example, a less-conditioned athlete will make larger gains in fitness than a more conditioned athlete who embarks on a training program.
The principle of overload requires that the training stimulus be greater than how the athlete normally performs in competition. Exercise frequency, duration, and intensity are manipulated to produce overload. These specific training variables (frequency, duration, and intensity) must be periodically increased for an athlete to progress ( Box 39-1 ).
F Frequency of training
I Intensity of training
T Type of exercise mode
T Time of exercise duration
Periodization
Periodization is one structured training approach that highlights this concept. It was developed by a Russian physiologist in the 1960s but has had a significant resurgence in today’s training programs ( Figure 39-1 ). In periodization, training is divided into defined “periods” to allow buildup of training stresses, time for rest and adaptation to training, and continual progression of fitness. These periods include macrocycles (commonly lasting 1 year), which are divided into shorter mesocycles (commonly lasting 1 month), which are then subdivided into microcycles (commonly lasting 1 week). Microcycles are generally the weekly training programs. Each mesocycle can be made up of 4 weekly microcycles with a gradual buildup of training frequency and intensity over the first 3 weeks and then a slight decrease in the fourth week to allow for rest and the subsequent metabolic training adaptations to occur. The athlete is then ready to enter the next 4-week mesocycle and progress through another 4 weekly microcycles. The amount and intensity of training in each mesocycle depend on where the athlete is in relationship to the competitive season, which is how macrocycles are structured.

A typical year-long training program will have three macrocycles: preseason or “buildup phase,” competitive season or “maintenance and fine-tuning phase,” and finally a postseason or “recovery phase.” The preseason macrocycle is typically the longest, occurring before the competitive season. It is designed to develop fitness in anticipation of the more intense training to follow. During the preseason macrocycle the athlete generally focuses on higher volume and lower intensity exercise.
The competitive season macrocycle follows the preseason period. The focus here is to develop and maintain peak fitness with a focus on high-intensity training and sport-specific technique drills. Because of the higher intensity training, the volume must be significantly reduced.
Finally, the postseason macrocycle is a time for the athlete to recover from the previous year’s training and physically and mentally prepare for the next year of training and competition. The early portion of this period necessitates “active rest.” The athlete generally participates in unstructured, non–sport-specific recreational activities to allow for recovery from the stresses of the competitive season. This is an important period for the athlete to adequately recover from injuries, prevent overtraining, and take a “mental break” from the competitive season.
During the competitive season, a typical microcycle includes a tapering period just before competition. The taper is a short period of reduced training before an important competition with the goal of optimizing performance. Although it is well recognized that a reduction in training before competition improves performance, it is not definitively known what is the most ideal taper strategy regarding altering training volume, intensity, and frequency. A recent metaanalysis demonstrated that the most efficient strategy to maximize performance gains appears to be a 2-week taper during which training volume is exponentially reduced by 41% to 60%. This study found that the ideal taper for endurance athletes kept training intensity and frequency stable, with only volume being progressively reduced.
Overtraining Syndrome
An important set of symptoms to be aware of for any athlete, coach, or sports medicine physician is that related to overtraining syndrome. When prolonged, excessive training occurs concurrently with insufficient recovery, the athlete might have unexplainable performance decrements resulting in chronic maladaptations leading to overtraining syndrome. Common symptoms of overtraining syndrome in addition to an unexplained performance decrement include generalized fatigue, mood disturbance, poor sleep, and increased rates of illness and injury. By definition, these symptoms persist despite more than 2 weeks of rest. The treatment is rest, from weeks to months, with gradual resumption of training. Prevention, however, is the best treatment, and following a periodized training program is one method to ensure adequate rest from more intense bouts of training.
Altitude Training
Altitude/hypoxic training is a controversial area of research and sports performance. Many elite endurance athletes incorporate altitude training into their typical training program in hope of enhancing performance in competition. For those athletes who do not live in mountainous areas, there are a variety of “altitude tents” on the market that can be used to simulate living at high altitude. Some controversy arose in 2006 when the World Anti-Doping Agency (WADA) considered placing “artificially-induced hypoxic conditions” on the Prohibited List of Substances/Methods. Another area of controversy is the uncertain primary physiologic mechanism(s) responsible for the effect of altitude training on sea-level performance. The proposed mechanisms include accelerated erythropoiesis (increased erythrocyte volume) as the primary hematologic effect, as well as nonhematologic factors such as improved muscle efficiency at the mitochondrial level, glucose transport alterations, and enhanced muscle-buffering capacity via pH regulation.
Most scientists agree that the most effective form of altitude/hypoxic training is the “live high–train low” method whereby athletes “live high” to stimulate erythropoietin and subsequently increase erythrocyte volume and “train low” at a higher intensity with improved oxygen flux to induce beneficial metabolic and neuromuscular training adaptations. The controversy here comes from not knowing what the optimal “dose” of altitude training truly is. So far, the research suggests that for athletes to derive the most beneficial hematologic effects while using natural altitude, they need to live at an elevation of 2000 to 2500 meters for 22 hours a day for 4 weeks. For those using simulated altitude environments, 12 to 16 hours of hypoxic exposure might be necessary but at a higher elevation (2500 to 3000 meters).
Injury Prevention and Rehabilitation
Injuries that occur in the course of an athlete’s life will be because of a multitude of causes. These include acute trauma, overuse, and injuries secondary to poor technique. Certain injuries are predictable and even preventable in many cases.
Kinetic Chain Assessment
The kinetic chain model is based on the idea that each complex, athletic movement is the summation of its constituent parts. For example, a quarterback’s throwing motion is created through the action of feet on the playing surface, loading of the lower limbs, rotation of the hips and abdominal muscles, activation of the latissimus dorsi, stabilization of the glenohumeral joint by the periscapular muscles, loading of the throwing arm through the deltoid and biceps, and finally from the upper limb motion of elbow extension and wrist flexion. The upper limb acts as a “funnel” for the energy generated by the core and lower limbs. Each of the “links” in the chain must function well to optimize performance and minimize potential tissue trauma.
“Catch-up” occurs when an athlete tries to compensate with one segment for a deficiency in a separate segment. This phenomenon puts higher stress on the tissues of the distal segment and predisposes it to injury. For example, a runner might present with patellofemoral pain for a variety of reasons. It could simply be an overtraining phenomenon, or it might be caused by weak or inhibited hip musculature, abnormal foot and ankle biomechanics, or poor running technique. Relief can be achieved through temporary resting, but the pain might consistently return unless the causative issue is corrected. The experienced sports medicine physician will identify the tissue diagnosis and treat it appropriately and will also identify and correct all predisposing factors. It is important that the physician is able to explain these concepts to the athlete and the treatment team to achieve optimal results.
