Spinal Cord Injury: Pharmacologic Agents, Thermal Cooling, and Timing of Interventions
Allan R. Martin, MD
Michael G. Fehlings, MD, PhD
Neither of the following authors nor any immediate family member has received anything of value from or has stock or stock options held in a commercial company or institution related directly or indirectly to the subject of this article: Dr. Fehlings and Dr. Martin.
Introduction
Sport-related injuries are the fourth leading cause of traumatic spinal cord injury (SCI) in the United States, and are particularly prevalent in the 16- to 30-year-old age group.1 Previously considered an untreatable condition, the chance for meaningful recovery after SCI has improved dramatically because of the discovery of numerous treatments and management strategies. This chapter summarizes the current best practices in management of acute SCI and highlights several emerging therapies that are candidates for clinical translation.
Pathophysiology of Spinal Cord Injury
Before discussing potential therapeutic agents for SCI, it is important to first elaborate on the pathophysiology of specific injury mechanisms. The biologic processes in traumatic SCI can be divided into primary and secondary injury followed by regeneration and functional recovery. Primary injury describes the immediate cellular and extracellular damage incurred by destructive forces and energy transfer. Secondary injury involves a cascade of mechanisms beginning immediately and lasting for weeks, including ischemia, vasospasm, thrombosis, inflammatory cytokines, breakdown of the blood–brain barrier, ion-mediated cellular damage, glutamate-related excitotoxicity, oxidative cellular damage, peroxidation of membrane lipids, sodium- and calcium-mediated cell injury, and apoptosis (Figure 5-1).2 The remaining spinal cord tissue bridges are precarious, and therefore secondary injury can be exacerbated by extrinsic factors such as mechanical instability that allows for repetitive trauma and systemic factors such as hypoxia, hypotension, and metabolic derangements that further injure the compromised tissue. After the acute phase, the spinal cord subsequently undergoes a period of limited repair, which is stimulated by cellular signaling and includes resolution of edema, remodeling of the disrupted blood–spinal cord barrier, axonal sprouting, remyelination, and reconnection of synapses (synaptic plasticity). However, this period of repair can also be negatively affected by the formation of a glial scar at the injury site, which hinders neural regeneration. Each of the individual mechanisms occurring at each stage after injury presents a potential target for intervention, and many such therapeutic agents are currently in various stages of research.
Neuroprotection
The primary goal of current SCI management is avoidance of additional injury, which involves a number of different strategies that fall under the category of neuroprotection. Preservation of viable spinal cord tissue is enhanced by careful avoidance of repeat trauma, support of hemodynamic function, timely spinal cord decompression, and focused regulation of the inflammatory response.
Supportive Measures
The optimal management of SCI relies on supportive measures provided by all types of health professionals, beginning in the field with first responders who identify a
potential injury, correctly immobilize the spine, and carefully transport the patient to a specialized trauma center. It is the responsibility of the trauma team to quickly assess and prioritize all injuries while maintaining spinal alignment and hemodynamic stability. The spinal surgeon should be involved in this process as early as possible to perform a complete physical examination, help guide imaging investigations, and lead clinical decision making. Expedient transfer to an intensive care unit (ICU) with respiratory, cardiac, and hemodynamic monitoring is critical because it has been shown to improve morbidity, mortality, and neurologic outcomes.3 Levi et al4 established in 1993 that hypotension caused by neurogenic shock is common in patients with SCI (primarily in cervical, motor-complete injuries), and prompt and aggressive treatment appeared to improve mortality and neurologic outcomes. A subsequent observational study of 77 SCI patients by Vale et al5 suggested that maintaining a mean
arterial pressure (MAP) above 85 mm Hg with crystalloids, or vasopressors, or both for 7 after following injury showed markedly improved neurologic outcomes compared with historical control participants, leading to the American Association of Neurological Surgeons (AANS) and the Congress of Neurological Surgeons (CNS) guideline of maintaining MAP at 85 to 90 mm Hg for 7 days.2
