Chapter objectives
- •
Incorporate biomechanical principles of the shoulder as they relate to prevention and postinjury or postsurgical rehabilitation for specific injuries.
- •
Associate anatomic structures of the shoulder to particular injuries based on function of the structures during the pitching act.
- •
Explain the role of the rotator cuff in shoulder arthrokinematics and prevention of injury.
- •
Develop a rehabilitation program for specific pathologic shoulder conditions that takes into account the biomechanical function and healing parameters for the anatomic structures involved.
- •
Advance an athlete through phases of shoulder rehabilitation based on specific criteria for progression.
- •
Incorporate rehabilitation limitations and concerns for specific postinjury and postsurgical pathologic shoulder conditions.
Most athletic shoulder injuries are due to one of two mechanisms: (1) repetitive overhead activity (microtrauma) or (2) a significant force (macrotrauma) applied to the shoulder complex. The violent act of throwing and other similar overhead movements results in the repeated application of high stress to both the shoulder and the elbow. Most of these injuries can be classified as microtraumatic and result from repetitive overuse mechanisms. Tullos and King reported that at least 50% of all baseball players experience sufficient shoulder or elbow joint symptoms to keep them from throwing for varying periods during their careers. Conte et al reported that 28% of all injuries sustained by professional baseball pitchers occurred in the shoulder joint. McFarland and Wasik found that upper extremity injuries in collegiate baseball players accounted for 75% of the time lost from the sport because of injury, with the pitcher being the most frequently injured player (69%). The most common injury cited was rotator cuff tendinitis. It has been reported that shoulder injuries are more common in pitchers than in position players. The synchronous kinematics of throwing can be influenced by a number of factors, including glenohumeral and scapulothoracic motion, connective tissue flexibility, osseous structure, and dynamic muscle balance and symmetry. The glenohumeral joint complex is also susceptible to traumatic injuries such as dislocations, subluxations, acromioclavicular joint sprains, soft tissue injuries, and other types of injuries that commonly occur during collision and contact sports. The shoulder region is further predisposed to athletic injury because the tremendous mobility afforded by the joint is the result of inherently poor glenohumeral stability. In this chapter we discuss the anatomy, biomechanics, common injuries, and rehabilitation programs specific to the shoulder joint complex.
Anatomy and biomechanics
The shoulder complex is composed of three synovial joints and one physiologic articulation. The sternoclavicular, acromioclavicular, and glenohumeral components are true joints, whereas the physiologic articulation is usually referred to as the scapulothoracic joint. These four articulations, along with the ligaments ( Fig. 12-1 ), rotator cuff complex, and the primary mover musculature of the upper quarter, work in unison to produce and control the various movements of the shoulder complex. Dysfunction in any of these interdependent structures can result in limited performance of the entire shoulder complex.

Sternoclavicular Joint
Functionally, the sternoclavicular joint is the only bony articulation connecting the shoulder complex to the thorax. It is a modified saddle joint with a joint capsule, three major ligaments, and an intraarticular disk. The costoclavicular ligament is the primary stabilizer of the joint. It stabilizes the clavicle against the pull of the sternocleidomastoid muscle and controls motion about the joint that produces elevation-depression and protraction-retraction. The costoclavicular ligament also functions to check elevation of the clavicle. The sternoclavicular ligament stabilizes the joint anteriorly and posteriorly by controlling anterior and posterior movements of the clavicular head on the sternum. The proximal end of the clavicle is separated from the sternal manubrium by an intraarticular disk or meniscus. This joint disk helps absorb centrally directed forces transmitted along the clavicle and improves the incongruence of the articular surfaces, thus decreasing the tendency of the clavicle to dislocate medially on the manubrium.
Acromioclavicular Joint
The acromioclavicular joint is a plane joint that consists of two major ligament complexes and an intraarticular meniscus. The two primary functions of the acromioclavicular joint are (1) to maintain the appropriate clavicle-scapula relationship during the early stages of upper limb elevation and (2) to allow the scapula additional range of rotation on the thorax in the later stages of limb elevation.
The integrity of the articulation between the acromion and the distal end of the clavicle is maintained by the surrounding ligaments rather than by the bony configuration of the joint. The coracoclavicular ligaments are the stabilizers of the primary acromioclavicular joint. The ligaments consist of a lateral portion, the trapezoid ligament, and a medial portion, the conoid ligament. It is these ligaments that are responsible for controlling the longitudinal rotation of the clavicle that is necessary for full unrestricted upper extremity elevation. The joint capsule is reinforced by the anterior, inferior, posterior, and superior acromioclavicular ligaments. The fibers of the deltoid and upper trapezius muscles, which attach to the superior aspect of the clavicle and acromion process, function to reinforce the acromioclavicular ligaments and further stabilize the joint.
A fall onto an outstretched arm tends to translate the scapula medially, and the small acromioclavicular joint alone cannot adequately control scapular motion, which frequently results in joint dislocation. As the scapula and its coracoid process attempt to move medially, the trapezoid ligament tightens, thereby shifting the force to the clavicle and, ultimately, to the strong sternoclavicular joint. Therefore, anterior-posterior stability of the acromioclavicular joint is maintained by the joint capsule, whereas vertical stability is provided by the coracoclavicular ligaments.
Glenohumeral Joint
The glenohumeral joint is the most mobile and the least stable of all the joints in the human body. It is also the most commonly dislocated major joint in the human body. Even though the glenohumeral joint exhibits significant physiologic motion, only a few millimeters of humeral head displacement occur during any of these movements in normal individuals. Conversely, on clinical examination, Matsen demonstrated excessive passive displacement consisting of 10 mm inferiorly and 8 mm anteriorly in normal asymptomatic shoulders. Therefore, stabilization of the humeral head within the glenoid is accomplished via the combined effort of the static and dynamic glenohumeral stabilizers. These two categories of joint stabilizers can be classified into passive and active stabilizing mechanisms ( Box 12-1 ).
Passive mechanisms
Bony
Glenoid labrum
Intraarticular pressure
Joint cohesion
Glenohumeral capsule
Glenohumeral ligaments
Active mechanisms
Joint compression
Dynamic ligament
Tension
Neuromuscular control
Scapulothoracic joint
The Static Stabilizers: Bony Geometry
The bony geometry of the glenohumeral joint sacrifices osseous stability to facilitate excessive joint mobility. The articular surface of the glenoid is pear-shaped, with the inferior half being 20% larger than the superior half. Additionally, the articular surface of the glenoid is much smaller than the articular surface of the humeral head, which is approximately three to four times that of the glenoid. At any given time during normal motion, only 25% to 30% of the humeral head is actually in contact with the glenoid. This lack of articular contact contributes to the inherent instability of the glenohumeral joint.
The glenoid faces superiorly, anteriorly, and laterally. The superior tilt of the inferior glenoid limits inferior translation of the humeral head on the glenoid. The glenoid articular surface is within 10° of being perpendicular to the blade of the scapula; thus, the glenoid fossa is retroverted approximately 6°. Excessive retroversion of the glenoid is considered a primary cause predisposing an individual to posterior glenohumeral instability, and increased glenoid anteversion is found in individuals suffering from recurrent anterior dislocations.
The areas of contact between the articular surfaces of the humeral head and the glenoid vary depending on arm position, with the greatest amount of articular contact occurring in the midpoint of elevation between 60° and 120°. With increasing arm elevation, contact points on the humeral head move from inferior to posterosuperior while the glenoid contact shifts from a central location posteriorly.
At the extremes of normal motion the glenohumeral joint does not function in a strict ball-and-socket fashion. In these extremes, rotation is coupled with humeral head translation on the glenoid. Normally, external rotation (ER) produces posterior translation and vice versa, whereas subjects with known anterior instability demonstrate anterior humeral head translation in extreme ER.
Bony defects on either the humeral head or the glenoid are commonly associated with glenohumeral instability. With recurrent anterior instability, an osseous defect is commonly noted on the posterolateral portion of the humeral head (Hill-Sachs lesion). In contrast, an anteromedial lesion is often noted with recurrent posterior instability (reverse Hill-Sachs lesion). Bony defects of the anterior or posterior glenoid rim may also occur with recurrent glenohumeral instability. Both these lesions represent impaction fractures and occur as the humeral head subluxates and then reduces repetitively over the glenoid rim.
The Glenoid Labrum
The glenoid labrum is a fibrous rim that serves to slightly deepen the glenoid fossa and allows attachment of the glenohumeral ligaments on the glenoid. The superior attachment of the labrum is loose and approximates the mobility of the meniscus within the knee joint, whereas the inferior attachment is firm and unyielding. The labrum functions to deepen the glenoid anywhere from 2.5 to 5 mm. The labrum may function in tandem with joint compression forces to stabilize the joint in the midrange of glenohumeral motion, where the ligamentous structures are lax. The labrum also serves as a buttress that assists in controlling glenohumeral translation, similar to a chock, which would prevent a wheel from rolling downhill. Finally, Bowen et al noted that the labrum also contributes to glenohumeral joint stability by increasing the surface area and acting as a load-bearing structure for the humeral head. In addition, the long head of the biceps brachii muscle inserts into the superior portion of the labrum. The vascular supply to the labrum arises mostly from its peripheral attachment to the capsule and is derived from a combination of the suprascapular circumflex scapular branch of the subscapular and the posterior circumflex humeral arteries. The anterosuperior labrum appears to generally have a poor blood supply, whereas the inferior labrum exhibits significant blood flow. The vascularity of the labrum decreases with increasing age. No mechanoreceptors have been identified within the glenoid labrum. However, free nerve endings have been isolated in the fibrocartilaginous tissue of the labrum, the biceps-labrum complex, and the connective tissue surrounding the labrum.
Shoulder Capsule and Ligaments
The shoulder joint capsule is large, loose, and redundant, which also allows the large range of glenohumeral motion naturally available at the glenohumeral joint. The capsule is composed of multilayer collagen fiber bundles of differing strength and orientation. The anteroinferior capsule is the thickest and strongest portion of the joint capsule. The collagen fibers within this portion of the joint capsule have two distinct orientations, radial fibers that are linked to each other by circular fibers. In this manner, rotational forces create tension within the fibers that produces compression on the joint surfaces while centering the articular surfaces.
The glenohumeral joint capsule is reinforced with capsular ligaments that contribute greatly to joint stability. The size, strength, and orientation of these capsular ligaments vary widely, and they typically function when the joint is placed in extremes of motion to protect against instability.
