Robotic Application of Unicompartmental Knee Arthroplasty
Joost A. Burger, MD
Andrew D. Pearle, MD
INTRODUCTION
Unicompartmental knee arthroplasty (UKA) has become a reliable treatment for isolated unicompartmental knee osteoarthritis (OA) that has a quicker recovery, improved function, less morbidity, lower risk of infection, and less blood loss compared to total knee arthroplasty.1,2 However, longevity of the UKA remains a concern, which contributes in preventing widespread acceptance. While high-volume UKA surgeons have reported comparable long-term survivorship to TKA, less specialized surgeons have shown inferior survival rates.3 Current registry-based studies have shown that surgeons, as well as hospital volume, influence survivorship for UKA.4,5
With the increased utilization of UKA, the understanding of the surgical factors influencing UKA outcomes has advanced. Lower limb alignment, implant positioning, implant size, and knee stability have all been shown to influence function and survivorship after UKA.6 When using a conventional technique, consistency of these surgical variables remains difficult in UKA, even when it is done by highly experienced surgeons.7 In addition, current surgeons often perform UKA with a minimally invasive technique to reduce soft-tissue and bone trauma, making the procedure even more demanding.8
Over almost 3 decades, technological advances have developed to control essential surgical variables to optimize UKA outcomes. In 1992, the first robotic system was used for joint arthroplasty. It was an autonomous system, meaning that the robot performed a predefined operative plan independent of any surgeon. Device-specific complications and the autonomous nature of the system led to concerns about the safety, resulting in initial rejection.9 Another technology used in the orthopedic operating room is computer-assisted navigation. This is classified as a passive device since it assists the surgeon in creating an operative plan and provides visual guidance while using surgical tools. Although this is a powerful visual aid, the outcome of the procedure still relies on conventional tools, which can limit the accuracy of the bone cuts. For this reason, semi-autonomous robotic systems were developed. In contrast to computer-assisted surgery, which provides passive guidance and feedback, these systems provide restraints for surgical bone resection within a predefined operative plan by feedback and safety controls. This prevents inadvertent bone removal and has been shown to be more accurate regarding implant placement than conventional techniques.10 In 2001, the first semi-autonomous robotic system was used in TKA, expanding later to UKA.11 Cobb et al studied the results of this system in UKA and showed that tibiofemoral alignment in the coronal plane was within 2° of the surgical plan in 100% of UKA patients using the semi-autonomous robotic system compared to 40% using a conventional technique.12 This led to growing interest and increase development of so-called robotic-assisted systems.
Currently, two robotic-assisted systems are commercially available for UKA. The Mako system (Stryker, Mahwah, NJ, USA) initially obtained FDA clearance in November 2008 and was first CE marked in January 2008. The Navio system (Smith & Nephew, Memphis, TN, USA) initially received FDA and CE Mark approval in February and December 2012, respectively. This chapter gives an overview of these systems and their performances in the setting of UKA, with the pearls and pitfalls of these systems being discussed.
MAKO SYSTEM
Registration and Planning Procedure
The Mako robot is an image-based system; therefore, a preoperative CT scan is acquired to generate a patient-specific 3D model of the knee. The system allows the surgeon to template the femoral and tibial component on the generated model, preoperatively, based on alignment parameters (Fig. 41-1). Before the patient arrives in the operation room, the Mako system is positioned. When the patient is positioned and sterilely draped, small incisions are used to place optical tracker pins into the tibia and femur, which helps the robotic system determine where the leg is in space. Next, an incision for the UKA procedure is made and anatomical landmarks are registered. The hip rotation center is located by rotating the femur in a clockwise fashion and an optically tracked pointer is used to capture ankle and knee landmarks (Fig. 41-2). The surgeon then registers several mapping points on the tibial and femoral bone surface, allowing the robotic system to merge the preoperative 3D model with the actual anatomy of the knee (Fig. 41-3). A virtual plan
is generated after the knee is put through a full range of flexion while valgus (medial UKA) or varus (lateral UKA) load is applied to restore collateral ligament tension. At this point the system provides the surgeon with implant tracking and gap-distance information throughout the entire range of flexion (Fig. 41-4). With these data, the surgeon will optimize the preoperative plan by adjusting component positioning and size, so that proper patient-specific kinematics can be restored and the Mako system can determine the final required bone resection areas.