potential injury, correctly immobilize the spine, and carefully transport the patient to a specialized trauma center. It is the responsibility of the trauma team to quickly assess and prioritize all injuries while maintaining spinal alignment and hemodynamic stability. The spinal surgeon should be involved in this process as early as possible to perform a complete physical examination, help guide imaging investigations, and lead clinical decision making. Expedient transfer to an intensive care unit (ICU) with respiratory, cardiac, and hemodynamic monitoring is critical because it has been shown to improve morbidity, mortality, and neurologic outcomes.3 Levi et al4 established in 1993 that hypotension caused by neurogenic shock is common in patients with SCI (primarily in cervical, motor-complete injuries), and prompt and aggressive treatment appeared to improve mortality and neurologic outcomes. A subsequent observational study of 77 SCI patients by Vale et al5 suggested that maintaining a mean
arterial pressure (MAP) above 85 mm Hg with crystalloids, or vasopressors, or both for 7 after following injury showed markedly improved neurologic outcomes compared with historical control participants, leading to the American Association of Neurological Surgeons (AANS) and the Congress of Neurological Surgeons (CNS) guideline of maintaining MAP at 85 to 90 mm Hg for 7 days.2
![]() FIGURE 5-1 A, Primary and secondary mechanisms of injury determining the final extent of spinal cord damage. The primary injury event starts a pathobiological cascade of secondary injury mechanisms that unfold in different phases within seconds of the primary trauma and continuing for several weeks thereafter. B, Longitudinal section of the spinal cord after injury. The epicenter of the injury progressively expands after the primary trauma as a consequence of secondary injury events. This expansion causes an increased region of tissue cavitation and, ultimately, worsened long-term outcomes. Within and adjacent to the injury epicenter are severed and demyelinated axons. The neuroprotective agents listed act to subvert specific secondary injuries and prevent neural damage, and the neuroregenerative agents act to promote axonal regrowth after damage has occurred. ATP = adenosine triphosphate. (Reprinted from Wilson JR, Forgione N, Fehlings MG: Emerging therapies for acute traumatic spinal cord injury. Canadian Medical Association Journal 2013;185(6):485–492. © Canadian Medical Association (2013). This work is protected by copyright and the making of this copy was with the permission of the Canadian Medical Association Journal (www.cmaj.ca) and Access Copyright. Any alteration of its content or further copying in any form whatsoever is strictly prohibited unless otherwise permitted by law.) |
Early Decompression of the Spinal Cord
After SCI, the spinal cord frequently faces ongoing mechanical compression, causing focal ischemia. Decompression may involve anterior or posterior surgery (or a combined approach) to remove tissues such as bone, disk, and blood that are compressing the spinal cord, often followed by an instrumented reconstruction with metallic implants such as screws, hooks, rods, or plates. Alternatively, in cases of dislocations of the spine, decompression may involve a closed-reduction maneuver under cervical traction. Closed reduction has been established as a safe intervention in awake patients who do not have additional rostral injuries, and this treatment is effective in approximately 80% of cases to relieve spinal cord compression and restore alignment.6
The issue of the optimal timing of spinal cord decompression has been debated within the surgical community because of concerns of the risk of surgery and an antiquated notion of the futility of surgery. However, recent evidence has provided clear justification that spinal cord decompression should be performed as quickly as possible,7,8 which is consistent with previous evidence from animal studies.9 A prospective study titled Surgical Timing in Acute Spinal Cord Injury Study (STASCIS) showed that patients undergoing surgery within 24 hours of injury have improved outcomes compared with those undergoing decompression after 24 hours.10 More specifically, the early group (mean time to decompression, 14.2 hours) was 2.8 times more likely to achieve a 2-grade American Spinal Injury Assocation Impairment Scale (AIS) improvement compared with the late group (mean, 48.3 hours). STASCIS also confirmed the safety of early surgery, with equivalent complication rates between early versus late decompression (24% vs. 30%; P = 0.21). Two other observational studies have also been conducted in large cohorts, confirming the benefits of early surgery, although one study found no benefit in the subgroup of AIS A patients.7,8 A European multicenter study, spinal cord injury-prospective, observational European multicenter study (SCI-POEM), is now underway, with similar methodology to STASCIS.11 Considering the compelling evidence in favor of early surgical decompression, early SCI should be performed as quickly as medically and logistically feasible.