Rather than view all similar overuse injuries as the same issue, the sports medicine physiatrist is able to break down the complex motions of the athlete into the constituent parts, identify the maladaptive pattern, and create a rehabilitation program that will prevent the injury from recurring. When examining the injured athlete, it is vital to make a biomechanical diagnosis rather than to focus exclusively on the injured tissue. Although it is easy to focus exclusively on the painful tissue, it is essential to assess the entire athlete and his or her mechanics to make appropriate tissue and biomechanical diagnoses ( Table 39-1 ).
Tissue Diagnosis | Potential Biomechanical Diagnosis |
---|---|
Lateral/medial epicondylitis | Posterior deltoid weakness |
Hamstring strain | Overly tight hamstring, weak gluteal musculature |
Metatarsal stress fracture | Supinated foot |
Athletic pubalgia | Weak core musculature, tight hip girdle |
Shoulder impingement with rotator cuff strain | Periscapular weakness or inhibition |
Patellofemoral syndrome—patellar cartilage irritation or chondromalacia | Quadriceps and gluteal weakness or inhibition, overpronation |
Repetitive ankle sprains—anterior talofibular ligament FL laxity | Weak peroneals, proprioceptive dysfunction |
Evaluating an injured athlete involves a thorough discussion of the current problem, previous athletic injuries in the same area, as well as other seemingly unrelated injuries. Discussion of training patterns, including intensity and volume, as well as competition details is important. Proper equipment fit and technique should also be assessed. Differentiating between a slowly worsening insidious process and a more acute injury is key to creating an appropriate treatment plan. These subtle distinctions can frequently reveal the underlying cause of the current injury.
The physical examination of the athlete must be focused on finding the biomechanical culprit, rather than focusing solely on the painful tissue. The athlete must be examined in detail to fully assess the possible causes of the injury. Select upper limb tests that help determine the role of the scapula in upper limb injuries include the scapular assistance test and the scapular retraction test. Core muscle function must be assessed dynamically and addressed as part of the rehabilitation plan. Examples of physical examination maneuvers to evaluate core muscular function in the athlete are demonstrated in Figures 39-2 to 39-5 . The kinetic chain assessment is designed to assess flexibility, strength, and functionality of the affected limb. The examination essentially assesses multiplane sports-specific dynamics rather than single-plane, solitary joint movements.




Proprioception and neuromuscular control are vital, and often unrecognized, components of athletic activity, and loss of these is increasingly noted as a predisposing factor for injury. Commonly called “muscle memory,” these abilities help an athlete control the limbs throughout the full spectrum of motion. Deficits here can be subtle and difficult to assess in the typical office visit. Testing must be multiplanar and mimic the athlete’s typical motion patterns.
It is vital for the examining physician to have a thorough understanding of the motions involved in the athlete’s sport to make the appropriate changes; for example, a difference of 10 degrees in knee extension during the cocking phase of a tennis serve increases the valgus load at the elbow by 21%. When necessary, this can be accomplished through discussion with the athlete, parent, coach, and/or trainer.
Prehabilitation
Prehabilitation is based on the concept that many sports injuries can be prevented if the athlete engages in an appropriate preseason prehabilitation program. Most of the scientific literature regarding prehabilitation programs focuses on noncontact anterior cruciate ligament tears. Poor form is also linked to numerous athletic injuries. Balance perturbation, plyometric training, and stretching programs all have a role in the prehabilitation of the athlete. Ideally, some of these issues can be assessed during the preseason physical examination and corrected before the start of the season. A large proportion of sports injuries are related to overuse, and it is wise to have a discussion of these issues with athletes and coaches in the preseason. Off-season cross-training, core muscle work, and cardiovascular preparation are generally considered key to a healthy season.
Prehabilitation can be viewed as an injury prevention program. These programs are sport specific, and often position specific, but always address the basic components of all athletic movements and the potential “breaks” in the kinetic chain: (1) flexibility, (2) strength, and (3) endurance. These programs can be used preseason, postseason, or during the season to treat previous injury or to prevent future injuries. For example, a soccer prehabilitation program could involve daily postactivity stretching, strength and plyometric training three times per week, and cardiovascular exercise three times per week. A thrower’s prehabilitation program might involve a focus on daily postexercise range of motion (ROM), strength training two times per week, and daily technique practice, with pitch/throw volumes increasing weekly. Additional focus can be placed on any component based on the individual athlete’s needs.
Injury Phases
Rehabilitation is guided by the status of the injured tissue, so an understanding of the timing of injury and recovery is essential. Injuries can be acute, subacute, chronic, or an exacerbation of a chronic condition. A detailed history is required because athletes will present at varying points of their tissue healing, and the treatment plan will have to be created appropriately. Four general phases are typically seen in tissue injury and repair. The physician caters the treatment to the individual tissue and the timing of the injury. The first phase involves the initial injury and the subsequent inflammation, edema, and pain. This phase is typically short, lasting days, depending on the severity of the injury. The reparative phase of the injured tissue might last from 6 to 8 weeks. It involves cell proliferation, granulation tissue formation, and neovascularization. The last phase is remodeling, which occurs as the tissue matures and realigns.
Excessive inflammatory response or exuberant repair can result in poor outcomes. For example, problems experienced during the remodeling phase can result in excessive scar tissue formation and development of recurrent/chronic injury. Many athletes return before the tissue itself is adequately healed and experience reinjury or an exacerbation of their previous symptoms. This likely occurs if the injured tissue is not fully healed or if the new tissue has not adapted to tolerate the desired motion or inherent stresses of the sport.
Stages of Rehabilitation
Rehabilitation can be viewed as a 3-stage spectrum from acute to recovery, and then to the final functional stage through return to full play ( Table 39-2 ). Each stage has individual goals that lead to the overall goal of RTP. The acute stage of rehabilitation is focused on managing the symptoms and signs of the injury. The classic PRICE (protection, rest, ice, compression, and elevation) approach is often followed. Medications, manual therapy, and physical modalities are also used during this phase. If necessary, bracing, injection, or surgery is performed to facilitate protection and future healing. ROM, strength, and cardiovascular fitness must be maintained as much as possible and tolerated. This can be accomplished by exercising the upper body of an athlete with a lower body injury. Passive ROM must be viewed with caution in the acute to subacute injury phase because it might injure the tissue, leading to increased pain and inflammation. Isometric strength exercises can be prescribed during this stage, if tolerated, to decrease pain, edema, and potential atrophy. The athlete can advance to the next stage of rehabilitation if adequate pain control and near-normal ROM is achieved.