The anterior glenohumeral joint capsule consists of three distinct ligaments: the superior glenohumeral ligament, the middle glenohumeral ligament, and the inferior glenohumeral ligament complex. The superior glenohumeral ligament arises from the anterosuperior labrum anterior to the biceps tendon and inserts superiorly into the lesser tuberosity of the humerus. The middle glenohumeral ligament originates adjacent to the superior glenohumeral ligament and extends laterally to attach onto the lesser tuberosity with the subscapularis tendon. The inferior glenohumeral ligament complex is composed of three functional portions: an anterior band, a posterior band, and an axillary pouch. These structures exhibit tremendous variation, with the middle glenohumeral ligament having the greatest degree of variation. Posteriorly, the capsule is the thinnest and has no distinct capsular ligaments except for the posterior band of the inferior glenohumeral ligament complex.
The anterior glenohumeral ligaments function as primary restrains to anterior translation of the humerus on the glenoid. The superior and middle glenohumeral ligaments are the primary restraints to anterior translation with the arm completely abducted. The middle glenohumeral ligament plays a significant role in limiting translation of the humeral head in the midrange of shoulder abduction. The inferior glenohumeral ligament complex, particularly the anterior band, is responsible for preventing translation of the humeral head with the arm abducted to 90° and beyond.
The constraints to posterior translation are also based on arm position. The posteroinferior portion of the inferior glenohumeral ligament complex is the primary passive stabilizer against posterior instability with the arm in 90° of abduction. At less than 90° of abduction, the posterior capsule is the primary restraint against posterior forces.
During the combined motion of abduction and ER, the anterior band of the inferior glenohumeral ligament complex fans out and surrounds the anteroinferior aspect of the humeral head like a hammock restraining anterior displacement while the posterior band provides inferior support. During abduction and internal rotation (IR), the anterior band of the inferior glenohumeral ligament complex moves inferiorly to resist inferior displacement, and the posterior band shifts posterosuperiorly to prevent posterior translation. Additionally, when the arm is positioned in 90° of abduction and 30° of extension, the anterior band of the inferior glenohumeral ligament complex becomes the primary stabilizer to both anterior and posterior forces. Finally, as a general rule, the superior capsular structures play significant roles in glenohumeral joint stability when the arm is adducted, whereas the inferior structures are the primary providers of joint stability between 90° of abduction and full elevation.
The forces required to dislocate the shoulder change with age. Less force is required in individuals younger than 20 and older than 40 years of age. Bankart described the “essential lesion” responsible for shoulder instability as detachment of the labrum and capsule from the glenoid (referred to subsequently as a Bankart lesion). It is interesting to note that a Bankart lesion is not one specific anatomic defect, but rather a wide spectrum of pathologic conditions related to detachment of the capsulolabral complex of the glenohumeral joint. Baker et al identified three types of Bankart lesions initially caused by acute anterior glenohumeral dislocation ( Table 12-1 ). It is also interesting to note that the degree of instability present during examination under anesthesia varies depending on the grade of the lesion ( Table 12-1 ). Approximately 85% of patients with traumatic anterior glenohumeral dislocations exhibit detachment of the glenoid labrum from the anterior glenoid rim, whereas the remaining individuals show interstitial stretch or rupture of the capsule without loosening or detachment of the labrum.
Grade | Definition | Instability Present Under Anesthesia |
---|---|---|
I | Capsular tears without labral lesions | Stable |
II | Capsular tears with partial labral detachments | Mildly unstable |
III | Complete capsular-labral detachments | Grossly unstable |
Intraarticular Pressure and Joint Cohesion
Normally, the capsule of the glenohumeral joint is sealed airtight and contains very little (less than 1 mL) fluid. This limited fluid volume contributes to joint stability in a manner similar to syringe-type suction and serves to hold the articular surfaces together with viscous and intermolecular forces. The normal intraarticular pressure of the glenohumeral joint is negative, which creates a relative vacuum that also helps resist translation. This force is relatively small, with only approximately 20 to 30 lb of stabilizing pressure being exerted, but when these properties are disrupted via capsular puncture or tear, subluxation tends to occur. In an unstable shoulder this vacuum effect of viscous and intermolecular forces is lost because the labrum is no longer able to function as a seal or gasket.
The Dynamic Stabilizers: Neuromuscular Control
The primary active stabilizers of the glenohumeral joint and the secondary stabilizers are listed in Box 12-2 . The most important function that the primary glenohumeral stabilizers provide is the production of a combined muscular contraction that enhances humeral head stability during active arm movements. These muscles act together in an agonist/antagonist relationship to both effect movement of the arm and at the same time stabilize the glenohumeral joint. The combined effect of the rotator cuff musculature is a synergistic action that creates humeral head compression within the glenoid and counterbalances the shearing forces generated by the deltoid.
Primary stabilizers
Rotator cuff muscles (supraspinatus, infraspinatus, teres minor, subscapularis)
Deltoid
Long head of the biceps brachii
Secondary stabilizers
Teres major
Latissimi dorsi
Pectoralis major
The second method of active glenohumeral joint stability is provided through blending of the rotator cuff tendons in the shoulder capsule, which produces tension with the capsular ligaments. This tension serves to actively tighten the glenohumeral ligamentous capsule and thereby accentuates centering of the humeral head within the glenoid fossa.
The third component that contributes to dynamic shoulder stability is neuromuscular control. This refers to the continuous interplay of afferent input and efferent output in an individual’s awareness of joint position (proprioception) and the ability to produce a voluntary muscular contraction to stabilize the joint or alter joint position so that excessive humeral head translation can be prevented. The ability to control the shoulder joint during active motion is referred to as reactive neuromuscular control . This factor is more important to normal shoulder function than joint position or repositioning ability. Reactive neuromuscular control is an individual’s ability to integrate proprioceptive information and motor control to react to the information.
Scapulothoracic Joint
The scapulothoracic joint is not a true anatomic joint because it has none of the usual joint characteristics, such as a joint capsule. However, it is a free-floating physiologic joint without any ligamentous restraints, except where it pivots about the acromioclavicular joint. According to Steindler, the primary force holding the scapula to the thorax is atmospheric pressure. The ultimate function of scapular motion is to orient the glenoid fossa for optimal contact with the maneuvering arm and to provide a stable base of support for controlled rolling and gliding of the articular surface of the humeral head. This relationship allows optimal function of the upper extremity in space by continually adjusting the length-tension relationships of all vital musculature as the scapula constantly repositions itself on the thoracic wall. The muscles of the scapulothoracic joint play a significant role in maintaining optimal scapular position and posture. Five muscles directly control the scapula: the trapezius (upper, middle, and lower), the rhomboids, the levator scapulae, the serratus anterior, and to a lesser extent, the pectoralis minor. These muscles act in a synchronous fashion to provide both mobility and stability to the scapulothoracic joint.
Coracoacromial Arch
The coracoacromial arch, or subacromial space, has also been considered a physiologic joint by some authors. It provides protection against direct trauma to the subacromial structures and prevents the humeral head from dislocating superiorly. It is bordered by the acromion process and acromioclavicular joint superiorly; the coracoid process anteromedially; and the rotator cuff and greater tuberosity of the humeral head inferiorly. The coracoacromial ligament, which serves as a “roof” over the greater tubercle of the humerus, rotator cuff tendons, portions of the biceps tendon, and the subdeltoid bursa, further decreases the available space within the arch. The space between the humeral head and the inferior aspect of the acromion depends on arm position and varies from approximately 30 ± 4.9 mm (when the arm is at 0° of abduction) and 6 ± 2.4 mm (when the arm is at 90° of abduction). Additionally, this space can be decreased in shoulders with inflamed or swollen soft tissues. Soft tissue structures such as the supraspinatus and infraspinatus tendons, which lie between the two unyielding joint borders, are at risk for impingement or compressive injuries in individuals with abnormal glenohumeral joint mechanics or trauma.
Rotator Cuff
The supraspinatus, infraspinatus, teres minor, and subscapularis muscles make up the rotator cuff ( Fig. 12-2 ). Collectively, each tendon blends with and reinforces the glenohumeral capsule, and all contribute significantly to dynamic stability of the glenohumeral joint. The rotator cuff muscles could be considered the fine tuners of the glenohumeral joint and shoulder girdle, whereas the latissimus dorsi, teres major, deltoid, and pectoralis muscles are the prime movers. All the rotator cuff muscles contribute in some degree to glenohumeral abduction, with the supraspinatus and deltoid muscles functioning as the primary abductors. The rotator cuff muscles also function to compress the glenohumeral joint and act to reduce or control vertical shear imparted onto the humeral head. The infraspinatus is considered the next most active rotator cuff muscle, after the supraspinatus. Selective nerve blocks have shown that the supraspinatus and infraspinatus muscles are responsible for 90% of the ER strength of the shoulder. The teres minor also contributes to ER of the glenohumeral joint. The subscapularis is the primary internal rotator, with abduction activity peaking at around 90°.

Shoulder elevation
Most glenohumeral motion occurs around the plane of the scapula. This plane of motion is approximately 30° to 45° anterior to the frontal plane. Codman first reported that abduction of the humerus to 180° overhead requires that the clavicle, scapula, and humerus move through essentially their full range of motion (ROM) in a specific pattern of interaction. When internally rotated, the humerus can abduct on the scapula to approximately 90° before the greater tubercle strikes up against the acromion. If the humerus is fully externally rotated, however, the greater tubercle and accompanying rotator cuff tendons clear the acromion, coracoacromial ligament, and superior edge of the glenoid fossa, thereby allowing another 30° of abduction. Thus, the glenohumeral joint contributes 90° to 120° of shoulder abduction, depending on the rotational position of the humeral head. The remaining 60° is supplied by scapular elevation. This combined motion between the scapula and the humerus is known as scapulohumeral rhythm. During the first 30° of glenohumeral abduction, the contribution of scapular elevation is negligible and is not coordinated with movement of the humerus. This is referred to as the setting phase, during which the scapula is seeking a position of stability on the thoracic wall in relation to the humerus. The purpose of scapular rotation is twofold : (1) to achieve a ratio of motion for maintaining the glenoid fossa in an optimal position to receive the head of the humerus, thus increasing ROM, and (2) to ensure that the accompanying motion of the scapula permits muscles acting on the humerus to maintain a satisfactory length-tension relationship. After the initial 30° of humeral elevation, scapular motion becomes better coordinated. Toward the end range of glenohumeral elevation, however, the scapula contributes more motion and the humerus less. In gross terms, it is generally agreed that every 1° of scapular motion is accompanied by 2° of humeral elevation. Scapulohumeral rhythm is therefore considered to be a ratio of 1:2. If scapular movement is prevented, only 120° of passive abduction and 90° of active motion are possible.