is generated after the knee is put through a full range of flexion while valgus (medial UKA) or varus (lateral UKA) load is applied to restore collateral ligament tension. At this point the system provides the surgeon with implant tracking and gap-distance information throughout the entire range of flexion (Fig. 41-4). With these data, the surgeon will optimize the preoperative plan by adjusting component positioning and size, so that proper patient-specific kinematics can be restored and the Mako system can determine the final required bone resection areas.
![]() FIGURE 41-1 Mako example: preoperative planning of the femoral component on top of the CT-based patient-specific 3D model of the knee. |
![]() FIGURE 41-2 Mako example: the hip rotation center landmark is located by rotating the femur in a clockwise fashion. |
Plan Execution
To begin bone preparation in accordance with the surgeon’s plan, the high-speed saline-cooled burr employed by the robotic arm is placed in the surgical field. The robotic arm and burr are under direct control of the surgeon. During execution of the predefined plan, movement and bone resection with the burr is virtually visible on the monitor (Fig. 41-5). When the surgeon reaches the virtual resection boundaries, the system provides auditory and tactile feedback, restricting bone cuts outside predefined resection areas. In addition, the burr automatically stops if the surgeon goes outside the area or when rapid movement of the patient’s anatomy occurs. After the bone is properly prepared, trial implants are placed to test kinematics of the knee. Finally, the cemented UKA components are implanted and the appropriate polyethylene insert is chosen (Fig. 41-6). The Mako system has a closed-implant platform, allowing only one UKA implant design to be used during the procedure.
Accuracy and Reproducibility
The majority of the literature regarding accuracy and reproducibility of the Mako robot has shown promising results for UKA. A randomized controlled trial by Bell et al compared the accuracy of component positioning in the axial, coronal, and sagittal plane between 62 Mako and 58 conventional UKAs using postoperative CT scans. Root mean squared (RMS) errors were lower using robotic UKA in all component parameters (P < .01). The number of patients with implant positioning within 2° of the target position was significantly higher for all tibial and femoral planes using the Mako system.7 Lonner and colleagues retrospectively reviewed 27 conventional and 31 Mako UKAs. They found that RMS error of the tibial slope was 3.1° in the conventional group and 1.9° in the robotic-assisted group, with 2.6 times greater variability for the conventional group (P = .02). In the coronal plan, RMS error was 3.4° with the conventional technique and 1.8° with the Mako system (P < .0001).13 Earlier findings by Coon et al found similar results, comparing 44 conventional and 33 robotic UKAs.14 Citak et al
used cadavers to compare implant positioning between Mako (6 knees) and conventional UKAs (12 knees). The authors found that translation and angular RMS errors for implant orientation were better using Mako than the conventional UKA. RMS error differences between the two techniques were 3.5 mm and 6.5° for femoral component placement; and 4.3 mm and 14.2° for tibial component placement.15
used cadavers to compare implant positioning between Mako (6 knees) and conventional UKAs (12 knees). The authors found that translation and angular RMS errors for implant orientation were better using Mako than the conventional UKA. RMS error differences between the two techniques were 3.5 mm and 6.5° for femoral component placement; and 4.3 mm and 14.2° for tibial component placement.15
![]() FIGURE 41-5 Mako example: the burring process during bone resection of the tibia is displayed on the monitor. |
A noncomparative study by Dunbar et al reported accuracy of implant placement in 50 Mako UKA patients. The authors showed that translation and angular RMS error was within 1.6 mm and 3.0°, respectively, for both the femoral component and tibial component in all planes.16 Khamaisy et al showed improved congruence and restored joint space width of the lateral compartment after robotic medial UKA.17 Comparable findings were noted for robotic lateral UKAs.18 A study by Plate et al examined soft-tissue balancing in 52 UKA patients through a full range of flexion angles (0°, 30°, 60°, 90°, 110°). They observed soft-tissue balancing was accurate up to 0.53 mm compared to the preoperative plan, with 83% of the patients within 1 mm.19