Induced Hypothermia
The use of induced hypothermia dates back to ancient Greece, where physicians treated traumatic brain injuries (TBIs) by cooling with ice baths.12 The use of induced hypothermia was cast into the media spotlight in 2007 when a professional football player was treated with systemic hypothermia after sustaining a cervical SCI, recovering the ability to walk several months later.13 Reports are unclear if the initial injury was truly motor complete or incomplete (AIS B or C), but his final neurologic status appears to be a highly functional AIS D. Moreover, it is unclear how much of the recovery was related to the treatment with induced hypothermia, early surgical decompression, intensive rehabilitation, or spontaneous recovery. However, related research in animal models of SCI has demonstrated a substantial benefit with intravascular cooling to a level of moderate hypothermia (32°–34°C), attenuating some of the mechanisms of secondary injury.14 Cooling of the spinal cord has also been used in humans, with intraoperative application of cold saline directly over the spinal cord, but no benefits were established, and the practice has been largely abandoned.15 Levi et al16 recently reported on a pilot study of systemic hypothermia in the acute management of 14 AIS A patients, which demonstrated the safety of this treatment with equivalent complication rates and a trend toward neurologic improvement (43% vs. 21%). However, further research is needed before this treatment is widely adopted, prompting the initiation of a phase II study to provide further evidence.17
Neuroprotective Pharmaceutical Agents
A large number of potential neuroprotective drugs have been investigated for numerous neurologic disorders, including stroke, Alzheimer disease, amyotrophic lateral sclerosis (ALS), TBI, and SCI. Unfortunately, only a small number of these have demonstrated therapeutic benefits, and further development is needed to identify agents with greater efficacy. The mechanisms of action of neuroprotective agents are often nonspecific, offering the possibility of using one compound to treat multiple diseases. In conditions with an acute onset, such as SCI, TBI, and stroke, the efficacy of neuroprotective treatments tends to strongly depend on the timing of administration, posing a substantial barrier to implement successful clinical trials. Furthermore, enrolment in a clinical trial requires that a diagnosis can be confidently made and consent obtained
before administration of the treatment, which can be time consuming and render the treatment ineffective. The design of SCI clinical trials has evolved to overcome these barriers, with many trials including a strict time window (treatment within 12 or 24 hours) and having dedicated research personnel quickly obtain consent and perform treatment administration immediately on arrival to the hospital. The field of SCI research is vast, and a large number of neuroprotective medications have been the subject of human or animal studies. The following sections focus on only the most promising neuroprotective pharmaceutical agents.
before administration of the treatment, which can be time consuming and render the treatment ineffective. The design of SCI clinical trials has evolved to overcome these barriers, with many trials including a strict time window (treatment within 12 or 24 hours) and having dedicated research personnel quickly obtain consent and perform treatment administration immediately on arrival to the hospital. The field of SCI research is vast, and a large number of neuroprotective medications have been the subject of human or animal studies. The following sections focus on only the most promising neuroprotective pharmaceutical agents.
Methylprednisolone
Methylprednisolone (MP) is available as an intravenous (IV) infusion and is among the most potent corticosteroids, inhibiting inflammation and membrane lipid peroxidation. MP is the most studied neuroprotective agent for SCI and was previously used widely because animal studies have shown strong benefits and the National Acute Spinal Cord Injury Study (NASCIS) 2 clinical trial demonstrated modest benefits in humans. However, MP has been the subject of substantial debate and controversy, including the fact that the 2002 AANS/CNS guidelines suggested MP as a “treatment option,” but the more recent 2013 version of the guidelines reversed this to “treatment not recommended” without substantial new evidence. In fact, a recently published Cochrane review synthesized the literature on MP, including six randomized controlled trials (RCTs) and several observational studies, and suggested that MP had evidence of efficacy and should remain a treatment option.2,18 This review included a meta-analysis that demonstrated no neurologic benefit in the overall data but a 4-point improvement on American Spinal Injury Association (ASIA) motor score when initiated within 8 hours. The analysis also identified a trend toward decreased mortality rates with MP but increased rates of wound infection and gastrointestinal bleeding. Critics of MP have argued that there is clear evidence of increased complications, but the improved efficacy is only seen in subgroup analysis, as was performed in the Cochrane review and in the original publication of NASCIS 2 results.2 However, it is important to note that the decision to perform subgroup analysis with the 8-hour time window in NASCIS 2 was made a priori rather than post hoc. It is also notable that the STASCIS data showed a 44% reduction in overall complications among patients who received MP, likely because the population was confined to cervical injuries, and wound infections are more common at lower levels.10 Furthermore, it is increasingly being recognized that cervical SCI has a greater potential for recovery than thoracolumbar injuries, indicating that future studies will be better powered by including only the cervical injury population.19 In light of the evidence from the Cochrane review, which comprises the highest level of the evidentiary pyramid, MP should remain a treatment option because it most likely offers a small neurologic benefit with modest additional risks. The optimal candidates for MP are young, healthy patients with cervical injuries treated within 8 hours who will undergo early surgical decompression and stabilization within 24 hours, based on work from the STASCIS trial.19 However, it must be stressed that this is an ongoing controversy within the SCI community, and SCI groups other than the AANS/CNS are likely to make differing recommendations regarding the use of MP.
