Key Points
- •
Approximately 90% of cases of hyperuricemia are caused by uric acid underexcretion from the kidneys. In addition, renal hypouricemia is caused by increased renal uric acid excretion. Thus, renal urate anion handling plays a major role in determination of serum urate level.
- •
The proximal tubule is the major site of renal urate anion transport, and this process is bidirectional, with reabsorption being predominant, and multiple transporters at apical and basolateral membranes playing significant roles.
- •
We propose that urate reabsorption in human proximal tubules is mainly performed by the “exchanger” URAT1 ( SLC22A12 ) at the apical membrane and the “facilitator” URATv1/GLUT9 ( SLC2A9 ) at the basolateral membrane in tandem, because there is in vivo evidence from human patient analysis that hypouricemia of renal origin has been caused by loss-of-function mutations of either transporter genes.
- •
Estrogen and androgens (via transcriptional effects), salicylates and nicotinate (niacin), and multiple potent uricosuric drugs (e.g., probenecid, benzbromarone) all influence specific mechanisms of renal urate anion transport in the proximal tubule.
Introduction
Humans have higher serum levels of urate, the ionized form of uric acid, than do other mammalian species due to evolutionary loss of the hepatic enzyme uricase that metabolizes relatively insoluble urate to ultimately generate highly soluble allantoin. Although uricase loss may have been beneficial to early primate survival by possibly providing antioxidant defense in the human body, sustained hyperuricemia has pathogenetic roles in gout and renal diseases as well as putative roles in hypertension and cardiovascular diseases. Since human serum urate (SUA) levels are largely determined by the balance between reabsorption and secretion of urate anion in the kidney, it is important to understand the molecular mechanism of renal urate handling. Although molecular identification of the kidney-specific urate/anion exchanger, SLC22A12 (URAT1), in 2002 paved the way for successive characterization of the physiologic roles of several urate transport-related proteins, the entire picture of effective renal urate handling in humans has not yet been clarified. Recently, several genomewide association studies (GWAS) have revealed substantial association between uric acid concentration and single nucleotide polymorphisms (SNPs) in at least 10 genetic loci related to uric acid metabolism including SLC2A9 (GLUT9/URATv1), ABCG2 (BCRP), and SLC17A3 (NPT4). In 2008, we functionally characterized the facilitatory glucose transporter family member SLC2A9 (GLUT9), one of the candidate genes for urate handling, as a voltage-driven urate transporter, URATv1, at the basolateral side of renal proximal tubules, which constitutes the main route of the urate reabsorption pathway, in tandem with URAT1 at the apical side. Recently, we found that the orphan transporter SLC17A3 (NPT4) functions as an apical exit path for both urate and drugs in renal proximal tubules with voltage-driven transport properties. Recent advances in research on renal urate transport and its significance in understanding SUA disorders are reviewed here. Reviews in the pre-GWAS era covered detailed information on renal urate transport. Several reviews that cover part of the present theme have also been published recently.
Urate Handling in the Kidney and Hyperuricemia
Uric acid is a breakdown product of ingested and endogenously synthesized purine nucleotides in humans and higher primates. Serum urate level is determined by interplay between the rates of production and elimination. Urate is produced primarily in the liver by xanthine oxidase, and two-thirds of it is excreted via the kidneys with the remaining third excreted into the gut. Therefore, in humans, increased uric acid production and/or reduced uric acid elimination causes hyperuricemia. Approximately 90% of hyperuricemia cases are caused by uric acid underexcretion from the kidneys. In addition, renal hypouricemia is caused by increased renal uric acid excretion. Thus, renal urate handling plays an important role in the determination of SUA level.
Urate is freely filtered through the glomerulus and it is almost completely reabsorbed, normally resulting in excretion of about 10% of its filtered load in humans. Thus, the presence of an effective renal urate reabsorption system in addition to the absence of uricase contributes to much higher basal SUA levels (about 300 μmol/L; about 5 mg/ml) in humans than in other mammals. The majority of studies indicate that the proximal tubule is the major site of renal urate transport. Bidirectional transport of urate, reabsorption being predominant, in proximal tubules was demonstrated by in vitro micropuncture and microperfusion studies in “urate reabsorber” species such as the rat, dog, and the Cebus monkey. In humans, it was suggested that urate is not only reabsorbed but also secreted as in other urate reabsorbers with a predominant reabsorption.
