Spinal Biomechanics
Jordan M. Glaser
Sheeraz A. Qureshi
Introduction to Spinal Biomechanics
The concept of biomechanics is generated from the combination of information arising from the biologic sciences and engineering mechanics. The interplay of anatomy (form and function) with structural and mechanical properties provides understanding of the natural behavior and limitations of the structure in question.
Regarding the spinal column, biologic materials include bone, cartilage, and intervertebral disk. These materials are arranged in a specific pattern to ultimately create a composite structure that is a load-bearing and load-transmitting protective conduit. This macrostructure is divided into motion segments which include two vertebrae and the intervertebral disk in between. This chapter serves to define and present basic mechanical concepts, consider the physical characteristics of the spine which influence function, apply mechanical principles to the structure of the spine (the individual motion segment and the composite structure), and evaluate the potential effect of surgical intervention and instrumentation on spinal mechanics.
The Spinal Motion Segment
Regarding individual motion segments and the spine as a whole, motion is interpreted based upon the natural anatomic limitations defined by anatomic design. Forces applied and resisted must be measured against tissue tolerance of the anatomic materials comprising the complex structure of the spine. Application of the global coordinate system of the body provides planes of reference to describe movement and direction of applied forces. The origin of this coordinate system (which defines three planes—sagittal, coronal, and axial or horizontal; and potential rotation about three axes—x, y, and z, respectively) is located at the base of the spine. Therefore, flexion and extension are defined in the sagittal plane and occur about the x-axis, lateral bending in the coronal plane about the y-axis, and rotation or twisting in the axial or horizontal plane about the z-axis. Force and moments can therefore be applied to the spine in any of these planes about any of these axes as well as combinations thereof, indicating the potential for the development of complex patterns of force vectors across any single motion segment. Forces occurring along the z-axis include compression and tension and may either compress the structures of the motion segment or stretch them. These structures potentially include the bony anatomy (vertebral body, pars, or facet joint surfaces), the intervertebral disks, or facet capsules. These forces may typically be generated by lifting a heavy object. Compressive loading can produce elevated intradiscal pressure within the nucleus pulposus, thereby placing the annulus fibrosus under tension and potentially causing vertebral end plate deformation. Tension through the motion segment produces the opposite effect by lengthening all of the fibers throughout the disk in the direction of the applied force.
Shear forces may also be experienced by the motion segment. These forces may typically be generated by pushing or pulling activities, during which the force vector applied is perpendicular to the end plate or the disk/end plate interface, directed along the y-axis. Shear can also occur along a laterally directed vector as well along the x-axis. Shear forces produce tension along the fibers of the annulus fibrosus oriented in the direction of the force applied. Torsion or twisting also produces tension in the fibers of the annulus lengthened by the applied movement.
In addition to potentially producing shear forces, torsion, twisting, or bending around an axis also produces a moment of force. Rotation about the x-axis would produce a flexion or extension moment, while rotation about the y-axis produces a lateral bending moment. Rotation about the z-axis produces a torsional moment resulting in a twisting of the spine. Location of this axis of rotation as it relates to the anatomy may have a profound effect on generated motion as well as potential injury of involved structures.
An understanding of the concept of location of axis of rotation helps to clarify the variability of normal motion segment kinematics as well as the mechanism of a traumatic injury or effect of degenerative changes.