Clavicular motion at both the acromioclavicular and sternoclavicular joints is essential for full shoulder elevation. Inman and Saunders demonstrated that for full abduction of the arm to occur, the clavicle must rotate 50° posteriorly. Surgical pinning of the clavicle to the coracoid process, which has been performed in some cases in which a complete tear of the coracoclavicular ligament is present, dramatically limits shoulder abduction.
Glenohumeral elevation in abduction is the primary function of the deltoid and supraspinatus muscles. The contribution of the deltoid and supraspinatus to shoulder abduction has been investigated extensively. It was commonly assumed that abduction of the arm is initiated by the supraspinatus and is continued by the deltoid. Studies in which selective nerve blocks were used to deactivate the deltoid and supraspinatus muscles have shown that complete abduction still occurs, though with a 50% loss in power, when one or the other muscle is deactivated. Simultaneous nerve blocks of both these muscles result in an inability to raise the arm. Thus, each muscle can elevate the arm independently, but there is a resultant loss of approximately 50% of the normal power generated in abduction.
Additionally, the other three rotator cuff muscles—the teres minor, infraspinatus, and subscapularis—are active to some degree throughout the full abduction ROM. These three rotator cuff muscles work as a functional unit to compress the humeral head and counteract the superior shear of the deltoid during arm elevation.
Throwing mechanism
Throwing is an integral part of many sports, but different techniques are required, depending on the endeavor. High-speed motion analysis has allowed investigators to slow down the pitching act and examine the kinetics and kinematics involved ( Fig. 12-3 ). The throwing act, as performed by a baseball pitcher, is a series of complex and synchronized movements involving both the upper and lower extremities. As described by several authors, the throwing mechanism can be divided into five phases ( Fig. 12-4 ): (1) windup, (2) cocking, (3) acceleration, (4) release and deceleration, and (5) follow-through. Injuries to the shoulder joint can occur during the cocking, acceleration, or deceleration phases. The overhead throw is the fastest human movement and takes approximately 0.5 second to complete.


Windup
The purpose of the windup is to put the athlete in an advantageous starting position from which to throw. In addition, it can serve as a distraction to the hitter. The windup is a relatively slow maneuver that prepares the pitcher for correct body posture and balance while leading the body into the cocking phase (see Fig. 12-4 ). It can last from 0.5 to 1.0 second and is characterized by shifting of the shoulder away from the direction of the pitch, with the opposite leg being cocked quite high and the baseball being removed from the glove. It is also during this phase that the head of the humerus can wear and roughen from leverage on the posterior glenoid labrum.
Cocking
The cocking phase is most often divided into early and late cocking phases. During the cocking phase (see Fig. 12-4 ), the shoulder is abducted to approximately 90°, externally rotated 90° or more, and horizontally abducted to approximately 30°. This is primarily accomplished by the deltoid and stabilized by the rotator cuff muscles, which pull the humeral head into the glenohumeral joint. The anterior, middle, and posterior deltoids reach peak electromyographic (EMG) activity in the early cocking phase when the arm is abducted to 90°. During late cocking, the activity of the deltoids decreases as the rotator cuff musculature becomes more dominant. Additionally, the pectoralis major, subscapularis, and latissimus dorsi act eccentrically to stabilize the humeral head during the late cocking phase. This position places the anterior joint capsule and internal rotators, which are used to accelerate the ball, in maximum tension. In addition, the opposite leg is kicked forward and placed directly in front of the body. Kinetic energy begins to be transferred from the lower extremities and trunk to the arm and hand in which the ball is held. Because of the significant stress placed on the anterior shoulder capsule, the capsule may “stretch out” with continuous throwing. If the anterior capsule is significantly stretched out, increased humeral head displacement during the late cocking phase can occur. This may be manifested clinically as “internal impingement” and is discussed in detail later. Furthermore, this extreme ER position (late cocking) may also lead to glenoid labrum lesions. This type of glenoid labrum lesion has been referred to as a peel-back lesion and is also discussed later.
Acceleration
The acceleration phase (see Fig. 12-4 ) begins at the point of maximal ER and ends at ball release. This phase lasts an average of 50 msec, approximately 2% of the duration of the pitching act. Muscles that once were placed on stretch in the cocking phase become the accelerators in a powerful concentric muscular contraction. The body is brought forward, with the arm following behind. The energy developed by the body’s forward motion is transferred to the throwing arm to accelerate the humerus. This energy is enhanced via contraction of the internal rotators (primarily the subscapularis) as the humerus is rotated internally from its previously externally rotated position, and the ball is accelerated to delivery speed. During this phase the maximum IR angular velocity is approximately 7365 ± 1503°/sec. An anterior displacement force of approximately 50% of body weight also occurs during this phase. Rotatory torque at the shoulder can start at approximately 14,000 inch-pounds and builds up to approximately 27,000 inch-pounds of kinetic energy at ball release.
During the acceleration phase, the pectoralis major and latissimus dorsi are the main muscles that actively generate velocity and arm speed. The subscapularis muscle is active in steering the humeral head. At ball release, the throwing shoulder should be abducted about 90° to 100°, regardless of the type of pitch being thrown or the style of the thrower. The difference between an “overhead” and a “sidearm” baseball pitcher is not the degree of glenohumeral abduction, but rather the degree of lateral tilt at the trunk. Because of the tremendous force acting at the glenohumeral joint, numerous injuries can result during this phase of overhead throwing, such as instability, labral tears, overuse tendinitis, and tendon ruptures.
Release and Deceleration
In the release and deceleration phases of throwing, the ball is released, and the shoulder and arm are decelerated (see Fig. 12-4 ). Generally, deceleration forces are approximately twice as great as acceleration forces but act for a shorter period (approximately 40 msec). Initially in the deceleration phase, the humerus has a relatively high rate of IR, and the elbow extends rapidly. Great eccentric force is applied to the posterior rotator cuff muscles to slow the IR and horizontal adduction of the humerus and to stabilize the humeral head within the glenoid cavity. Jobe et al analyzed the throwing mechanism with electromyography and found that the muscles of the rotator cuff are extremely active during the deceleration phase. It has been reported that the posterior rotator cuff must resist as much as 200 lb of distraction force that is attempting to pull the arm out of the glenohumeral joint in the direction that the ball has been thrown. Labral tears at the attachment of the long head of the biceps, subluxation of the long head of the biceps as a result of tearing of the transverse ligament, and various lesions of the rotator cuff, such as tearing of the undersurface or tensile overload, can be incurred during this phase of throwing.
Follow-Through
During the follow-through phase (see Fig. 12-4 ), the body moves forward with the arm, thereby effectively reducing the distraction force applied to the shoulder. This results in relief of tension on the rotator cuff muscles.
Because of the repetitive action of throwing, a baseball pitcher’s shoulder undergoes adaptive changes that should be recognized and distinguished from pathologic lesions. The throwing shoulder has significantly increased ER and decreased IR in comparison to that on the nonthrowing side. This is considered a functional adaptation to throwing but may lead to specific pathologic conditions as discussed later. The various shoulder injuries that can occur in each phase of throwing are summarized in Table 12-2 .
Phase | Potential Injuries |
---|---|
Windup | None that are common |
Cocking | Anterior subluxation Internal impingement Glenoid labrum lesions |
Acceleration | Shoulder instability Labral tears Overuse tendinitis Tendon ruptures |
Release and deceleration | Labral tears at the attachment of the long head of the biceps Subluxation of the long head of the biceps by tearing of the transverse ligament Lesions of the rotator cuff, such as undersurface tears or tensile overload |
Follow-through | Tear of the superior aspect of the glenoid labrum at the origin of the biceps tendon Abnormal glenohumeral kinematics caused by tight posterior shoulder structures forcing the humeral head anteriorly and superiorly into the acromial arch during this phase |
Glousman et al compared the EMG activity of the shoulder girdle muscles in pitchers with isolated anterior glenohumeral instability and in normal subjects. In pitchers, the supraspinatus and serratus anterior exhibited increased activity throughout late cocking and acceleration, the infraspinatus exhibited enhanced activity during early cocking and acceleration, and the biceps brachii was noted to have an increase in activity during the acceleration phase. Additionally, the subscapularis, latissimus dorsi, and pectoralis major demonstrated less EMG activity in subjects with anterior instability during all phases of the throwing motion.
Wick et al noted numerous significant differences between throwing a baseball overhead and throwing a football. During the acceleration phase, the angular velocity for throwing a baseball was 7365° ± 1503°/sec versus 4586° ± 843°/sec for throwing a football. At ball release, the shoulder was externally rotated approximately 21° more for throwing a football. Also at ball release, shoulder abduction was calculated to be 99° during baseball throwing and 114° during football throwing. The duration of the football throw was also significantly longer (0.20 second) than a baseball throw (0.15 second).
Overview of the rehabilitation program
The shoulder rehabilitation program presented in this chapter is designed to restore shoulder ROM and strength in a functional and progressive manner. The exercises may be implemented with specific limitations of certain motions, depending on the injury sustained or surgical procedure performed. Frequently, emphasis is placed on the rotator cuff musculature and the scapular stabilizers. Rehabilitation exercises that concentrate on the rotator cuff musculature are paramount after any shoulder injury. With shoulder rehabilitation the clinician should concentrate on increasing dynamic stability, particularly that of the rotator cuff, because of the relatively weak nature of the static restraints of the glenohumeral joint.
The entire upper kinematic chain should be evaluated when a shoulder rehabilitation program is designed because of the importance of proximal stability for distal mobility. Synchronous interplay among each of the joints of the shoulder complex is vital for active, balanced joint stability and normal glenohumeral function. The scapular stabilizers (e.g., trapezius, latissimus dorsi, rhomboids, and serratus anterior) are often overlooked when shoulder injuries are addressed. The scapular stabilizers maintain the appropriate scapula–glenohumeral joint relationship, thereby allowing normal kinematics during functional activities. This is particularly true in the pitching motion, in which these muscles play a large role during the deceleration phase of throwing.
Strengthening exercises for the shoulder musculature, especially the rotator cuff, have been critically analyzed by numerous investigators. Jobe and Moynes first examined the effect of specific exercises on the rotator cuff musculature. They reported that the supraspinatus can best be exercised apart from the other cuff muscles with the arm abducted to 90°, horizontally adducted to 30°, and fully internally rotated, which is referred to as the empty can position. The infraspinatus and teres minor can be exercised in the side-lying position with the arm held close to the side and the elbow flexed to 90°. The subscapularis can be strengthened with the individual in the supine position, the affected arm held close to the side, and the elbow flexed to 90°.