Phase | Goal | Timing |
---|---|---|
Phase 1 (acute) | Allow injured tissue time to heal, decrease symptoms, maintain range of motion. | <72 hr |
Phase 2 (recovery) | Increase demands on the athlete; flexibility, strength, endurance training; kinetic chain corrections; maintain cardiovascular fitness through cross-training. | Variable |
Phase 3 (functional) | Advance toward full return to play, advance cardiovascular fitness. | 2 to 4 wk |
The recovery stage is often the longest part of the rehabilitation program and shifts the focus from symptom management to restoration of strength and endurance as it applies to function. Tissue healing can continue at this time and will dictate the type of treatments recommended. Therapies move from passive to active during this stage. Flexibility, strength, symmetry, and proprioception are all addressed at this time.
Strength exercises advance from isometric to isotonic, including both concentric and eccentric movements. Open-chain exercises are ones in which the distal segment is free, whereas closed-chain exercises are ones in which the distal segment is fixed. Closed-chain activities are designed to stabilize an injured segment or to decrease the force passing across a joint. They also allow for a stepwise approach to kinetic chain rehabilitation. The rehabilitation program should typically focus on correcting erroneous movement patterns rather than creating completely new patterns for the athlete to learn.
In the case of an athlete with a shoulder injury, the rehabilitation program moves from predominantly closed-chain exercises, to axial-loaded exercises, to open kinetic chain exercises, to full sport-specific rehabilitation. Kinetic chain rehabilitation is modified to the individual athlete’s symptoms and dysfunction. Rather than view this type of rehabilitation as a series of discrete steps, the physiatrist should consider it as a transition from dysfunction to function and adjust the treatment plan and timing accordingly.
The parameters of the strength training program (repetitions, resistance, speed, multijoint movements) can be adapted during this time, depending on the clinical scenario. Preexisting kinetic chain issues or tissue overload issues from the current injury are addressed at this time as well. As the rehabilitation program continues, increasingly athletic maneuvers (running, jumping, cutting) are used. Neuromuscular control (NMC) involves proprioception, muscle control, and the interplay between them. Without sufficient NMC, the athlete might continue to be predisposed to future injury. This recovery phase is the most variable and has the highest potential for reinjury, as the tissue is actively remodeling. The athlete must be reevaluated frequently during this phase because setbacks are common and can require modification of the rehabilitation plan. The athlete can advance to the final stage once pain-free ROM is achieved and strength is 75% to 80% or greater (compared with the noninjured side).
During the final functional stage of rehabilitation, the focus remains on kinetic chain issues and technique errors. Strength balance, power, endurance, functional ROM, and NMC are aggressively addressed. Sport-specific drills are used during this stage and advanced to include practice. Full RTP is achieved when the injury is no longer painful; when there is normal flexibility, strength, and proprioception; and when appropriate sport-specific mechanics and sport-specific skills are achieved and reproducible. In many cases, this rehabilitation phase becomes the maintenance program.
Biomechanics of Sports
Throwing
Pitching a baseball is a classic example of the body acting as a kinetic chain of motion where foot placement and ankle ROM will affect how the pitcher uses his or her shoulder girdle and subsequently throws the ball. If there is a “weak link” in the pitcher’s kinetic chain, there can be a predisposition to injury because of the repetitive nature of pitching. Whole body activity is involved in transferring momentum from the body to the ball. The momentum begins with a drive from the large leg muscles and rotation at the hips, progressing through segmental rotation of the trunk and shoulder girdle, transferring energy through elbow extension, next through the small forearm and hand muscles, and finally to the ball. This transfer of momentum and subsequent energy is related to a series of body segment accelerations and decelerations. When an initial segment of the body (e.g., trunk) accelerates, the next segment (e.g., arm) within the kinetic chain is left behind. When the trunk decelerates, the momentum is transferred to the arm with an increased velocity of the arm that is accentuated by the forces acting on the shoulder/arm. This motion and the forces are ultimately transferred to the ball.
Approximately 50% of the velocity of a pitch results from the step and body rotation (from the potential energy stored in the large leg and trunk musculature). The other 50% comes from the smaller muscles of the shoulder, elbow, wrist, and hand. When forward stride is not allowed, the peak velocity of a pitched ball decreases to 84%. When the lower body is restricted, it decreases to 64%. Peak velocities in water polo are approximately 50% that of baseball because of the lack of a ground reaction force.
The baseball pitch is composed of six phases ( Figure 39-6 ). Understanding these phases is critical to understanding, diagnosing, preventing, and treating throwing injuries.

Windup begins when the pitcher initiates motion and ends with maximum knee lift of the lead leg when the ball is removed from the glove. Push-off of the stride leg allows the center of gravity of the body to move forward. Few injuries occur in this early phase because the shoulder musculature is relatively inactive and most forces arise in the lower half of the body.
Early cocking is next and is often called the stride phase. The stride leg extends toward the batter, as does the knee and hip of the pivot leg, propelling the body forward into the stride. As the hips rotate forward, the trunk follows and subsequently, the throwing shoulder abducts, extends, and externally rotates, leaving the shoulder in a “semicocked” position. Again, in this early phase, there is less risk of injury because most forces are still being generated in the trunk and lower limbs; however, shoulder activity does become more evident. The trapezius and serratus anterior demonstrate moderate to high activity to protract and upwardly rotate the scapula. The middle deltoid generates the abduction force, and the supraspinatus fine-tunes humeral head positioning within the glenoid.
The next phase is late cocking. The hallmark of this phase is when maximal shoulder external rotation is obtained. The shoulder begins this phase in approximately 50 degrees of external rotation and ends in about 175 degrees at maximal external rotation. This extreme amount of rotation allows the greatest accelerating force to the ball over the greatest possible distance. The amount of external rotation obtained correlates with the speed of the pitched ball. A majority of injuries occur in the late cocking and deceleration phases of throwing ( Box 39-2 ). For late cocking, this is caused by the forces needed to stabilize the shoulder in this extreme ROM. The dynamic stabilizers of the anterior shoulder (long head of the biceps, subscapularis, and pectoralis major) are very active in this phase. The static stabilizers (glenohumeral ligaments, capsule, and labrum) are active as well. The glenohumeral ligaments and capsule increase in laxity because of the extreme ROM in the overhead athlete. This laxity is necessary for performance; however, overstretching these ligaments enhances the work of the dynamic stabilizers with a resultant potential for injury.