For example, regarding flexion and extension of a lumbar motion segment, the normal axis of rotation on the x-axis is located typically at the center of the intervertebral disk. Flexion and extension shift the location of this rotational axis slightly anteriorly with flexion and slightly posteriorly with extension based on the normal coupling of flexion and extension with small translational movements along the y-axis. Traumatic injury may be the result of dramatic flexion or extension beyond the anatomic limits of the spinal motion segment imparted by tissue factors of bone, ligament, and muscle and anatomic configuration. By applying the concept of axis of rotation we can generate an understanding of the injury pattern that would be produced depending on the location of this axis by the inciting trauma. If the axis is located at the center of the disk and the mechanical structural limits are surpassed by the trauma in flexion, the anterior aspect of the vertebral bodies and disk will be loaded in compression while the posterior elements will undergo distraction and produce a tension force. This movement pattern can then lead to compression or burst fracture patterns. If the location of the axis of rotation is located at the anterior aspect of the annulus, it can be understood that the structures posterior to this axis will experience tension as a result of the distraction produced by flexion at the motion segment. In this scenario, tissues such as the disk, would fail under tension and vertebral bodies would rotate from one another while posterior elements would rotate and be distracted from one another. If the axis of rotation was placed anterior to the spine itself and vertebral bodies in the motion segment were flexed about this axis, a potential flexion distraction injury would occur in which anterior and posterior elements of a given vertebra would be rotated and distracted from the adjacent vertebra, producing a flexion/distraction injury due to failure under tension (Figs. 38.1 and 38.2).
For example, regarding flexion and extension of a lumbar motion segment, the normal axis of rotation on the x-axis is located typically at the center of the intervertebral disk. Flexion and extension shift the location of this rotational axis slightly anteriorly with flexion and slightly posteriorly with extension based on the normal coupling of flexion and extension with small translational movements along the y-axis. Traumatic injury may be the result of dramatic flexion or extension beyond the anatomic limits of the spinal motion segment imparted by tissue factors of bone, ligament, and muscle and anatomic configuration. By applying the concept of axis of rotation we can generate an understanding of the injury pattern that would be produced depending on the location of this axis by the inciting trauma. If the axis is located at the center of the disk and the mechanical structural limits are surpassed by the trauma in flexion, the anterior aspect of the vertebral bodies and disk will be loaded in compression while the posterior elements will undergo distraction and produce a tension force. This movement pattern can then lead to compression or burst fracture patterns. If the location of the axis of rotation is located at the anterior aspect of the annulus, it can be understood that the structures posterior to this axis will experience tension as a result of the distraction produced by flexion at the motion segment. In this scenario, tissues such as the disk, would fail under tension and vertebral bodies would rotate from one another while posterior elements would rotate and be distracted from one another. If the axis of rotation was placed anterior to the spine itself and vertebral bodies in the motion segment were flexed about this axis, a potential flexion distraction injury would occur in which anterior and posterior elements of a given vertebra would be rotated and distracted from the adjacent vertebra, producing a flexion/distraction injury due to failure under tension (Figs. 38.1 and 38.2).
The effect of degenerative changes of the intervertebral disk and loss of disk height also may affect the location of the axis of rotation in flexion and extension. Disk degeneration can produce differences in spine loading and movement characteristics of the motion segment. It has been reported that the axis of rotation migrates posteriorly toward the facet joints to a greater degree than is seen without disk degeneration during extension, thereby leading to increased loading of the posterior elements.
Spinal Motion Segment Tolerance
Limitations related to tissue or material strength, anatomic design, applied force, and direction of force have been studied in cadaveric models as they apply to specific segments of the spine. These limitations produce a characteristic load tolerance of the spinal structure to an applied force or moment of force. However, an obvious limitation to the study of tissue characteristics in cadaveric specimens is that the tissue does not have the capacity to adapt or increase tolerance as live tissue would. Also, in vitro tissue preparation and processing techniques may affect its material properties. An understanding of the relative strengths of these tissues as they are tested by moments in varied and coupled motions sheds additional light on the potential mechanism of injury as related to biomechanics.
Muscle tolerance has been estimated at 32 MPa, significantly lower than that of tendon, estimated between 60 MPa and 100 MPa. This difference indicates why muscle is likely to fail at a significantly lower load than healthy tendon. Ultimate ligament stress has been estimated at 20 MPa, while ultimate bone stress has been determined to depend greatly on the direction of the applied force. It can be noted that spinal ligament tolerance generally varies by region of the spine, increasing incrementally in the caudal direction, although there are exceptions. Tolerance of bone has been found to range from 51 MPa in the transverse plane to 190 MPa under longitudinal compression.