Since the study of Jobe and Moynes, Blackburn et al investigated rotator cuff activation with intramuscular electromyography while subjects performed specific rotator cuff exercises. Although Jobe and Moynes reported that the supraspinatus is best isolated and exercised with the arm abducted to 90°, horizontally flexed 30°, and fully internally rotated, Blackburn et al noted that the supraspinatus is involved whenever the arm is elevated, whether the subject is standing or prone. Isolation of supraspinatus function appears to occur during pure abduction with neutral rotation of the arm while standing. In addition, Blackburn et al noted that a significant increase in supraspinatus function can be achieved in the prone position with the arm in maximal ER and 100° of horizontal abduction ( Fig. 12-5 ). Malanga et al analyzed the EMG activity of the rotator cuff and deltoid muscles in the empty can and prone positions advocated by Blackburn et al. The empty can position produced high levels of EMG activity in the supraspinatus (107% maximum voluntary isometric contraction), but the middle deltoid (104%) and anterior deltoid (96%) were also extremely active. Conversely, during prone horizontal abduction (at approximately 100°) with ER, the most active muscles were the middle deltoid (111%), the posterior deltoid (96%), and the supraspinatus (94%). The investigators concluded that neither position isolated the supraspinatus but that both positions could be used for exercising. In addition, Kelly et al reported that the best test position to isolate the supraspinatus with the least activation of the infraspinatus is with the arm positioned at 90° of elevation in the scapular plane and 45° of humeral ER. This position is referred to as the full can position. We recommend this position for manual muscle testing of the supraspinatus ( Fig. 12-6 ). Townsend et al studied seven glenohumeral muscles during 17 traditional shoulder exercises and reported that certain exercises were better for recruiting selected muscles. For instance, the best exercise noted in the study to recruit the teres minor muscle was side-lying ER followed by prone horizontal abduction with ER. Table 12-3 provides detailed information about specific exercises and muscular activity.


Muscle and Exercise | Electromyographic Activity (% MVIC ± SD) | Peak Arc Range (°) |
---|---|---|
Anterior Deltoid | ||
Scaption, IR | 72 ± 23 | 90-150 |
Scaption, ER | 71 ± 39 | 90-120 |
Flexion | 69 ± 24 | 90-120 |
Middle Deltoid | ||
Scaption, IR | 83 ± 13 | 90-120 |
Horizontal abduction, IR | 80 ± 23 | 90-120 |
Horizontal abduction, ER | 79 ± 20 | 90-120 |
Posterior Deltoid | ||
Horizontal abduction, IR | 93 ± 45 | 90-120 |
Horizontal abduction, ER | 92 ± 49 | 90-120 |
Rowing | 88 ± 40 | 90-120 |
Supraspinatus | ||
Military press | 80 ± 48 | 0-30 |
Scaption, IR | 74 ± 33 | 90-120 |
Flexion | 67 ± 14 | 90-120 |
Subscapularis | ||
Scaption, IR | 62 ± 33 | 90-150 |
Military press | 56 ± 48 | 60-90 |
Flexion | 52 ± 42 | 120-150 |
Infraspinatus | ||
Horizontal abduction, ER | 88 ± 25 | 90-120 |
ER | 85 ± 26 | 60-90 |
Horizontal abduction, IR | 74 ± 32 | 90-120 |
Teres Minor | ||
ER | 80 ± 14 | 60-90 |
Horizontal abduction, ER | 74 ± 28 | 60-90 |
Horizontal abduction, IR | 68 ± 36 | 90-120 |
In a recent study performed at our center, Fleisig reported that the exercise movement that produced the highest EMG activity in the posterior rotator cuff was side-lying ER, followed by prone ER, and then standing ER at 90° of abduction. Furthermore, the investigators noted that use of a towel roll between the humerus and body enhanced EMG activity of the infraspinatus and teres minor by 18% to 20%. Moreover, they showed via an indwelling EMG study that the full can exercise produced significantly less activity of the middle and posterior deltoid muscles and may be the optimal position to recruit the supraspinatus muscle for rehabilitation and manual muscle testing.
After injury or surgery, modalities may be used as needed. Cryotherapy both before and after exercise is recommended in the acute stages of healing to reduce the inflammatory process. Cryotherapy may also be used prophylactically after the acute phase has subsided. Iontophoresis, moist heat, or ultrasound may be indicated for chronic overuse injuries of the shoulder to promote blood flow and a healing response in the tissue.
Therapeutic exercises for the shoulder should address strength, endurance, and dynamic stability. A variety of machines, dumbbells, and manual techniques can be used to accomplish specific goals. An upper body ergometer can be used in the early phases of rehabilitation for restoration of ROM and in the later phases for muscular endurance. Exercises that result in an eccentric contraction are particularly important for athletes performing overhead movements, especially for the muscles involved in deceleration movements of the shoulder. Manual resistance exercises, such as rhythmic stabilization exercises, can be used to promote cocontractions and facilitate muscle synergy ( Fig. 12-7 ). Finally, surgical tubing is a versatile tool that can be used for strengthening in diagonal patterns or simulating the throwing act ( Figs. 12-8 and 12-9 ). Use of each of these exercise types during the rehabilitation process depends on the goals of the particular phase. Specific uses for each exercise are explained throughout the treatment sections.



Glenohumeral mobilization techniques (see Figure A6-1 , Figure A6-2 , Figure A6-3 , Figure A6-4 , Figure A6-5 , Figure A6-6 on Expert Consult @ www.ExpertConsult.com ) are important for attaining accessory motion in the early stages of healing without subjecting the joint to the high forces involved in passive stretching. Grade I or II anterior-posterior, inferior-superior, and long arm distraction mobilizations can be used early in the rehabilitation program to neuromodulate the athlete’s pain. Grade III or IV mobilizations can be added in later phases of rehabilitation to increase flexibility within the capsule. In older patients (aged 45 to 60), it is imperative to restore inferior capsular mobility early in the rehabilitation process (especially after rotator cuff repair).
Glenohumeral flexibility, particularly flexibility of the posterior shoulder structures, is paramount for athletes using overhead movements. This is particularly evident with inflexibility of the posterior capsule and musculature, which is manifested as decreased motion in horizontal adduction and IR. Tightness in the posterior aspect of the shoulder leads to increased stress on the posterior shoulder structures during the follow-through phase of pitching. Tight posterior shoulder structures can also cause abnormal glenohumeral kinematics by forcing the humeral head anteriorly and superiorly into the acromial arch. The flexibility exercises illustrated in Chapter 12 Appendix (see Figures A12-1 , Figures A12-2 , Figures A12-3 , Figures A12-4 , Figures A12-5 , Figures A12-6 , Figures A12-7 , Figures A12-8 , Figures A12-9 , Figures A12-10 , Figures A12-11 , Figures A12-12 , Figures A12-13 , Figures A12-14 , Figures A12-15 , Figures A12-16 , Figures A12-17 , Figures A12-18 , Figures A12-19 , Figures A12-20 , Figures A12-21 , Figures A12-22 , Figures A12-23 , Figures A12-24 , Figures A12-25 , Figures A12-26 , Figures A12-27 , Figures A12-28 , Figures A12-29 , Figures A12-30 , Figures A12-31 , Figures A12-32 , Figures A12-33 , Figures A12-34 , Figures A12-35 , Figures A12-36 , Figures A12-37 , Figures A12-38 , Figures A12-39 , Figures A12-40 on Expert Consult @ www.ExpertConsult.com ) should be undertaken not only after injury or surgery but also during the off-season to help prevent posterior glenohumeral restriction and injury ( Fig. 12-10, A and B ).

Muscular strength and endurance of the scapular stabilizing musculature are important for maintaining correct joint arthrokinematics. The role of the scapulothoracic joint and surrounding musculature in maintaining a normally functioning shoulder is critical. Muscular spasm, weakness, and poor neuromuscular coordination of these stabilizing muscles directly affect glenohumeral motion. If the scapula cannot rotate on the thoracic cage, maintain the correct length-tension muscular relationship, or properly orient the glenoid fossa with the humeral head, asynchronous motion at the shoulder complex will lead to injury. Scapula stabilizers can be strengthened by having the athlete perform push-ups, prone horizontal abduction, scapular retraction exercises, and neuromuscular control drills.
Because the rotator cuff muscles are mainly endurance-type muscles, the progressive resistance exercise (PRE) program is based on low weight and high repetition. This not only increases muscular endurance but also decreases the potential for perpetuating the inflammatory process by performing the exercises with too much weight. A common view held by numerous clinicians is that the PRE program should be progressed by having the athlete work from 30 to 50 total repetitions and not add weight until 50 repetitions can be performed comfortably (see Chapter 4 ). The PRE program is begun with a gradual progression toward more dynamic exercises as healing progresses. This decreases the chance of rotator cuff inflammation and trauma while still producing muscle strength and endurance of the rotator cuff.
On the basis of numerous studies and EMG recordings during specific movements, Wilk et al developed a core exercise program for athletes who use overhead movements called the Throwers’ Ten Exercise Program (see Figures A-1 , Figures A-2 , Figures A-3 , Figures A-4 , Figures A-5 , Figures A-6 , Figures A-7 , Figures A-8 , Figures A-9 , Figures A-10 on Expert Consult @ www.ExpertConsult.com . The program emphasizes the key muscles and muscle groups responsible for the throwing motion. The goal of this exercise program is to reestablish dynamic stability of the glenohumeral joint by strengthening the rotator cuff musculature and achieving neuromuscular control. Athletes should begin with a traditional PRE program and stretching exercises and gradually progress to eccentric and isokinetic exercises and then into a more dynamic program consisting of high-speed movements performed to muscular fatigue, proprioception exercises, use of inertia machines (see Fig. 4-11 ), and upper extremity plyometrics. The athlete should return to throwing gradually by progressing through an appropriate interval rehabilitation program, as outlined in Appendix B (on Expert Consult @ www.ExpertConsult.com ).
A plyometric exercise program can be extremely useful in the advanced phases of rehabilitation of an injured athlete. Plyometric exercise combines strength with speed of movement and has significant implications for overhead throwers. The drills apply a stretch-shortening cycle to the muscle, that is, an eccentric contraction followed by a concentric contraction. This stimulates the neurophysiologic components of the muscle to produce greater force. Table 12-4 presents the three phases of a plyometric drill. Successful plyometric training relies heavily on the rate of stretch rather than on the length of stretch. With plyometric exercise the neuromuscular system is trained by using the stretch reflex, proprioceptive stimulus, and muscular activation. Plyometrics should be performed only two or three times weekly because of the microtrauma that may occur with this type of aggressive exercise. Performing plyometrics daily can result in additional trauma to a healing shoulder in some athletes.