- •
Anterior instability
- •
Internal (posterior-superior) impingement
- •
Type II SLAP lesion
- •
Articular surface rotator cuff tears
- •
Bicipital tendinopathy
- •
Medial tension injuries at the elbow
- •
Ulnar collateral ligament injury
SLAP, Superior labrum, anterior to posterior.
During the acceleration phase, the shoulder is powerfully internally rotated from 175 degrees of external rotation to 90 to 100 degrees at ball release. Shoulder abduction is relatively fixed in all throwers at 90 degrees. Trunk lateral flexion (and not shoulder abduction) generally determines what position the arm is in relative to the vertical plane. “Over the top” throwers have a greater amount of contralateral trunk flexion, and “sidearm” throwers have less. If there is less than 90 degrees of abduction as a result of fatigue, weakness, or just poor form, the common observation is a “dropped elbow.” “Hanging” or dropping the elbow results in a reduced pitch velocity and an increased risk of injury to the shoulder (rotator cuff) and the elbow (ulnar collateral ligament injury). Another common injury in the acceleration phase, particularly in older pitchers, is from subacromial impingement as they internally rotate and adduct the abducted arm during acceleration.
The deceleration phase is manifested by large eccentric muscular forces of the posterior shoulder girdle to decelerate that rapid internal rotation of the acceleration phase. Deceleration begins after ball release and ends when the arm reaches 0 degrees of internal rotation. The posterior shoulder girdle is active in this phase, including the scapular muscles, rotator cuff external rotators (particularly the teres minor), and the posterior deltoid. Because of these large eccentric contractions, injury is common in this phase ( Box 39-3 ).
- •
Posterior instability
- •
Isolated rotator cuff tears
- •
SLAP lesions
SLAP, Superior labrum, anterior to posterior.
The final phase of pitching is the follow-through phase. This is a “passive” phase in which the body is merely catching up with the throwing arm. Low-grade eccentric loading of the shoulder musculature and little risk of injury are noted during this phase.
Because of the high risk of overuse injury in the young pitcher’s throwing arm, age-based pitch count guidelines have been developed to decrease this risk ( Table 39-3 ). For similar reasons, curve balls and sliders should not be pitched until the athlete reaches puberty. It is also recommended that young pitchers not compete in baseball more than 9 months per year. During those 3 “off” months, they should not compete in other overhead arm sports, such as competitive swimming or javelin throwing.
Age | Pitches Per Game | Per Week | Per Season | Per Year |
---|---|---|---|---|
9-10 | 50 | 75 | 1000 | 2000 |
11-12 | 75 | 100 | 1000 | 3000 |
13-14 | 75 | 125 | 1000 | 3000 |
Running
Notable differences are observed between a walking gait cycle and a running gait cycle. One in particular is a third phase in running called the float phase. Float is a period when neither foot is in contact with the ground. It occurs at the beginning of initial swing and the end of terminal swing ( Figure 39-7 ).

The walking gait cycle has a period of double limb support, which is not present in running; it occurs in the first and last 10% of the stance phase. The stance phase of gait is decreased from 60% while walking to 30% while running and 20% while sprinting. Consequently, as speed increases, the stance phase decreases. As speed increases, the velocity and range of lower limb motion increase, which in turn serve to minimize vertical displacement and improve efficiency. The body lowers its center of gravity by increasing hip flexion, knee flexion, and ankle dorsiflexion; consequently, the major kinematic difference between walking and running occurs in the sagittal plane of motion.
Faster running also changes foot contact. In slower running and walking, contact is typically heel to toe. As running speed increases, foot strike occurs with the forefoot and heel simultaneously, or the forefoot strikes initially followed by the heel lowering to the ground. In sprinting, the athlete maintains weight bearing on the forefoot from loading response to toe-off.
The angle of gait is different between walking and running. This is the angle between the longitudinal bisection of the foot and the line of progression. This angle is 10 degrees in walking. In running, it approaches 0 degrees as foot strike is on the line of progression to allow more efficient locomotion by limiting deviation of the center of gravity.
Barefoot running, simulated by running in minimalist shoes, has gained popularity over the past decade. Minimalist shoes are generally defined (and considered different from conventional running shoes) by less heel cushioning and a “zero drop” from heel to toe, meaning that the shoe’s heel contour is not built-up so that the heel is at the same level as the forefoot. Running in minimalist shoes tends to move initial contact to the forefoot and lessens impact forces, which could potentially lessen overuse injuries in runners (though this has not been proven). Runners who run in minimalist shoes tend to forefoot strike and have an increased stride cadence. However, it is not known if the shoes or if changing a runner’s stride (increased cadence with a shortened stride and running “light and quiet”), which minimalist shoes facilitate, is the driving factor for lessening impact forces and thus potentially lessening injury rate.
Swimming
Four competitive strokes are used in swimming: freestyle (crawl), backstroke, butterfly, and breaststroke. Four phases of the swim stroke are common to freestyle, backstroke, and butterfly. The first phase is the entry or catch. This encompasses hand entry into the water until the beginning of its backward movement. The propulsive phase is divided into two separate phases: pull and push. The pull phase ends as the hand arrives in the vertical plane of the shoulder. During the push phase, the hand is positioned below the shoulder and pushes through the water until its exit from the water historically at the level of the greater trochanter. The final phase is the recovery phase, which entails the aerial return of the hand.
During the propulsive phases of swimming, the arm is moved through adduction and internal rotation starting from a stretched position of abduction and external rotation. The pectoralis major and latissimus dorsi are the major contributors to this motion; however, the serratus anterior and the internal rotator function of the subscapularis and teres provide a major assist.
During the recovery phase, scapular retraction is provided by the rhomboids and middle trapezius, whereas shoulder external rotation is provided by the posterior deltoid, teres minor, and infraspinatus. In preparation for hand entry during midrecovery, the serratus anterior and upper trapezius upwardly rotate the scapula for shoulder stabilization.
Kick patterns are also an important part of swimming mechanics. In the flutter kick for the freestyle stroke, the knees should flex only about 30 to 40 degrees, and hip flexion should be minimal. The breaststroke kick is a whip-kick that creates a significant valgus moment at the knee. Because of this increased knee valgus, medial knee injuries in breaststrokers are common. The pain can be from a medial collateral ligament sprain or a medial plica/synovitis.