Despite the aforementioned results favoring the Mako system, one retrospective study reported little to no radiographic differences between the Mako and conventional UKA.20 In addition, a prospective study by MacCallum et al compared tibial positioning of 87 Mako with 177 conventional UKAs using postoperative radiographs. Tibial baseplate positioning in the coronal plane was significantly more accurate with the Mako system than with the conventional technique; however, the conventional UKA was more accurate in the sagittal plane.21
Clinical Outcomes
Due to the relative recency of the Mako development, mid- to long-term data are not widely available yet. As this system is utilized more for UKA procedures, the available data pool will become larger and more mature. At this moment, Pearle et al have examined survivorship in a multicenter cohort of 1080 robotic UKAs with a minimum follow-up of 2 years (mean 2.5 years).22 A survival rate of 98.8% and a satisfaction rate of 92% were reported. Kleeblad et al studied survivorship and patient satisfaction as well, including 432 robotic UKAs with a minimum follow-up of 5 years (mean 5.7 years). They found a survival rate of 97.5% and reported that 91% patients were satisfied with their overall knee function.23 These findings seem to be higher than other large cohorts, including conventional UKAs.22,24
With regard to functional outcomes, a retrospective study by Hansen et al found greater range of movement at 2 weeks follow-up in the conventional than the Mako group. However, the authors reported that Mako UKA patients had a shorter length of stay in the hospital and earlier clearance of physical therapy.20 An RCT by Blyth et al showed faster decrease of postoperative pain scores in the Mako than the conventional group. In addition, the authors found significantly higher AKKS scores at 3 months follow-up for the robotic UKA patients,
although this significant difference was no longer observed at 1 year.25 Interestingly, comparative studies analyzing gait demonstrated that the Mako UKA resulted in a more normal gait pattern than conventional UKA at short- and mid-term follow-up.26,27 Although the literature is highly supportive of the Mako system’s accuracy when compared to conventional UKA, more evidence is needed in order to confidently evaluate the clinical benefits of each method.
although this significant difference was no longer observed at 1 year.25 Interestingly, comparative studies analyzing gait demonstrated that the Mako UKA resulted in a more normal gait pattern than conventional UKA at short- and mid-term follow-up.26,27 Although the literature is highly supportive of the Mako system’s accuracy when compared to conventional UKA, more evidence is needed in order to confidently evaluate the clinical benefits of each method.
NAVIO SYSTEM
Registration and Planning Procedure
The registration and planning process of the Navio system is fairly similar to the Mako system for UKA. However, instead of using a CT scan to generate a 3D model of the patient’s knee, a mapping procedure with an optically tracked pointer is used. Therefore, the Navio system is classified as an imageless system. Similar to the Mako system, optical tracker pins are fixed to the femur and tibia for spatial orientation, landmarks (rotation of the hip center, knee, and ankle) are registered, and the incision for the approach to the knee is made. The surgeon will then move the pointer over the bony surface of the hemi-tibial plateau and the femoral hemi-condyle (Fig. 41-7). This “painting” procedure allows the system to create a 3D model of the medial (medial UKA) or lateral knee (lateral UKA). A gap-balancing process comparable to the Mako system is then initiated to provide the surgeon with tracking and gap-distance information throughout the entire range of flexion (Fig. 41-8). Based on these data and lower limb alignment, implant size and position are determined by the system. Final adjustment can be made by the surgeon prior to bone resection for proper patient-specific kinematics (Fig. 41-9).