Phase | Description |
---|---|
I | Stretch phase or the eccentric muscle loading that activates the muscle spindle |
II | Amortization phase, which represents the time between the eccentric and concentric phases |
III | Shortening phase or the response or concentric phase |
The upper extremity plyometric program is organized into four different exercise groupings: (1) warm-up drills, (2) throwing movements, (3) trunk drills, and (4) wall drills. Some of the plyometric drills are illustrated in Appendix C (on Expert Consult @ www.ExpertConsult.com ), and others can be found in Chapter 26 . For a complete description, the reader is encouraged to review several articles.
The nonoperative rehabilitation program for an overhead thrower can be divided into four distinct phases. Each phase should represent specific goals and contain various exercises to accomplish these goals. Box 12-3 provides an overview of the program.
Phase I: acute phase
Goals :
- •
Diminish pain and inflammation
- •
Normalize or improve motion and flexibility
- •
Retard muscular atrophy
- •
Enhance dynamic stabilization
- •
Phase II: intermediate phase
Goals :
- •
Improve muscular strength and endurance
- •
Maintain or improve flexibility
- •
Promote concentric-eccentric muscular training
- •
Maintain dynamic stabilization
- •
Phase III: advanced phase
Goals :
- •
Initiate sport-specific training
- •
Enhance power and speed (plyometrics)
- •
Maintain a rotator cuff strengthening program
- •
Improve muscular endurance
- •
Phase IV: return-to-sport phase
Goals :
- •
Gradually return to sports activities
- •
Maintain gains in strength, power, endurance, and flexibility
- •
Nonoperative rehabilitation guidelines
Range of Motion
Most throwers exhibit an obvious disparity in motion in which ER is excessive and IR is limited in 90° of abduction. Several investigators have documented the fact that pitchers have greater ER than position players do. Brown et al reported that professional pitchers exhibited 141° ± 15° of ER measured at 90° of abduction. This was approximately 9° more than the ER of the nonthrowing shoulder and approximately 9° more than that of the throwing shoulder of positional players measured in 90° of abduction. Bigliani et al examined the ROM of 148 professional players. They reported that a pitcher’s ER at 90° of abduction averaged 118° (range, 95° to 145°) on the dominant shoulder whereas the ER of a position player’s dominant shoulder averaged 108° (range, 80° to 105°). In an ongoing study of professional baseball players, Wilk et al assessed the ROM of 369 professional baseball pitchers. They noted that pitchers exhibit an average of 132.9° of ER and 52° of IR when passively assessed at 90° of abduction. In pitchers, ER is approximately 7° greater in the throwing shoulder than in the nonthrowing shoulder, whereas IR is 7° greater in the nonthrowing shoulder. They also noted that pitchers exhibit the greatest total arc of motion (i.e., ER and IR at 90° abduction), followed closely by catchers, then outfielders, and finally infielders. Furthermore, when left-handed pitchers are compared with right-handed pitchers, left-handed throwers exhibit approximately 7° more ER and 12° more total motion than right-handed throwers. These findings were statistically significant ( P < .01).
Recently, the disparity between IR of the dominant and nondominant shoulder has received increased attention from numerous clinicians. This difference, evidenced as less IR in the throwing shoulder, has been referred to as glenohumeral joint IR deficit (GIRD). GIRD, as defined by Burkhart et al, is a loss of IR of the throwing shoulder of 20° or greater in comparison to the nonthrowing shoulder and may be a cause of specific shoulder injuries. In addition to the GIRD principle, Wilk et al proposed the total rotational motion (TRM) concept, in which the amount of ER and IR at 90° of abduction is added together and a TRM arc is determined. The authors reported that the TRM in the throwing shoulders of professional baseball pitchers is within 5° of the nonthrowing shoulder. Furthermore, the authors suggested that a TRM arc outside the 5° range may be a contributing factor to shoulder injuries in the presence or absence of GIRD.
As a result of the repetition of such high forces generated during the throwing motion, an overhead-throwing athlete can exhibit numerous and substantial adaptations. Some of the most common adaptations seen occur at the glenohumeral joint. Most throwers have an obvious motion disparity whereby ER is excessive and IR is limited at 90° of abduction. , The loss of IR has been reported in the literature by numerous authors. Numerous reasons have been proposed for the motion adaptations, including osseous adaptations (increased retroversion), posterior musculature, and soft tissue adaptations of the capsule. Crockett et al tested dominant and nondominant shoulder retroversion in 25 professional pitchers and compared them with a control group of nonthrowers’ shoulders. They reported that the humeral head of the throwing shoulder had an 17° increase in retroversion in comparison to the nonthrowing shoulders. Reinold et al reported that after a pitching performance, there is 9.5° loss of glenohumeral joint IR that lasts for 24 hours. Burkhart et al suggested that GIRD is the result of posterior capsular tightness. They concluded that stretching is the most appropriate treatment to address the IR deficit. They went on to recommended a posterior capsular release should an aggressive stretching program not improve IR passive range of motion (PROM).
Borsa et al documented that pitchers have greater posterior translation than anterior translation. Furthermore, they reported that some individuals with very small amounts of IR PROM exhibit considerable posterior glenohumeral laxity on objective testing. They have also proposed that the loss of IR is the result of muscular tightness and humeral head retroversion and not posterior capsular tightness. Crockett et al and others documented greater retroversion in throwers’ dominant shoulder than in their nondominant shoulder. Thus, the primary and most frequent cause of the change in IR is the result of osseous adaptation, muscular tightness of the external rotators, and scapular positioning. Consequently, if the loss of IR is the result of osseous adaptation and muscular tightness, a stretching program directed toward the tightened posterior rotator cuff muscles appears to be most appropriate for treating GIRD. In this review we reported that pitchers exhibited greater glenohumeral ER and less IR on the throwing side than on the nonthrowing side. This is consistent with previous research. Furthermore, the average TRM was within 6°, nearly the same as reported by Wilk et al in a previously published article.
There appears to be increasing concern regarding the correlation between sustaining a shoulder injury and the loss of IR and GIRD. In a study looking at the relationship between ROM and injury rates in professional baseball pitchers, we found that pitchers with GIRD had almost twice the risk of sustaining a shoulder injury than did pitchers without GIRD. Pitchers whose TRM comparison was outside the acceptable 5° difference range had a 2.5 times higher risk of sustaining a shoulder injury. Clinically, we believe that pitchers with GIRD and TRM differences outside the 5° window are at greater risk for injury.
Laxity
Most throwers have significant laxity of the glenohumeral joint, which permits excessive ROM. The hypermobility of the thrower’s shoulder has been referred to as thrower’s laxity . The laxity of the anterior and inferior glenohumeral joint capsule can be recognized by the clinician during assessment of the stability of the overhead thrower’s shoulder joint. Posteriorly, however, Borsa et al documented that pitchers exhibit greater posterior translation than anterior translation. Furthermore, they reported that some individuals with very small amounts of IR PROM are found to have considerable posterior glenohumeral laxity on objective testing. They have also proposed that the loss of IR is the result of muscular tightness and humeral head retroversion and not posterior capsular tightness. Some clinicians have reported that the excessive laxity exhibited by throwers is the result of repetitive throwing and have referred to this as acquired laxity , whereas others have documented that overhead throwers have congenital laxity . Bigliani et al examined glenohumeral laxity in 72 professional baseball pitchers and 76 position players. They noted a high degree of inferior glenohumeral joint laxity, with 61% of pitchers and 47% of position players exhibiting a positive sulcus sign in the throwing shoulder. Additionally, in the players who exhibited a positive sulcus sign in the dominant shoulder, 89% of the pitchers and 100% of the position players had a positive sulcus sign in the nondominant shoulder. Thus, it would appear that some baseball players have inherent congenital laxity, with superimposed acquired laxity occurring as a result of adaptive changes from throwing.
Muscular Strength
Several investigators have examined muscular strength parameters in overhead-throwing athletes with varying results and conclusions. Wilk et al performed isokinetic testing on professional baseball players as part of their physical examination during spring training ( Table 12-5 ). They demonstrated that the ER strength of a pitcher’s throwing shoulder is significantly weaker ( P > 0.05) than that of the nonthrowing shoulder by 6%. Conversely, the IR strength of the throwing shoulder was significantly stronger ( P < 0.05) by 3% than that of the nonthrowing shoulder. In addition, adduction strength of the throwing shoulder was also significantly stronger than that of the nonthrowing shoulder by approximately 9% to 10%. The authors believe that important isokinetic values are the unilateral muscle ratios, which describe the antagonist/agonist muscle strength relationship. A proper balance between agonist and antagonist muscle groups is thought to provide dynamic stabilization to the shoulder joint. To provide proper muscular balance, the ERs should be at least 65% of the strength of the IRs. Optimally, the external-to-internal rotator muscle strength ratio should be 66% to 75%. This provides proper muscular balance. Magnusson et al measured the isometric muscular strength values of professional pitchers with a handheld dynamometer and compared them with the values in a control group of nonthrowing, nonathletic individuals. In pitchers, the supraspinatus muscle was significantly weaker on the throwing side than on the nonthrowing side when measured by an isometric manual muscle test (the empty can maneuver). Additionally, shoulder abduction, ER, IR, and the supraspinatus muscle were weaker in pitchers than in the control group of non–baseball players.