General rehabilitation and prehabilitation principles have been established for swimmers with shoulder pain, and all sports medicine clinicians should be familiar with them. The overarching principle is that most shoulder pain in swimmers (impingement and rotator cuff tendinopathy) is caused by dynamic muscle imbalances, weakness, and biomechanical faults, and not hard anatomic factors. A major tenet of shoulder rehabilitation for the swimmer is scapular stabilization, with a prime focus on endurance training of the serratus anterior and lower trapezius. The serratus anterior is a muscle of particular focus because it has been demonstrated to function at 75% of its maximum test ability in swimming and is active throughout the swim stroke cycle. Other tenets of shoulder rehabilitation in swimmers include stretching the internal rotators and posterior capsule and cervical and thoracic mobilization.
Jumping and Landing
The biomechanics of jumping and landing in sports is particularly well researched in the setting of noncontact anterior cruciate ligament (ACL) injuries of the knee. Noncontact ACL injuries occur more frequently with the knee in less flexion. In this position there are greater knee extensor loads with greater forces creating anterior tibial translation and subsequent ACL injury. A well-defined gender difference observed in jumping and landing mechanics is likely one reason for the higher rate of noncontact ACL injuries in female athletes. Female athletes land more erect with less knee and hip flexion. They land with less hip external rotation and abduction. They also generally have an imbalance of increased quadriceps to hamstrings activation ratio, creating greater knee extension and lesser knee flexion forces. One goal of ACL injury prevention programs is to improve jumping and landing technique by increasing knee and hip flexion during landing and balancing the quadriceps to hamstring activation ratio.
Pharmacology in Sports
“Doping” refers to any substance or method used to increase performance, possibly to the detriment of the health of the athlete or the ethics of the competition. WADA was created to unify the fight against doping. WADA rulings are accepted by all National Olympic committees, the International Olympic Committee (IOC), International Paralympic Committee (IPC), national governments, and international sports federations. The agency, based in Montreal, Canada, consists of government representatives from all continents and members of the Olympic movement. The World Anti-Doping Code was developed in 2003 and enforced in 2004, with a complete listing of banned substances and methods. A brief history of doping in sports is presented in Box 39-4 .
- •
World War II—Steroids used in the belief that male hormones could benefit the emaciated prisoners rescued from concentration camps
- •
1950s-1960s—Amphetamines and anabolic steroids widely used by athletes
- •
1962—International Olympic Committee passed a resolution against doping, banned steroid use
- •
1967—Medical commission started medical controls in 1968 Olympics
- •
1976—Anabolic steroids controlled for the first time in Olympics
- •
1988—First gold medal stripped in track and field because of doping
- •
1990—The Anabolic Steroid Act passed
- •
1998—The World Anti-Doping Agency formed in Montreal, Quebec, Canada
- •
2003—The World Anti-Doping Code is passed
A summarized list of substances banned by WADA is available online. Banned substances are divided into those that are banned in and out of competition and those that are banned in competition only. The lists of drugs and supplements banned by the National Football League (NFL), National Collegiate Athletic Association (NCAA), and other organizations are also available online.
When an athlete has an illness or condition that requires treatment with a banned medication, a therapeutic use exemption (TUE) can provide the authorization to take the needed medicine. Per WADA, all international federations and national antidoping organizations must have a process in place whereby athletes with documented medical conditions can request a TUE.
The following is a brief overview of therapeutic and performance-enhancing drugs and supplements, all of which are given careful consideration by these agencies.
Therapeutic Drugs
Analgesics
Acetaminophen can be linked to decreased muscle building after exercise (to an extent similar to that of ibuprofen). Prostaglandins are normally released after eccentric resistance exercise. This response might be blunted after consumption of maximal doses of ibuprofen or acetaminophen, profoundly influencing the anabolic response of muscle to this type of exercise.
Antiinflammatories
One study found that 15% of high school football players used nonsteroidal antiinflammatory drugs (NSAIDs) daily. Although the pain relief provided by NSAIDs might enhance performance, NSAIDs are not considered ergogenic. NSAIDs can mask pain and interrupt a natural defense mechanism for preventing injury. Because of their antiinflammatory effects, NSAIDs likely inhibit the production of prostaglandin E2, which is known to play a role in bone healing. Consequently, in those with suspected or known fractures, NSAIDs might be contraindicated.
Corticosteroids are potent antiinflammatories. In general, when given orally, rectally, intravenously, or intramuscularly, their use is prohibited by WADA. Topical preparations for skin, eye, ear, nose, or buccal cavity or for iontophoresis are allowed. For applicable athletes, epidural and intraarticular steroid injections, as well as inhaled steroids, require a TUE.
Antihypertensives
Experts recommend diuretics as first-line treatment of hypertension in the general population, possibly with early addition of a β-blocker or angiotensin-converting enzyme inhibitor (ACE-I). ACE-I or calcium channel blockers can be considered first-line agents for treatment of hypertension in athletes because diuretics and β-blockers have side effects that may hinder performance. The detection and management of hypertension and the efficacy of various agents are beyond the scope of this chapter. Rather, this discussion will focus on the aspects of these medications that affect athletes.
Diuretics can decrease plasma volume, cardiac output, and peripheral vascular resistance. Dehydration and electrolyte alterations might result in cramps or even heat stroke. For amateur athletes, the benefits of antihypertensive therapy with diuretics likely outweigh the risks. In the case of elite athletes, diuretics are banned in part for their theoretical ability to increase urine output and mask use of other banned agents (although this method rarely works optimally).
β-Blockers can reduce exercise tolerance by increasing perceived effort. They can also inhibit glycolysis and glycogenolysis with resulting hypoglycemia after exercise. The negative chronotropic effects of β-blockers can decrease heart rate recovery after exercise. β-Blockers are banned in certain sports because of their anxiolytic effects.
Diabetes Drugs
Insulin doses might need to be adjusted for persons with insulin-dependent diabetes starting a new exercise program. A 20% to 40% reduction is typical because of increased insulin sensitivity with exercise. High-intensity exercise (i.e., greater than 80% maximum oxygen consumption [V o 2max ]) can cause a temporary increase in blood glucose secondary to increased sympathoadrenal activation. In that situation, supplemental insulin, if used, should be given at a smaller dose than that given for hyperglycemia at rest.
Intramuscular injections of insulin should be avoided because muscle contraction can accelerate insulin absorption. Heat can increase absorption rates of insulin, although cold can decrease them. Therefore athletes with insulin-dependent diabetes mellitus should avoid modalities that use extremes of temperature, such as hot or cold whirlpools. Extreme ambient temperature can also reduce insulin action in athletes.