Regarding compression tolerance specifically, as it applies, to the spinal motion segment, it has been determined that the end plate is the “weak point of the system” and its resistance to load is both age and gender dependent (the end plate is more susceptible to damage
in older, female individuals). End plate tolerance in females is typically 2 kN less than that in males. Based on collected data reviewed by Jager et al., the magnitude of force necessary for end plate failure follows a normal distribution ranging from 2,000 N to 14,000 N. End plate strength increases in each of the caudal vertebral segments moving distally in the lumbar spine by 3 kN per lumbar level. The cephalad end plate of the vertebral body is typically damaged rather than the caudal. This damage is thought to be initiated by a compressive force directed caudally by the nucleus pulposus above. This theory is supported by data compiled by Jager et al. indicating that the strength of the vertebrae is approximately 8 kN lower than the disk. Damage generally occurs to either the end plate itself or the supportive adjacent trabecular bone supporting the end plate. It is even possible at this point for disk material to herniate into the vertebral body and eventually calcify, creating the radiographic feature known as a Schmorl node. Compression of the end plate into the vertebral body in turn decompresses the nucleus pulposus, creating more space and compromising the nucleus’s capacity to then resist compressive loads. In turn, additional compressive loads are borne by the annulus, rather than the force transmitted outwardly to create hoop stress resisted by the annulus.
in older, female individuals). End plate tolerance in females is typically 2 kN less than that in males. Based on collected data reviewed by Jager et al., the magnitude of force necessary for end plate failure follows a normal distribution ranging from 2,000 N to 14,000 N. End plate strength increases in each of the caudal vertebral segments moving distally in the lumbar spine by 3 kN per lumbar level. The cephalad end plate of the vertebral body is typically damaged rather than the caudal. This damage is thought to be initiated by a compressive force directed caudally by the nucleus pulposus above. This theory is supported by data compiled by Jager et al. indicating that the strength of the vertebrae is approximately 8 kN lower than the disk. Damage generally occurs to either the end plate itself or the supportive adjacent trabecular bone supporting the end plate. It is even possible at this point for disk material to herniate into the vertebral body and eventually calcify, creating the radiographic feature known as a Schmorl node. Compression of the end plate into the vertebral body in turn decompresses the nucleus pulposus, creating more space and compromising the nucleus’s capacity to then resist compressive loads. In turn, additional compressive loads are borne by the annulus, rather than the force transmitted outwardly to create hoop stress resisted by the annulus.
With flexion and extension of the lumbar spine, different structures are responsible for compressive force resistance. Adams et al. reported that 60% to 70% of applied forces are resisted by the neural arch (posterior elements) in extension. The anterior longitudinal ligament and disk offer resistance to extension and the zygapophyseal joints and interspinous ligament may be the first structures susceptible to damage secondary to compressive load. Adams et al. reported that flexion can lead to structural injury with applied moments ranging from 50 to 80 Nm with 5 to 9 degrees of angulation in the upper lumbar spine and 10 to 16 degrees per segment in the lower lumbar spine. The first structures to apparently fail in flexion were found to include the supraspinous and interspinous ligaments.