A. Bilateral Comparisons—Glenohumeral Joint |
180°/sec (%) | 300°/sec (%) | 450°/sec (%) | ||||||
---|---|---|---|---|---|---|---|---|
ER | 95-109 | 85-95 | 80-80 | |||||
IR | 105-120 | 100-115 | 100-110 | |||||
Abd | 100-110 | 100-110 | — | |||||
B. Unilateral Muscle Ratios—Glenohumeral Joint |
180°/sec (%) | 300°/sec (%) | 450°/sec (%) | ||||||
---|---|---|---|---|---|---|---|---|
ER/IR | 63-70 | 65-72 | 62-70 | |||||
Abd/Add | 82-87 | 92-97 | — | |||||
ER/Abd | 64-69 | 66-71 | — | |||||
C. Isokinetic Torque/Body Weight Ratios—Glenohumeral Joint |
180°/sec (%) | 300°/sec (%) | |||||||
---|---|---|---|---|---|---|---|---|
ER | 18-23 | 15-20 | — | |||||
IR | 27-33 | 25-30 | — | |||||
Abd | 26-32 | 20-26 | — | |||||
Add | 32-36 | 28-33 | — | |||||
D. Scapular Muscle |
Protract | Retract | Elevation | Depression | |||||
---|---|---|---|---|---|---|---|---|
D | ND | D | ND | D | ND | D | ND | |
Catchers | 71 ± 10 | 74 ± 13 | 62 ± 8 | 60 ± 7 | 83 ± 14 | 84 ± 5 | 22 ± 6 | 18 ± 5 |
Position players | 68 ± 10 | 73 ± 10 | 63 ± 5 | 59 ± 7 | 88 ± 15 | 85 ± 8 | 21 ± 4 | 16 ± 5 |
E. Unilateral Muscle Ratios—Scapular |
Protraction/Retraction | Elevation/Depression | |||||||
---|---|---|---|---|---|---|---|---|
D (%) | ND (%) | D (%) | ND (%) | |||||
Pitchers | 87 | 81 | 27 | 21 | ||||
Catchers | 93 | 81 | 24 | 19 | ||||
Position players | 98 | 94 | 29 | 27 |
The scapular muscles play a vital role during the overhead-throwing motion. Proper scapular movement and stability are imperative for asymptomatic shoulder function. These muscles work in a synchronized fashion and act as force couples about the scapula to provide both movement and stabilization. Wilk et al documented the isometric scapular muscular strength values of professional baseball players. Their results indicated that pitchers and catchers exhibit a significantly different increase in strength of the scapular protractors and elevators than position players do (see Table 12-5 ). All players (except infielders) had significantly stronger scapular depressors on the throwing side than on the nonthrowing side. In addition, they believed that optimal agonist/antagonist muscular ratios are important for the scapular muscles in providing stability, mobility, and symptom-free shoulder function. It is often clinically beneficial to enhance the ratio of lower trapezius to upper trapezius strength. In the opinion of the authors, the poor posture and muscle imbalance commonly seen in patients with a variety of shoulder pain pathology are often a result of poor muscle balance between the upper and lower trapezius, with the upper trapezius being more dominant. A study by McCabe et al reported that bilateral ER at 0° of abduction resulted in the greatest lower trapezius–to–upper trapezius ratio when compared with several other similar trapezius exercises ( Fig. 12-11 ). Cools et al also identified side-lying ER and prone horizontal abduction at 90° and ER as two beneficial exercises to enhance the ratio of lower to upper trapezius activity.

Relatively high lower trapezius activity occurs in prone rowing; prone horizontal abduction at 90° and 135° with ER and IR; prone and standing ER at 90° of abduction; D 2 diagonal pattern flexion and extension; proprioceptive neuromuscular facilitation (PNF) scapular clock; standing high scapular rows; and scaption, flexion, and abduction below 80° and above 120° with ER. Lower trapezius activity tends to be relatively low at less than 90° of scaption, abduction, and flexion and then increases exponentially from 90° to 180°. Significantly greater lower trapezius activity has been reported with prone ER at 90° of abduction than with the empty can exercise. As mentioned previously, the lower trapezius is an extremely important muscle in shoulder function because of its role in scapular upward rotation, ER, and posterior tilt.
The serratus anterior works with the pectoralis minor to protract the scapula and with the upper and lower trapezius muscles to upwardly rotate the scapula. The serratus anterior is an important muscle because it contributes to all components of normal three-dimensional scapular movements during arm elevation, including upward rotation, posterior tilt, and ER. The serratus anterior is likewise important in athletics, such as during overhead throwing, to accelerate the scapula during the acceleration phase of throwing. The serratus anterior also helps stabilize the medial border and inferior angle of the scapula and thereby prevent scapular IR (“winging”) and anterior tilt.
Several exercises elicit high serratus anterior activity, such as D 1 and D 2 diagonal pattern flexion; D 2 diagonal pattern extension; supine scapular protraction; supine upward scapular punch; military press; push-up plus; IR and ER at 90° of abduction; and flexion, abduction, and scaption above 120° with ER. , Serratus anterior activity tends to increase in a somewhat linear fashion with arm elevation. 154 However, increasing arm elevation increases risk for subacromial impingement, and arm elevation at lower abduction angles also generates relatively high serratus anterior activity.
Not surprising is high serratus anterior activity generated during the push-up. When performing the standard push-up, push-up on knees, and wall push-up, serratus activity is greater when full scapular protraction occurs after the elbows fully extend (push-up plus) ( Fig. 12-12 ). Moreover, serratus anterior activity was lowest in the wall push-up plus, exhibited moderate activity during the push-up plus on knees, and had relatively high activity during the standard push-up plus. When compared with the standard push-up, performing a push-up plus with the feet elevated produced significantly greater serratus anterior activity. These findings demonstrate that serratus anterior activity increases in a linear manner as the positional challenge increases.

Proprioception
Proprioception is defined as the conscious or unconscious awareness of joint position, whereas neuromuscular control is the efferent motor response to afferent (sensory) information. The thrower relies on enhanced proprioception to influence the neuromuscular system to dynamically stabilize the glenohumeral joint because of significant capsular laxity and excessive ROM. Allegrucci et al tested shoulder proprioception in 20 healthy athletes participating in various overhead sports. Testing of joint proprioception was performed on a motorized system with the subject attempting to reproduce a specific joint angle. These investigators noted that the dominant shoulder exhibited diminished proprioception in comparison to the nondominant shoulder. They also noted improved proprioception near end ROM in comparison to that at the starting point. Blasier et al reported that individuals who have clinically appreciable generalized joint laxity are significantly less sensitive during proprioceptive testing. Wilk et al (unpublished data, 2000) studied the proprioception capability of 120 professional baseball players. They passively positioned the players at a documented point within the players’ ER ROM. The athletes were then instructed to actively reposition the shoulder in the same position. The researchers noted no significant difference between the throwing shoulder and nonthrowing shoulder. In addition, Wilk et al (unpublished data, 2000) compared the proprioception ability in 60 professional baseball players with that of 60 non–overhead-throwing athletes. They noted no significant differences between baseball players and non–overhead-throwing athletes. However, baseball players exhibited slightly improved proprioception abilities at ER ROM than did non–overhead-throwing athletes, but these results were not significantly different. See Chapter 24 for an in-depth discussion of proprioception and neuromuscular control.
Painful Arc
A painful arc or “catching point,” particularly with shoulder abduction (but also possibly present in other planes), is characterized by specific points throughout ROM at which pain intensifies but dissipates once past that point. As many as three or four catching points may be present through an arc of motion. The most common ROM in which patients demonstrate a painful arc is from 70° to 120° of arm elevation. The athlete may attempt to exercise through these points of pain, which often dissipate within 5 to 7 days after initiation of treatment. Painful arcs in a young athlete can develop as a result of structural factors such as a hooked acromion, fracture malunion, or bursal swelling, or they may develop from loss of dynamic humeral head stability, which can cause the humeral head to displace superiorly during arm elevation. If the clinician determines the latter to be the cause, exercises that enhance dynamic stability should be emphasized immediately.
Crepitus
Crepitus within the shoulder joint is a common occurrence and is usually asymptomatic. Generally, crepitus can be detected after shoulder surgery or rotator cuff tendinitis. If the crepitus remains asymptomatic, the athlete should attempt to exercise through it. Crepitus should also dissipate within 7 to 10 days after initiation of an appropriate rotator cuff exercise program.
Middle Deltoid and Elbow Pain
Middle deltoid and elbow pain can be referred from the shoulder, often from a tight shoulder capsule. It is usually exacerbated with ER in the supine position with 90° of abduction and 90° of elbow flexion (90/90 position). This pain is generally seen in individuals with chronic shoulder problems or in those who have undergone prolonged immobilization. Frequently, the elbow pain at the end range of shoulder ER may be greater than that of the actual shoulder pain. This condition usually responds well to moist heat, application of ultrasound to the shoulder capsule, and glenohumeral joint mobilization. A shoulder with a limitation in ER should not be stretched aggressively in the 90/90 position because this will tend to exacerbate the elbow pain. Most patients can tolerate long arm distraction with imposed ER better than aggressive ER stretching in the 90/90 position. As treatment proceeds and normal synchronous glenohumeral motion is restored, the athlete should report a decrease in elbow and middle deltoid pain.
Pain Beyond 90° of Elevation
If impingement or rotator cuff tendinitis is present, pain beyond 90° of elevation is a common occurrence. Athletes with this complaint should begin active assisted range-of-motion (AAROM) exercises through full ROM, and joint mobilization techniques should be used as indicated. The pain is often propagated by a decrease in muscular strength and generally improves with a rotator cuff therapeutic exercise program. If symptoms are severe and the athlete has radiographic changes indicative of a grade III (or hooked acromion) type of injury, the prognosis is poor with nonoperative therapy.
Shoulder injuries
Scapulothoracic Joint Lesions
The scapulothoracic joint should also be assessed after any shoulder injury or surgery. Pathologic scapulothoracic conditions occur in chronic pain states in which glenohumeral motion has gradually decreased or the glenohumeral joint has been immobilized for a long period. In most instances the scapulothoracic joint becomes involved as a secondary problem. Increased shoulder pain can result in muscle spasm within the supraspinatus, trapezius, rhomboids, latissimus dorsi, and subscapularis. As mentioned earlier, for every 2° of glenohumeral abduction, approximately 1° of associated scapular motion must take place to achieve 180° of arm elevation. If the scapulothoracic joint is not functioning properly, the athlete may be able to attain only 100° to 120° of passive abduction, and active abduction may be even more limited.
The scapulothoracic joint should be evaluated for spasm in the rhomboids, latissimus dorsi, upper and lower trapezius, subscapularis, teres minor, infraspinatus, and supraspinatus muscles. These areas should also be assessed for active trigger points, as discussed by Travell and Simons. Activation of trigger points in these muscles may refer pain to the middle deltoid and elbow and, with severe spasm, down the arm. In addition, the clinician may find that the vertebral scapular border cannot be distracted off the thoracic cage, which results not only in increased pain for the athlete but also in an increase in localized muscle spasm.
Before decreased glenohumeral motion can be treated, scapulothoracic motion must be restored. This is usually accomplished by reducing the muscle spasm with moist heat, ultrasound, soft tissue mobilization, trigger point release, and scapular mobilization ( Fig. 12-13 ). With severe spasm, the spray-and-stretch technique of Travell and Simons, which uses Fluori-Methane spray *
* Available from Gebauer Chemical Co., Cleveland, Ohio.
to desensitize the trigger points, may be indicated. Trigger point injection may also be considered. As trigger points are diminished, the athlete should report a decrease in shoulder pain, neck stiffness, and referred pain, along with an associated increase in glenohumeral abduction. A careful cervical (see Chapter 16 ) and thoracic spine evaluation is necessary any time that a patient has scapular pain and muscle spasm.