Riluzole
Riluzole is a voltage-gated sodium channel blocker that reduces aspects of secondary injury, such as glutamatergic excitotoxicity and astrocytosis.20 This drug was initially developed for treatment of ALS, receiving regulatory approval in the 1990s. Riluzole slows the degeneration of motor neurons and prolongs survival in ALS patients, albeit with a mean improvement of only 2 to 3 months in this typically aggressive disease.21 In animal models of SCI, riluzole attenuates the secondary injury cascade and improves behavioral outcomes such as locomotion.20 Recently, a phase I/II clinical study in SCI that treated 36 patients (AIS A, B, or C, 28 cervical and 8 thoracic) with riluzole showed an improvement of 15.5 points on the ASIA motor score compared with matched registry control participants (P = 0.02).22 The multicenter phase II/III RCT Riluzole in SCI Study (RISCIS) is now underway in several countries around the world, only enrolling patients with cervical SCI.23
Minocycline
Minocycline is a derivative of the acne medication tetracycline, possessing antibiotic and anti-inflammatory properties and having an established safety profile. It appears to have multiple mechanisms of action in SCI, including the inhibition of microglial activation, tumor necrosis factor α (TNF-α), nitric oxide synthase (NOS), and metalloproteinases.24,25 Preclinical research has demonstrated benefits of minocycline in SCI, with improved motor function, reduced lesion size, and a greater preservation of axons.24,25 A small single-center RCT of minocycline versus placebo in 44 patients with
cervical or thoracic SCI showed a weak trend toward improvement on ASIA motor scores (6 points; P = 0.20).26 However, it was noted that the cervical subgroup of 25 patients demonstrated substantially greater improvement (14 points; P = 0.05).26 These results have prompted a multicenter phase III RCT titled Minocycline in Acute Spinal Cord Injury (MASC), which is now underway and is also focused solely on the cervical injury population.27
cervical or thoracic SCI showed a weak trend toward improvement on ASIA motor scores (6 points; P = 0.20).26 However, it was noted that the cervical subgroup of 25 patients demonstrated substantially greater improvement (14 points; P = 0.05).26 These results have prompted a multicenter phase III RCT titled Minocycline in Acute Spinal Cord Injury (MASC), which is now underway and is also focused solely on the cervical injury population.27
Granulocyte Colony-Stimulating Factor
Granulocyte colony-stimulating factor (G-CSF) is an endogenous hormone and cytokine best known for its role in inducing the mobilization of stem cells from bone marrow into the blood. Preclinical studies have also identified several nonhematopoietic functions of G-CSF, offering a neuroprotective effect in both SCI and stroke that appears to preserve myelin, promote angiogenesis, and attract stem cells to the injury site while also inhibiting TNF-α and interleukin-1 (IL-1).28 A pilot study using IV injection of G-CSF in 16 human participants demonstrated safety and tremendous efficacy, with all 16 patients showing improvement in AIS grade.29 A subsequent multicenter nonrandomized controlled study also showed intriguing results, with 15 of 17 participants receiving G-CSF improving at least one AIS grade.30 Further research of this promising therapeutic agent is anticipated, but a larger scale trial has yet to be announced.