Historically, “the four-component model” ( Figure 4-1 ) was proposed for the mechanism of urate transport in human proximal tubules based on the hypothesis that pyrazinoate (PZA), an active metabolite of pyrazinamide, inhibits urate secretion. Since trans- stimulation of urate uptake via urate/anion exchanger SLC22A12 by PZA was demonstrated by Enomoto et al., the four-component model seems incomplete or inaccurate. Here, we propose a simple “three factor model” to explain renal urate handling related to the onset of hyperuricemia as shown in Figure 4-2 . We propose that urate reabsorption in human proximal tubules is mainly performed by the “exchanger” SLC22A12 (URAT1) at the apical membrane and the “facilitator” SLC2A9 (URATv1/GLUT9) at the basolateral membrane in tandem because there is in vivo evidence from patient analysis that renal hypouricemia was caused by loss-of-function mutations of either transporter genes. Recently, Kamatani et al. reported strong GWAs with urate to SNPs in SLC22A12 ( p = 2.34 × 10 –31 ) and SLC2A9 ( p = 7.09 × 10 –24 ) from about 14,700 Japanese individuals, replicating associations previously identified by GWA studies in European populations. In addition, luminal “facilitator” SLC17A3 (NPT4), which was also reported its association to hyperuricemia, has been proposed to function as exit pathways for urate reabsorbed by SLC22A12 because urate exit from the basolateral side is limited by the gatekeeper of urate, SLC2A9 . In this model, increased SLC2A9 function and/or decreased SLC17A3 function may induce hyperuricemia (see Figure 4-2 , B and C ).


Renal Urate Transporters
Urate Transporter/Channel LGALS9 (UAT)
The research group of Ruth Abramson screened rat cDNA using antibodies to pig liver uricase and isolated a protein comprising 322 amino acid residues. This protein, termed UAT (urate transporter/ LGALS9 ), was found in renal proximal tubules. When recombinant LGALS9 protein was reconstituted in planar lipid bilayers, membrane potential-dependent, channel-like currents evoked by urate were recorded. LGALS9 is for this reason regarded as a channel (urate channel) rather than a transporter. However, transport of urate by LGALS9 was not confirmed in a tracer experiment (RI-labeled urate), and at present it is unclear whether LGALS9 actually contributes to transmembrane transport of urate. LGALS9 is also hypothesized as being a 4-transmembrane domain protein, but this structure differs substantially from a typical transporter with 12-transmembrane domains. LGALS9 is expressed ubiquitously, not only in the kidney, and is hypothesized to efflux urate, produced by intracellular purine metabolism, outside cells.
Organic Anion Transporter (OAT) Family SLC22
Uric acid is a weak organic acid that dissociates H + under physiologic conditions and is present in the blood in the form of urate. This fact has encouraged speculation that urate may be transported by an organic anion/drug transporter. Excretion of organic anions and drugs via the kidneys is carried out by multispecific organic anion transporters (OATs) expressed in the renal tubules. Beginning with SLC22A6 (OAT1), the first organic anion transporter identified in the rat kidney by our research group in 1997, four isoforms of human organic anion transporter— SLC22A6 (OAT1), SLC22A7 (OAT2), SLC22A8 (OAT3),and SLC22A11 (OAT4)—were identified in the kidneys. These isoforms are expressed variously at the basolateral ( SLC22A6 to SLC22A8 ) or luminal ( SLC22A11 ) membranes in the proximal-to-distal tubules and serve to transport endogenous or exogenous (e.g., drug) organic anions. Oat1 – and Oat3 -null mice demonstrated decreased secretion rather than reabsorption, suggesting that OAT1 and/or OAT3 is involved in urate secretion in vivo. Kolz et al. conducted a meta-analysis of GWAS with a total of 28,141 participants of European descent and reported that SLC22A11 is one of the five new loci that influence uric acid concentrations. Hagos et al. reported that SLC22A11 (OAT4) takes up urate by exchanging OH – and suggested an involvement of SLC22A11 as a cause of hydrochlorothiazide-induced hyperuricemia, because urate uptake increases when cells are pretreated with thiazide diuretics. However, there has been no in vivo evidence that OAT4 is involved in renal urate transport—for example, no SLC22A11 mutation was found in renal hypouricemia patients and further analysis is necessary.
SLC22A12 (URAT1; urate transporter 1) is a member of the OAT family and is localized in the apical membrane of proximal tubular epithelial cells. Functional studies using a Xenopus oocyte expression system showed that URAT1 mediates the transport of urate in a time- and concentration-dependent manner ( K m about 370 μmol/L). The driving force for urate transport via URAT1 was shown to be exchange of luminal urate and intracellular inorganic (Cl – ) and organic anions (lactate, nicotinate [niacin], PZA), indicating that intracellularly accumulated organic anions will favor the uphill reabsorption of urate in exchange for these anions, which move down their electrochemical gradients into the lumen. Administration of benzbromarone for patients with hereditary renal hypouricemia caused by SLC22A12 mutation failed to show a stimulating effect, indicating that URAT1 is the site of action for an in vivo effect of benzbromarone.