Rotator Cuff Lesions
The rotator cuff can become injured through a variety of mechanisms. Most commonly, the cuff can become frayed, which can progress to a full-thickness tear after repetitive wear, and it gradually degenerates. However, in young athletes the cuff most commonly exhibits partial tearing or fraying with symptoms of tendinitis. Andrews and Meister classified rotator cuff lesions into categories based on the specific pathomechanics ( Table 12-6 ). A few of these categories will be briefly discussed as they pertain to an athlete with a shoulder injury. First, an athlete involved in overhead sports (i.e., thrower or swimmer) is prone to capsular laxity, which contributes to the clinical syndrome referred to as posterior impingement . In addition, such athletes are susceptible to undersurface rotator cuff tearing (tensile overload) and occasionally to compressive cuff disease. In contrast, athletes involved in collision sports such as football, lacrosse, or hockey are more likely to sustain a traumatic rotator cuff tear because of the athlete’s arm being forcefully abducted or violently pulled away from the body. Full-thickness tears of the rotator cuff are unusual in young athletes.
Type | Classification |
---|---|
1 | Primary compressive disease |
2 | Instability with secondary compressive disease |
3 | Primary tensile overload |
4 | Tensile overload because of capsular instability |
5 | Rotator cuff tear |
6 | Primary internal impingement |
7 | Calcific tendinitis |
8 | Partial articular-sided tendon avulsion (PASTA) lesion |
9 | Partial articular tear with intratendinous extension (PAINT) lesion |
10 | Secondary internal impingement with primary hypermobility |
11 | Secondary tensile overload with primary hypermobility |
Impingement Syndrome
The term impingement syndrome was popularized by Neer in 1972. He emphasized that both the supraspinatus insertion to the greater tubercle and the bicipital groove lie anterior to the coracoacromial arch with the shoulder in the neutral position and that with forward flexion of the shoulder, these structures must pass beneath the coracoacromial arch, thereby providing the opportunity for impingement. He introduced the concept of a continuum in the impingement syndrome from chronic bursitis to partial or complete tears of the supraspinatus tendon, which may extend to involve ruptures of other parts of the rotator cuff.
Impingement of the rotator cuff may occur in some athletes such as baseball players, quarterbacks, swimmers, and others whose activities involve repetitive use of the arm at or above 90° of shoulder abduction. Matsen and Arntz defined impingement as encroachment of the acromion, coracoacromial ligament, coracoid process, or acromioclavicular joint on the rotator cuff mechanism, which passes beneath them as the glenohumeral joint is moved, particularly in flexion and IR. Impingement usually involves the supraspinatus tendon. When the supraspinatus muscle assists in stabilizing the head of the humerus within the glenoid, the greater tubercle cannot butt against the coracoacromial arch. Whether impingement is the primary event causing rotator cuff tendinitis or whether rotator cuff impingement results from rotator cuff disease is undetermined. In all likelihood both mechanisms of injury can occur.
Approximately 5 to 10 mm of space lies between the humeral head and the undersurface of the acromial arch at 90° of shoulder abduction (depending on the anatomy). Thus, whenever the arm is elevated, some degree of rotator cuff impingement may occur. The shoulder is most vulnerable to impingement when the arm is at 90° of abduction and the scapula has not rotated sufficiently upward to free the rotator cuff of the overhanging acromion and coracoacromial ligament. Impingement of the glenohumeral joint can occur with horizontal adduction of the arm, which causes impingement against the coracoid process. Forward flexion with IR of the humerus also jams the greater tubercle under the acromion, the coracoacromial ligament, and at times, the coracoid process. If the arm is raised in ER, however, the greater tubercle is turned away from the acromial arch, and the arm can be elevated without impingement.
Impingement syndrome is perpetuated by the cumulative effect of many passages of the rotator cuff beneath the coracoacromial arch. This results in irritation of the supraspinatus and, possibly, the infraspinatus tendons, as well as in enlargement of the subacromial bursa, which can become fibrotic and thus further decrease the already compromised subacromial space. Furthermore, with time and progression of wearing and attrition, microtears and partial-thickness rotator cuff tears may result. If these continue, secondary bony changes (osteophytes) can develop under the acromial arch and propagate full-thickness rotator cuff tears.
Secondary factors may also contribute to the development of subacromial impingement. Issues of capsular hypomobility or hypermobility may result in altered glenohumeral arthrokinematics. The altered mechanics may overly stress the surrounding soft tissue structures, particularly the rotator cuff, and cause tendinopathy. Scapula position on the rib cage has also been implicated as a source of glenohumeral dysfunction. The term scapular dyskinesis describes an alteration in the normal position or motion of the scapula during coupled scapulohumeral movements. The altered scapula position may contribute to pathology of the rotator cuff, bursa, and surrounding structures. Rehabilitation is directed toward improving the scapula’s position and affording a normal scapulohumeral coupling motion, particularly with overhead movements.
The cause of impingement syndrome is usually multifocal, and the supraspinatus tendon is the structure most likely to be involved. Several factors have been proposed as contributing to the development of impingement syndrome. Tendon avascularity has long been thought to contribute to impingement. In 1939, Lindblom first reported avascularity of the rotator cuff at the supraspinatus attachment to the greater tubercle and described this as the “critical zone” in which many lesions occur. However, from their work, Moseley and Goldie concluded that the critical zone is no more avascular than the rest of the rotator cuff. Finally, Iannotti et al, using laser Doppler technology, reported substantial blood flow in the critical zone of the rotator cuff.
Although it now appears that the rotator cuff is not a completely avascular structure, Rathbun and Macnab and Sigholm et al proposed two mechanisms that may compromise supraspinatus blood flow. Rathbun and Macnab noted that shoulder adduction places the supraspinatus under tension and “wrings out” its vessels, thereby resulting in tissue necrosis. Sigholm et al demonstrated that active forward flexion increases subacromial pressure to a level sufficient to reduce tendon microcirculation substantially. However, in interpreting these findings, Matsen and Arntz point out that “since the shoulder is frequently moved, it is unclear whether either of these mechanisms could produce ischemia of sufficient duration to cause tendon damage.” Brewer reported that the blood supply to the critical zone diminishes with age. The relationship among cuff vascularity, impingement, and rotator cuff lesions is still speculative, and more research is needed. However, it appears that the critical zone is an area of hypovascularity and is prone to impingement and thus to tendon damage, especially in aging patients with a shoulder injury.
The shape of the acromion has been studied in individuals with impingement syndrome. Figure 12-14 illustrates the three types of acromial shape. It appears that rotator cuff lesions are more likely to occur if a hooked acromion is present, but it cannot be determined whether the acromial shape is caused by or results from a cuff tear.

Finally, a weakened rotator cuff mechanism can predispose an athlete to rotator cuff impingement. The rotator cuff functions to stabilize the shoulder against the actions of the deltoid and pectoralis major muscles. In the presence of a weakened cuff mechanism, contraction of the deltoid causes upward displacement of the humeral head, which squeezes the remaining cuff against the coracoacromial arch ( Fig. 12-15 ). Other factors that can result in rotator cuff impingement include degenerative spurs, chronic bursal thickening, rotator cuff thickening related to chronic calcium deposits, tightness of the posterior shoulder capsule, and capsular laxity.

Neer has described three progressive stages of impingement syndrome ( Fig. 12-16 ). Stage I is a reversible lesion usually seen in individuals younger than 25 years of age. These patients have an aching type of discomfort in the shoulder. This stage usually involves only inflammation of the supraspinatus tendon and long head of the biceps brachii. Stage II is generally seen in individuals 24 to 40 years of age and involves fibrotic changes in the supraspinatus tendon and subacromial bursa. Again, an aching type of pain is present, which may increase at night, and individuals may be unable to perform the movement that produces the impingement syndrome. Injuries in this stage sometimes respond to conservative treatment, but surgical intervention may be required. Stage III seldom occurs in those younger than 40 years of age. In this stage the individual has had a long history of shoulder pain, and osteophyte formation, a partial-thickness or eventually a full-thickness rotator cuff tear, and obvious wasting of the supraspinatus and infraspinatus muscles are often present. Injuries in this stage do not generally respond well to conservative treatment.

Rotator cuff impingement is a self-perpetuating process. Matsen and Arntz noted the following: (1) muscle or cuff tendon weakness causes impingement from loss of the humeral head stabilizing function, which leads leading to tendon damage, disuse atrophy, and additional cuff weakness; (2) bursal thickening causes impingement as a result of subacromial crowding, which produces greater thickening of the bursa; and (3) posterior capsular tightness can lead to impingement, disuse, and stiffness because the tight capsule forces the humeral head to rise up against the acromion. Numerous factors, both structural and functional, contribute to impingement, especially in young athletes. Additionally, if the capsule is especially lax and if the dynamic stabilizers are not sufficient, the humeral head may displace anterosuperiorly and lead to complaints of impingement.
The goal in treating athletes with impingement syndrome, either nonoperatively or surgically, is to reduce compression and friction between the rotator cuff and subacromial space. Box 12-4 lists several factors that are necessary to minimize the compression. The primary complication of impingement syndrome is a rotator cuff tear. If impingement syndrome is diagnosed in its early stages, the prognosis is encouraging. With acute impingement syndrome, time, rest from noxious stimuli, nonsteroidal antiinflammatory drugs, local modalities (e.g., cold, heat, and electrical stimulation), and a general shoulder rehabilitation program of flexibility and a PRE program (see Fig A12-1 , Fig A12-2 , Fig A12-3 , Fig A12-4 , Fig A12-5 , Fig A12-6 , Fig A12-7 , Fig A12-8 , Fig A12-9 , Fig A12-10 , Fig A12-11 , Fig A12-12 , Fig A12-13 , Fig A12-14 , Fig A12-15 , Fig A12-16 , Fig A12-17 , Fig A12-18 , Fig A12-19 , Fig A12-20 , Fig A12-21 , Fig A12-22 , Fig A12-23 , Fig A12-24 , Fig A12-25 , Fig A12-26 , Fig A12-27 , Fig A12-28 , Fig A12-29 , Fig A12-30 , Fig A12-31 , Fig A12-32 , Fig A12-33 , Fig A12-34 , Fig A12-35 , Fig A12-36 , Fig A12-37 , Fig A12-38 , Fig A12-39 , Fig A12-40 [on Expert Consult @ www.expertconsult.com ]) are indicated.