Asthma Drugs
Exercise-induced bronchospasm (EIB) can be treated effectively with a short-acting β-agonist, such as albuterol, within 15 minutes before exercise. If not sufficient to prevent symptoms, cromolyn (a mast cell stabilizer) can be added. Inhalation of either cromolyn sodium or a β-agonist, or both, 15 minutes before exercise is almost always successful in blocking EIB and airway inflammation.
For patients who have chronic persistent asthma (forced expiratory volume in 1 second less than 80% of predicted and symptoms greater than twice a week), inhaled corticosteroids are standard treatment. Inhaled corticosteroids do not appear to have ergogenic or anabolic effects.
Performance-Enhancing Drugs
Anabolic Steroids
It is estimated that 1 to 3 million athletes in the United States alone have used anabolic steroids (ASs), with annual market sales well in excess of $100 million. The Centers for Disease Control and Prevention found an overall prevalence of lifetime steroid use in females, grades 9 to 12, to be 3.2%. As many as 1 in 10 steroid users is a teenager. One study found that 6.3% of high school varsity football players were current or former AS users.
ASs have three general effects that enhance athletic performance. By binding androgen receptors, ASs stimulate messenger RNA synthesis, increasing structural and contractile protein synthesis and producing an anabolic state. ASs are also anticatabolic via competitive inhibition of the glucocorticoid receptor, inhibiting the catabolic effects of cortisol and preserving muscle mass. Finally, ASs have emotional effects, pushing athletes to train more intensely and more often.
Because ASs are illegal, studying their health risks and side effects is a challenge. Inconsistent formulations, dose administrations, and training habits make it difficult to derive statistically sound conclusions. Some studies show minimal effects on body composition and strength; although others show that supraphysiologic doses of testosterone (or its derivatives) can lead to an increase in lean mass and muscle size in humans ( Table 39-4 ). Typical users of steroids can take 10 to 40 times the prescribed dosage for disease states. Users often share needles, at a rate of up to 25% in adolescents, with reports of HIV, hepatitis B and C, and abcesses. Premature deaths have resulted from AS use, most commonly as a result of suicide and acute myocardial infarction.
System | Effects |
---|---|
Cardiovascular | Increases in total LDL Decreases in HDL Hypertension Myocardial ischemia (imbalance between myocardial oxygen supply and demand) Myocardial infarction Cerebrovascular accident |
Hepatic | Hepatocellular dysfunction (abnormal liver function tests) Peliosis hepatis (benign intrahepatic vascular disorder) Hepatocellular carcinoma |
Male reproductive | Oligospermia, azoospermia Gynecomastia Decreased testicular size |
Female reproductive | Decreased LH, FSH Decreased progesterone Menstrual irregularities Male pattern alopecia Hirsutism (irreversible) Clitoromegaly (irreversible) Deepening voice (irreversible) |
Skeletal | Premature closure of the epiphyses |
Skin | Acne (increased number of sebaceous glands) Striae |
Psychologic | Decreased libido Mood swings Hypermania/hypomania Aggressive behavior (“roid rage”) Withdrawal Depression Addiction |
The Anabolic Steroid Control Act of 1990 prohibited the use of steroids for any use other than disease treatment, thereby classifying ASs as schedule III drugs within the Controlled Substances Act. ASs are banned by all major sporting leagues, the IOC, and the NCAA.
Erythropoietin and Blood Doping
Endurance athletes are particularly sensitive to the oxygen-carrying capacity in their blood. For years, athletes sought a competitive advantage by training at altitude or sleeping in altitude tents. This effect can be reproduced by blood doping, blood transfusion, or administration of the drug recombinant human erythropoietin (rhEPO).
Erythropoietin (EPO) is a glycoprotein hormone produced mainly in the kidney in response to tissue hypoxia. EPO is part of a negative feedback cycle that controls tissue oxygen delivery by controlling the number of erythrocytes in the blood. Both rhEPO and transfusions have been shown to increase V o 2max .
The risks of artificially elevated hemoglobin/hematocrit include stroke, myocardial infarction, and pulmonary embolism. Blood transfusions, even conducted according to hospital procedures, carry risks for infection, including HIV and hepatitis, as well as transfusion reaction.
Stimulants
Stimulants might be the most common and underrecognized supplement used by high school athletes. Common stimulants include caffeine, ephedrine (ephedra or ma huang), pseudoephedrine, phenylephrine, amphetamines, and methamphetamines. Stimulants increase arousal, respiratory rate, heart rate, and blood pressure. Side effects include dizziness, insomnia, agitation, restlessness, anxiety, confusion, paranoia, hallucinations, dyskinesias, gastrointestinal disturbances, heat intolerance, stroke, myocardial infarction, arrhythmia, and death.
Pseudoephedrine (Sudafed) is a commonly used drug in this class. Although improved cycling power output and pace in a 10-km run have been demonstrated in studies of this drug, other trials contradict these findings. Pseudoephedrine is a chemical precursor in the illicit manufacture of methamphetamine. Federal policies now restrict sales by limiting purchase quantities to consumers of a minimum age. Pseudoephedrine is monitored in competition but is not included on the prohibited list.
The herbals ephedra and ma huang have similar structural properties and physiologic effects to pseudoephedrine. Ephedra and ephedra-containing products were taken off the market in 2004. Ephedra is banned by the NCAA, IOC, and major league organizations.
Caffeine is an adenosine receptor antagonist with stimulant properties. Studies have shown that caffeine is ergogenic in most, if not all, exercise situations, with few negative effects during exercise. Caffeine acts by binding to adenosine receptors in most tissues, including brain, heart, smooth muscle, fat, and skeletal muscle, with a wide spectrum of interacting responses. Caffeine also stimulates the secretion of epinephrine and the central nervous system and enhances peripheral neuromuscular transmission and muscle contractility. Side effects of caffeine include nausea, heart palpitations, headache, and muscle tension. Caffeine might also act as a diuretic, although no studies have shown a risk for dehydration in athletes. Caffeine is monitored in competition but has not been prohibited by WADA since 2004.
Supplements
A drug is any substance that exerts an effect on a body system, but a supplement is defined as a substance taken to augment the diet. Regulatory bodies within the U.S. government handle drugs and supplements differently, and supplement manufacturers are not held to the same standards as drug companies. Supplement manufacturing is overseen by the U.S. Food and Drug Administration (FDA) and treated as food as long as no drug claims are made.