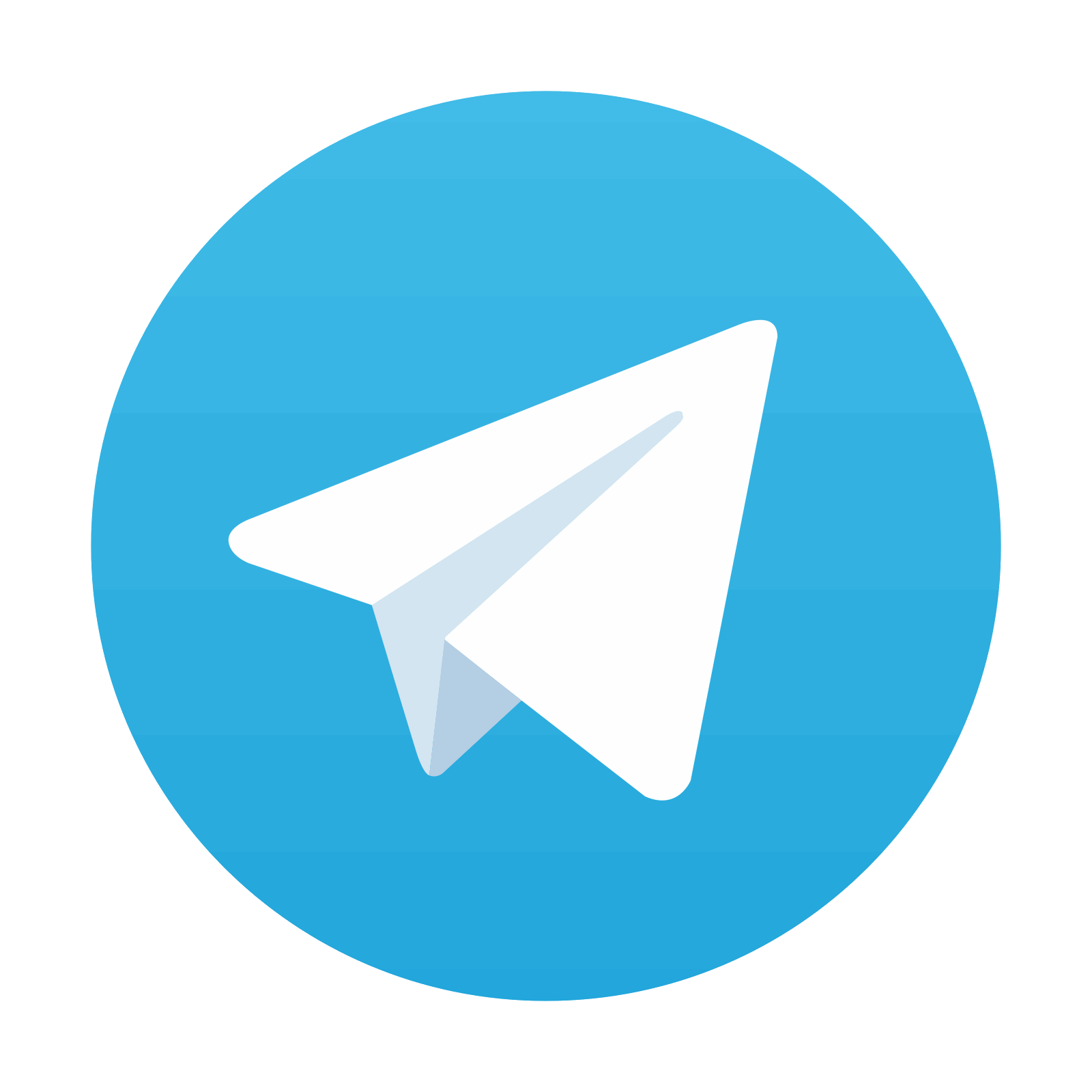
Stay updated, free articles. Join our Telegram channel

Full access? Get Clinical Tree
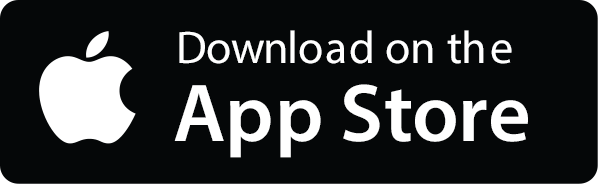
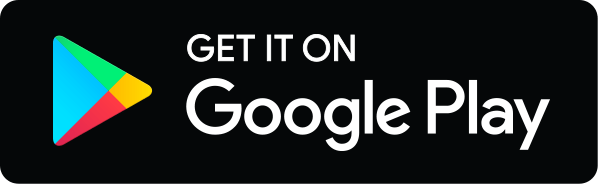