Inhibitors of Glutamate-Related Excitotoxicity
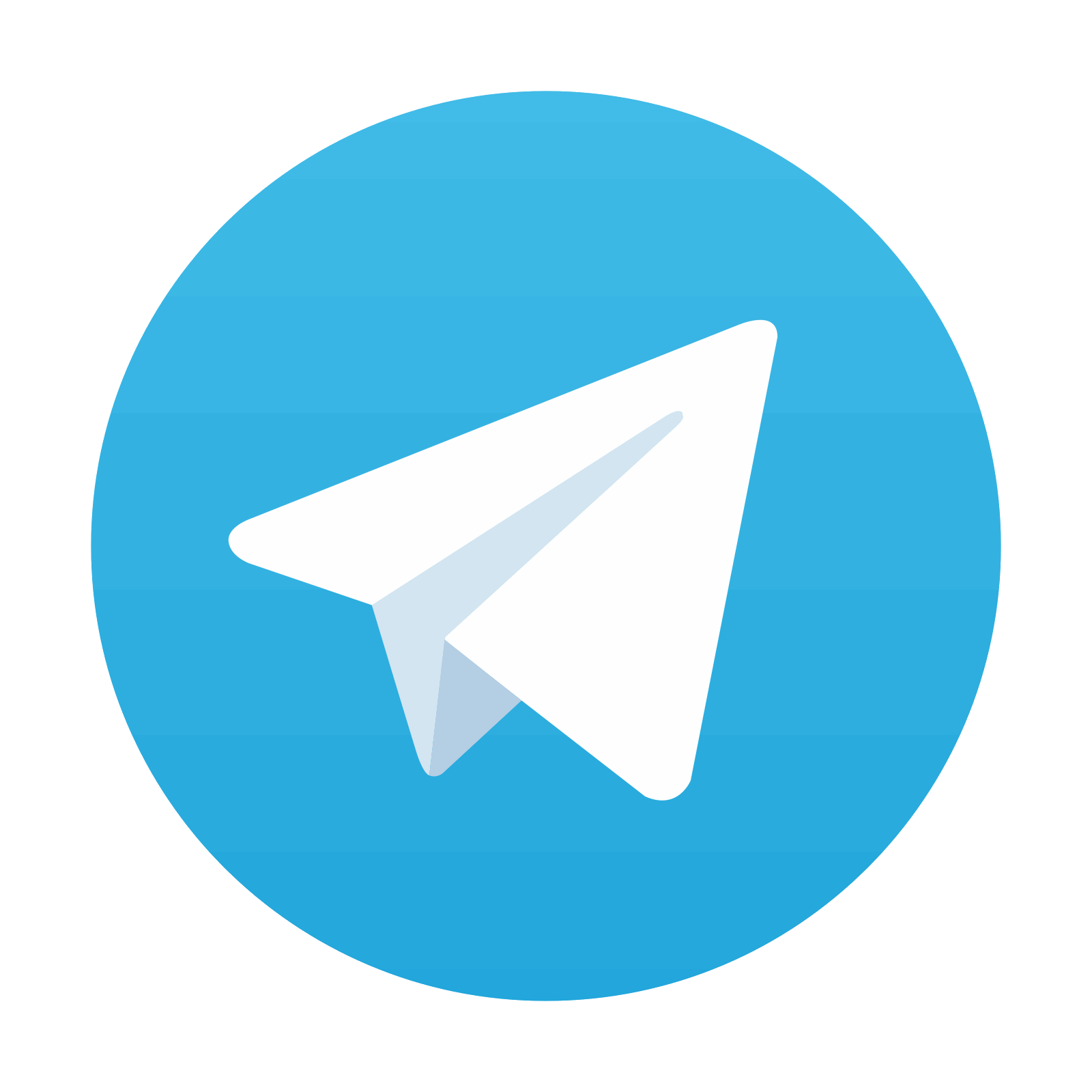
Stay updated, free articles. Join our Telegram channel

Full access? Get Clinical Tree
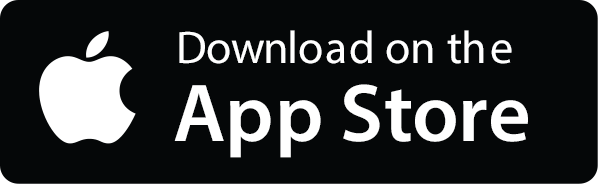
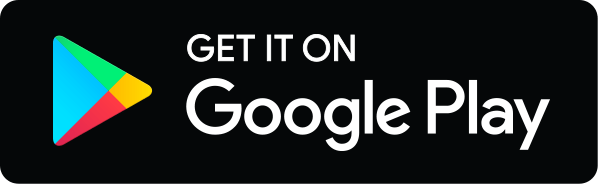