Most experts agree that a well-balanced, isocaloric diet of commonly available food is sufficient to guarantee basic nutritional requirements for most athletes, including macronutrients (carbohydrates, proteins, and lipids) and micronutrients (vitamins, minerals, and trace elements). Despite this, many athletes take megadoses of essential nutrients as dietary supplements (amino acids, vitamins, minerals), at times above tolerable levels. The supplement industry has been estimated to earn $1.2 to $3 billion per year. Surveys have found that 25% to 38% of high school athletes used supplements. A 2002 metaanalysis found that, of more than 250 dietary products available, only β-hydroxy-β-methylbutyrate and creatine supplements have sufficient scientific evidence to conclude that they significantly augment lean body mass and strength with resistance training.
Creatine
According to one source, 90% of weightlifters and bodybuilders in the United States are regular users of creatine (Cr). They tend to be male, have an average age of 32 years, attended college, and have a substantial income. In a survey of NFL trainers and team physicians, all teams had players actively taking the supplement, with estimated usage 33% to 90%.
Cr, a naturally occurring compound made from amino acids glycine, arginine, and methionine, is the most popular nutritional supplement on the market. It is proposed that Cr phosphate supplementation benefits short-duration, high-intensity, repetitive exercise by enhancing adenosine triphosphate regeneration.
Cr appears to be most effective for activities that involve repeated short bouts of high-intensity physical activity, such as jumping, sprinting, and cycling. When maximal force or strength is the outcome measure, Cr has consistently been found to significantly affect force production regardless of sport, sex, or age.
Common side effects of Cr include muscle cramping and gastrointestinal distress. A number of cases of renal failure have been reversed by withdrawal of Cr supplementation. It is notable that most studies in the literature were short term with healthy subjects. Also, there are insufficient data on the possible effects of Cr on other Cr-containing tissues, such as the brain, cardiac muscle, or testes.
Because it is not classified as a drug, Cr is not under direct regulation by the FDA and is widely available over the counter. The NCAA does not allow member teams to provide Cr to its players. According to one survey, 40% of NFL teams provided Cr for their players.
For a summary of drugs and supplements used in sports, see Table 39-5 .
Drug/Supplement (Street Name in Parenthesis) | Postulated Effect | Evidence of Effectiveness | Adverse Effects | Legal Status |
---|---|---|---|---|
Anabolic steroids (testosterone or “T,” designer steroids) | Increase lean muscle mass | Effective | Significant, multiple systems | Illegal unless prescribed for disease state |
Androstenedione and DHEA (Andro) | Increase testosterone and lean muscle mass | No effect with androstenedione; DHEA with some benefits in patients >70 years old | Possible cardiovascular effects | Prohibited by WADA, NFL, and NCAA; androstenedione is illegal. |
β-Hydroxy-β-methylbutyrate (HMB) | Decrease protein breakdown; increase synthesis | Mixed | None, but long-term data lacking | Legal |
Blood transfusion or erythropoietin (doping, EPO) | Increase endurance and oxygen delivery | Effective | Significant cardiovascular risks | Prohibited by WADA, NFL, and NCAA |
Caffeine | Increases energy, systemic effects not fully known | Effective | Minimal | Legal |
Creatine | Increase muscle mass and strength | Effective | Few case reports of renal failure; proposed risk of dehydration | Legal |
Ephedrine, pseudoephedrine (Ma huang, guarana) | Increases energy, metabolism, decreases fatigue | Mixed | Significant cardiovascular, psychiatric risks, and others | Prohibited by WADA, NFL, and NCAA |
Human growth hormone | Increase muscle protein synthesis and strength | Lack of evidence | Significant | Illegal unless prescribed for disease state |
Preparticipation Examination
The primary goals of the PPE are to (1) identify life-threatening conditions, (2) identify conditions that can limit competition, (3) identify factors that predispose the athlete to injury, and (4) meet the legal requirements of the institution and state. Added opportunities are to discuss preventative health and high-risk behaviors, establish rapport with the athlete, and evaluate the general health of a potentially underserved population. The preparticipation physical evaluation (PPE) is a consensus publication by the major sports medicine associations and is an excellent guide for the team physician. It encourages safe participation and disqualifies less than 1% of high school athletes and 0.2% of college athletes. However, 14% of athletes have been found to require further evaluation. The PPE should be performed with enough lead time to allow appropriate subsequent investigation before the season begins.
The PPE is not intended as a substitute for a comprehensive visit with a primary care physician. It typically does not allow for extended time for individual education, but it is a potential opportunity for the sports medicine physician to identify factors that are associated with future injury. When appropriate, the athlete should have an extended musculoskeletal examination assessing flexibility, strength, technique, and neuromuscular control. If abnormalities are found, the athlete should be referred to physical therapy for a prehabilitation program.
The most common settings for the PPE include individual examinations performed in the physician’s office, station-based examinations, and group locker room examinations. Although individual examinations are the most commonly performed, station-based examinations might lead to more effective identification of musculoskeletal issues. Individual examinations have the advantages of privacy and more opportunity to discuss other general health and safety issues.
Current reviews of systems, medical history, surgical history, injury history, gynecologic history, family history, and vaccination history are recorded. Screening questions regarding performance-enhancing drugs/supplements, nutrition, drug, alcohol, and medication (including over the counter and supplement) use are also reviewed.
Height, weight, vital signs, hearing examination, and visual examination are performed and recorded. Normal values for blood pressure in the adolescent and pediatric population should be available for the examiner. The physical examination can be performed by different providers but must include the head, ears, eyes, nose, throat, cardiovascular system, pulmonary auscultation, abdomen, genitalia (males only), hernia palpation, skin, and neurologic and musculoskeletal systems ( Figure 39-8 ). The female genitourinary examination is deferred to the primary care physician.

Many subspecialties might be involved in the PPE. The lead physician must be determined before the decision-making process begins. It is also vital for individual providers to be able to identify suspicious findings outside their own area of specialty and refer to the appropriate specialists when necessary. A provider who is unsure of a finding should always refer to a specialist for further investigation or consultation on final clearance to play, rather than make the decision on his or her own.
Clearance to Play
The lead physician must make the determination to clear the athlete without restrictions or with recommendations for further evaluation or treatment. Alternatively, athletes might not be cleared for participation in certain sports or might not be cleared for participation in any sport.