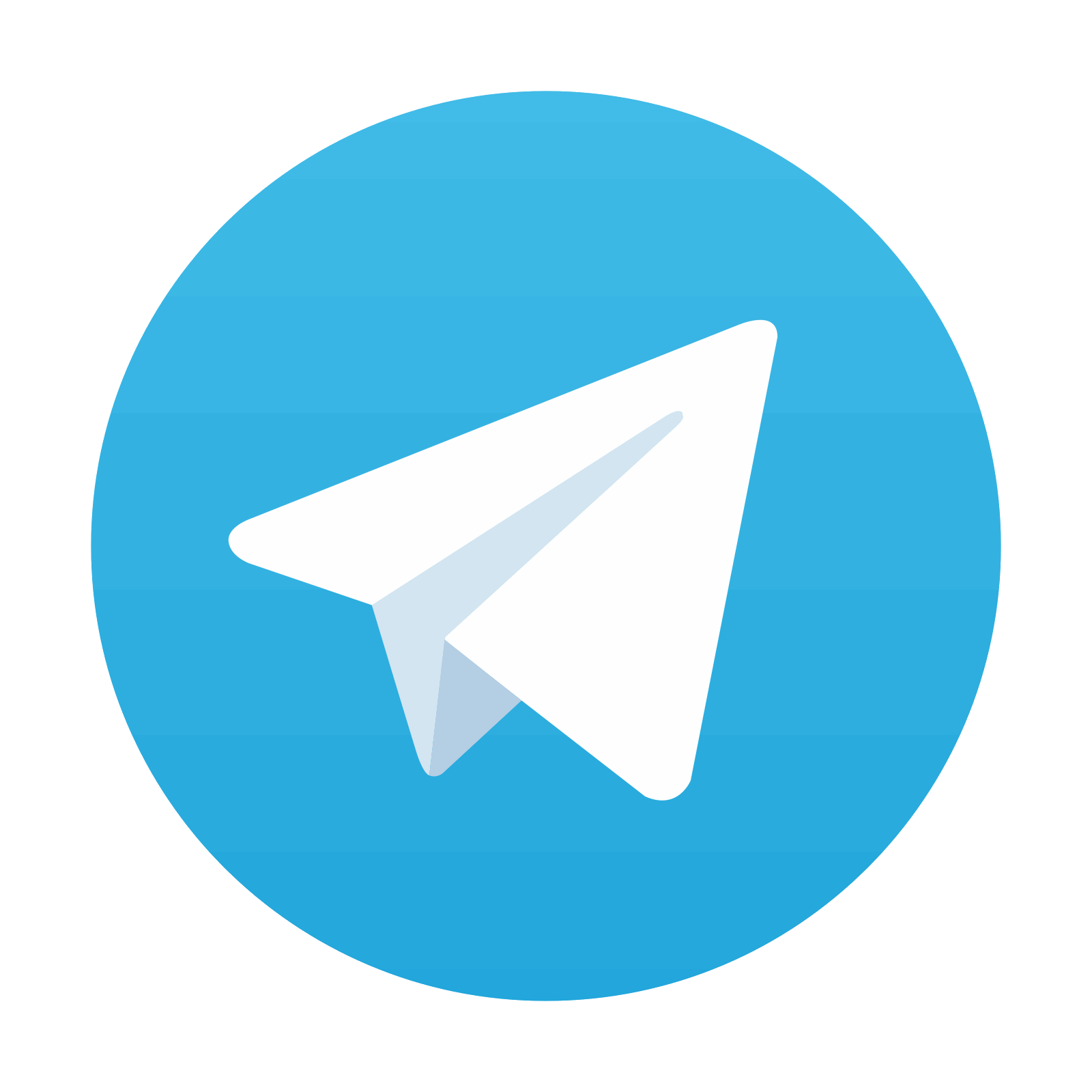
Stay updated, free articles. Join our Telegram channel

Full access? Get Clinical Tree
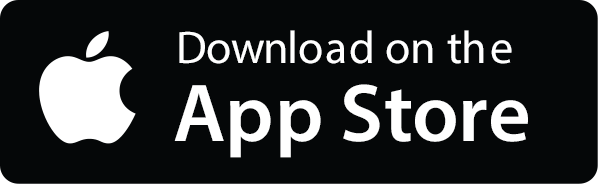
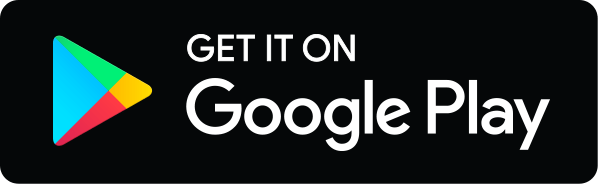