Shape of the coracoacromial arch, which allows passage of the adjacent rotator cuff mechanism
Normal undersurface of the acromioclavicular joint
Normal bursa
Normal function of the humeral head stabilizers (rotator cuff)
Normal capsular laxity
Smooth upper surface of the rotator cuff mechanism
Normal function of the scapular stabilizers
On evaluation, active ROM may be limited with an empty or muscular guarding end-feel as a result of pain. This may be caused by posterior capsule stiffness. In such instances, moist heat, ultrasound, joint mobilization, and a general shoulder flexibility program with emphasis on supine IR and ER and horizontal adduction are appropriate. Most young throwers will exhibit functional loss of IR and therefore have decreased flexibility of the posterior musculature or, less likely, a tight posterior capsule. It is important to stretch the posterior capsule (if tight) and inferior capsule and normalize the degree of IR. Box 12-5 provides an outline of the rehabilitation protocol after nonoperative treatment of shoulder impingement syndrome.
Subacromial impingement is a chronic inflammatory process produced by one of the rotator cuff muscles and the subdeltoid bursa “pinching” against the coracoacromial ligament and/or anterior acromion when the arm is raised above the head. The supraspinatus portion of the rotator cuff is the most common area of impingement. This syndrome is commonly seen in individuals who use their arms repetitively in a position above shoulder height. It also occurs in golfers, tennis players, and swimmers.
This four-phase program can be used for conservative management of impingement. The protocol is designed to attain maximal function in minimal time. This systematic approach allows specific goals and criteria to be met and ensures safe progression of the rehabilitation process. Client compliance is critical.
Maximal protection—acute phase
Goals :
- 1.
Relieve pain and inflammation
- 2.
Normalize range of motion
- 3.
Reestablish muscular balance
- 4.
Educate the patient and improve posture
- 1.
Avoidance :
- •
Elimination of any activity that causes an increase in symptoms
- •
Range of Motion :
- •
L – bar
- •
Flexion
- •
Elevation in the scapular plane
- •
External and internal rotation in the scapular plane at 45° of abduction
- •
Progress to 90° of abduction
- •
- •
- •
- •
Horizontal abduction/adduction
- •
Pendulum exercises
- •
Active assisted range of motion—limited symptom-free available range of motion
- •
Rope and pulley
- •
Flexion
- •
- •
Joint Mobilization :
- •
Emphasize inferior and posterior glides in the scapular plane
- •
Goal is to establish balance in the glenohumeral joint capsule
- •
Modalities :
- •
Cryotherapy
- •
Iontophoresis
- •
Strengthening Exercises :
- •
Rhythmic stabilization exercises for external/internal rotation
- •
Rhythmic stabilization drills: flexion/extension
- •
External rotation strengthening
- •
Submaximal isometrics (external rotation, internal rotation, abduction)
- •
Scapular strengthening
- •
Retractors
- •
Depressors
- •
Protractors
- •
Patient Education :
- •
Activity level, activities
- •
Pathologic condition of avoidance of overhead reaching and lifting activities
- •
Correct seating posture (consider a lumbar roll)
- •
Seated posture with shoulder retraction
- •
Guideline for Progression :
- 1.
Decreased pain and/or symptoms
- 2.
Normal range of motion
- 3.
Elimination of painful arc
- 4.
Muscular balance
- 1.
Intermediate phase
Goals :
- 1.
Reestablish nonpainful range of motion
- 2.
Normalize athrokinematics of shoulder complex
- 3.
Normalize muscular strength
- 4.
Maintain reduced inflammation and pain
- 1.
Range of Motion :
- •
L – bar
- •
Flexion
- •
External rotation at 90° of abduction
- •
Internal rotation at 90° of abduction
- •
Horizontal abduction/adduction at 90°
- •
- •
- •
Rope and pulley
- •
Flexion
- •
Abduction (symptom-free motion)
- •
Joint Mobilization :
- •
Continue joint mobilization techniques for the tight aspect of the shoulder (especially the inferior)
- •
Initiate self–capsular stretching
- •
Grade II/III/IV
- •
Inferior, anterior, and posterior glides
- •
Combined glides as required
- •
Modalities (As Needed) :
- •
Cryotherapy
- •
Ultrasound/phonophoresis
- •
Iontophoresis
- •
Strengthening Exercises :
- •
Progress to complete shoulder exercise program
- •
Emphasize rotator cuff and scapular muscular training
- •
External rotation tubing
- •
Side-lying external rotation
- •
Full can
- •
Shoulder abduction
- •
Prone horizontal abduction
- •
Prone rowing
- •
Prone horizontal abduction with external rotation
- •
Biceps/triceps
- •
Standing lower trapezius muscular strengthening
- •
- •
Functional Activities :
- •
Gradually allow increase in functional activities
- •
No prolonged overhead activities
- •
No lifting activities overhead
- •
Advanced strengthening phase
Goals :
- 1.
Improve muscular strength and endurance
- 2.
Maintain flexibility and range of motion
- 3.
Gradual increase in functional activity level
- 1.
Flexibility and Stretching :
- •
Continue all stretching and range-of-motion exercises
- •
L – bar external/internal rotation at 90° of abduction
- •
Continue capsular stretch
- •
Maintain/increase posterior/inferior flexibility
- •
Strengthening Exercises :
- •
Start fundamental shoulder exercises:
- •
Tubing external/internal rotation
- •
Lateral raises to 90° with dumbbell
- •
Full can with dumbbell
- •
Side-lying external rotation
- •
Prone horizontal abduction
- •
Prone extension
- •
Push-ups
- •
Biceps/triceps
- •
- •
Guideline for Progression :
- 1.
Full nonpainful range of motion
- 2.
No pain or tenderness
- 3.
Strength test fulfills criteria
- 4.
Satisfactory clinical examination
- 1.
Return-to-sport phase
Goal :
- 1.
Unrestricted symptom-free activity
- 1.
Initiate Interval Sport Program :
- •
Throwing
- •
Tennis
- •
Golf
- •
Maintenance Exercise Program :
- •
Flexibility exercises
- •
L – bar
- •
Flexion
- •
External rotation and internal rotation at 90° of abduction
- •
Self–capsular stretches
- •
- •
- •
- •
Isotonic exercises
- •
Fundamental shoulder exercises
- •
Perform 3 times per week
- •
- •
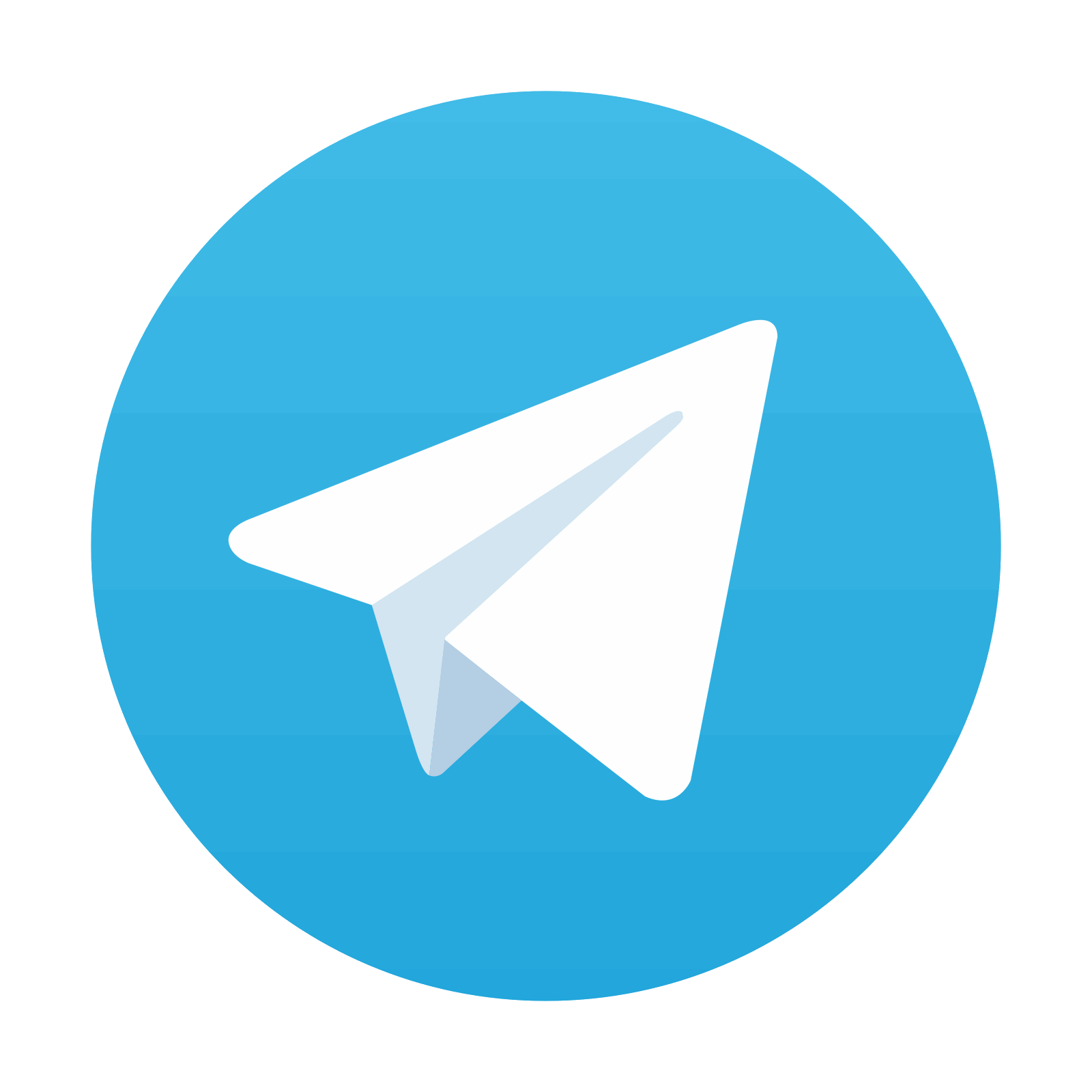
Stay updated, free articles. Join our Telegram channel

Full access? Get Clinical Tree
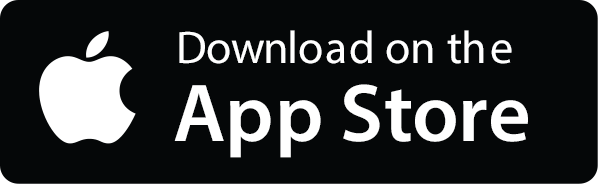
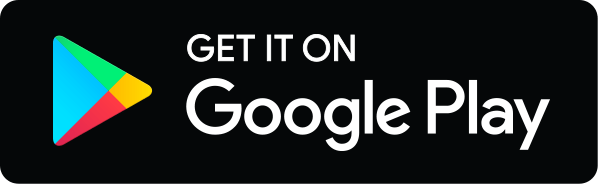