Cardiovascular Screening
Sudden cardiac death (SCD) is a rare and devastating condition seen at all levels of sport. The American Heart Association’s (AHA) recommendations for cardiovascular screening include specific questions regarding family history, personal history, and a thorough physical examination ( Box 39-5 ). Controversy exists in sports medicine regarding screening electrocardiograms (ECG) and echocardiography. The AHA currently does not recommend a screening 12-lead ECG, echocardiograms, or exercise stress testing for athletes without risk factors. The IOC and the European Society of Cardiology recommend a screening ECG as part of the PPE, in part because of different etiologies of SCD between the United States and Europe. The AHA decision is based on the low prevalence of SCD, limited resources, impracticality of mass screening, and lack of a physician base to interpret the ECG, as well as the consequences of false-positive test results. Hypertrophic cardiomyopathy (HCM) is the most common cause of SCD in athletes in the United States. It is an autosomal dominant disease, and detailed family history might detect premature cardiac death and lead to further diagnostic testing in the athlete. Echocardiography is the most effective way of diagnosing HCM but would require the screening of 200,000 athletes before finding one with the condition.
Medical History
Personal medical history
- 1.
Exertional chest pain/discomfort
- 2.
Unexplained syncope/near syncope
- 3.
Excessive exertional and unexplained dyspnea/fatigue
- 4.
Previous recognition of heart murmur
- 5.
Elevated systemic blood pressure
Family history
- 1.
Premature death (sudden and unexpected, or otherwise) before 50 years as a result of heart disease, in ≥1 relative
- 2.
Disability from heart disease in a close relative <50 years of age
- 3.
Specific knowledge of certain cardiac conditions in family members: hypertrophic or dilated cardiomyopathy, long QT syndrome, or other ion channelopathies, Marfan syndrome, or clinically important arrhythmias
Physical Examination
- 1.
Heart murmur
- 2.
Femoral pulses to exclude aortic coarctation
- 3.
Physical stigmata of Marfan syndrome
- 4.
Brachial artery blood pressure (sitting position)
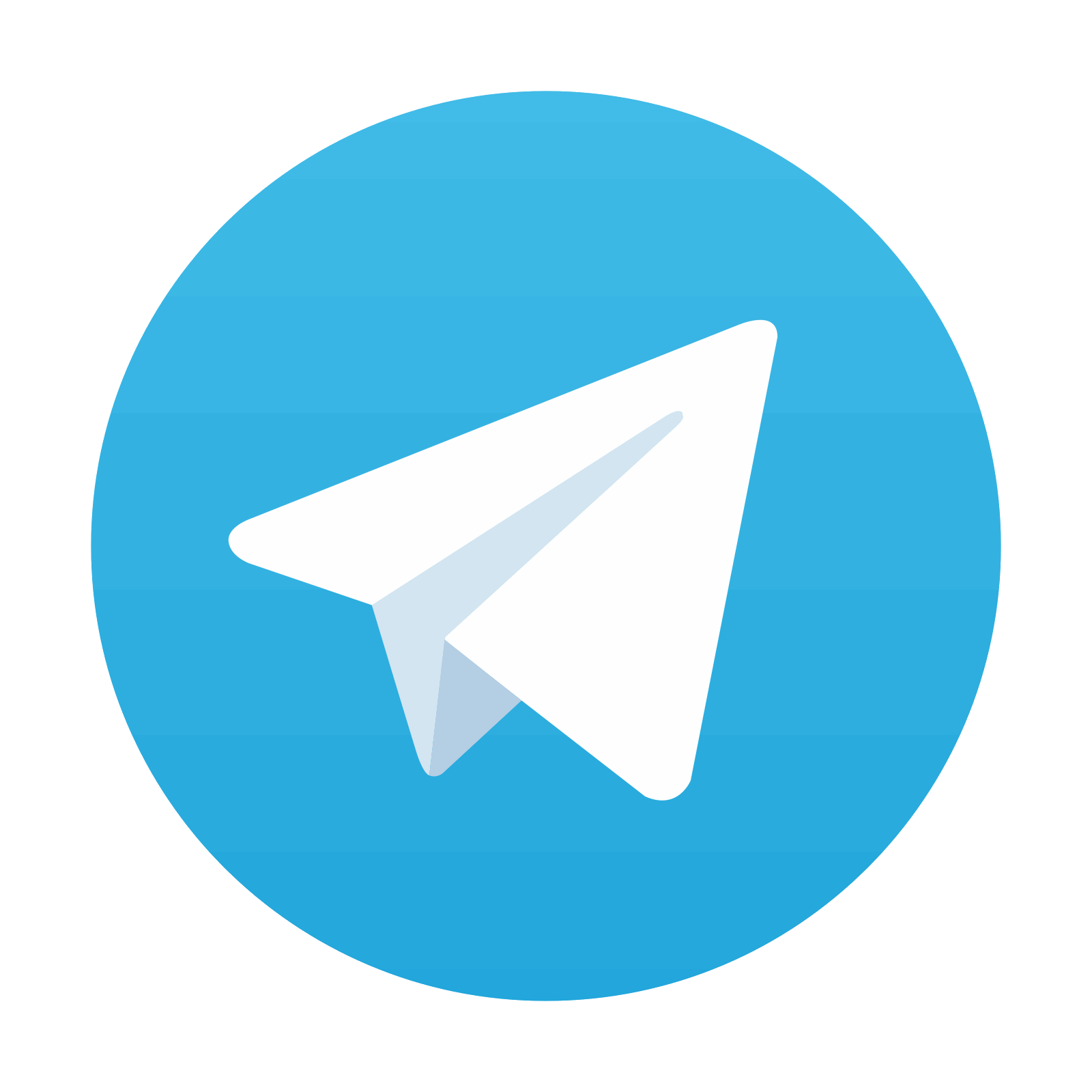
Stay updated, free articles. Join our Telegram channel

Full access? Get Clinical Tree
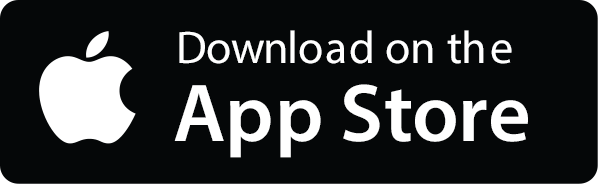
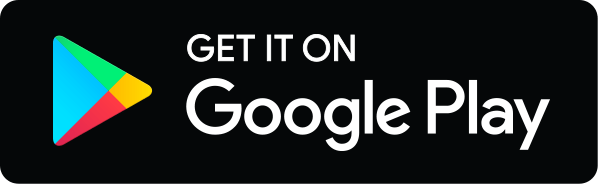