Transport function of a mouse ortholog of SLC22A12 (mUrat1), or renal-specific transporter (RST), was also characterized. mUrat1 transports urate ( K m 1.2 mmol/L) and is cis -inhibited by probenecid, benzbromarone, and lactate. The fact that there are higher mRNA and protein levels of mUrat1 in male kidneys than in female kidneys may explain why the SUA level is higher in males than in females.
Facilitated Glucose Transporter Family SLC2A9
In 2007, Li et al. first reported that the glucose transporter family GLUT9 ( SLC2A9 ) is a gene correlated with the SUA level based on the results from a GWAS of a genetically isolated population in Sardinia. Similar results have been reported from several facilities. Vitart et al. also reported that SLC2A9 (GLUT9), initially reported as a fructose transporter, mediates the transport of urate. Human GLUT9 has two isoforms (GLUT9 and GLUT9ΔN) depending on the splicing of the intracellular part of the N-terminal region. When artificially expressed in polarized MDCK cells, GLUT9 (or long form) is expressed at the basal side, and GLUT9ΔN (or short form) is expressed at the apical side.
After the report by Li et al., we also evaluated the urate transport activity of SLC2A9 using a Xenopus oocyte expression system. We found that the SLC2A9 gene product is URATv1, a voltage-driven bidirectional urate transporter, which transports urate in a potential-dependent manner. In addition, since SLC2A9 is mainly expressed on the basolateral membrane of proximal tubular epithelial cells in the human kidney in vivo, we suggested that SLC2A9 (URATv1) is involved in the efflux of urate toward the blood side. Recently, we confirmed the voltage-dependent property of SLC2A9 -mediated urate transport by using the two-electrode voltage clamp method, since another facility had also shown that mouse Glut9 is involved in voltage-dependent urate transport. In 2009, Dinour et al. reported that homozygous SLC2A9 mutations (L75R) cause severe renal hypouricemia leading to exercise-induced acute renal failure. It is concluded that renal hypouricemia can be induced by mutation of not only SLC22A12 (URAT1), an apical influx transporter of urate, but also SLC2A9 (URATv1), a basolateral efflux transporter of urate. Therefore, at least two types of renal hypouricemia (i.e., SLC22A12 and SLC2A9 types) are likely to exist.
To investigate the effects of URATv1 gene overexpression in the kidney, we constructed SLC2A9 (human URATv1 ) transgenic (Tg) mice, in which the transgene was under the control of mouse Urat1 promoter that induces transgene expression in renal proximal tubules. We confirmed exogenous expression of human URATv1 only at the basolateral side of renal proximal tubules and found that SLC2A9 Tg mice showed reduced urinary urate secretion compared with that in wild-type mice, although SUA levels showed no change. In contrast, there is no phenotype in apical Urat1 Tg mice. Since almost all GWA studies have demonstrated that SLC2A9 has a strong association with SUA concentration, it is likely that the basolateral SLC2A9 is a gatekeeper to determine net urate transport in renal proximal tubules in vivo.
ATP-Binding Cassette ABC Transporters ABCC4 and ABCG2
MRP4 (multidrug resistance-associated protein 4; ABCC4 ), which exists at the apical membrane of renal proximal tubules, was reported to perform ATP-dependent urate anion excretion ( K m 1.5 mmol/L). MRP4 is proposed to function as another exit pathway for urate in urate secretion, although there is no clear in vivo evidence for these functions.
As mentioned earlier, Dehghan et al. proposed that, in addition to SLC2A9 (URATv1/GLUT9), ABCG2 (BCRP) and SLC17A3 (NPT4) are candidates for genetic causes of hyperuricemia. BCRP, breast cancer resistance protein ( ABCG2 ), a member of the same ABC transporter family to which ABCC4 (MRP4) belongs, was reported recently to transport uric acid. In addition, the finding that Q141K polymorphism associated with gout leads to decreased uric acid excretion capacity suggests that ABCG2 contributes to urate anion excretion at the apical membrane of renal proximal tubules and/or at the luminal membrane of the intestine. Although the human kidney is known to express many drug efflux pumps at the apical side of proximal tubules such as ABCB1 (P-glycoprotein/MDR1), ABCC2 (MRP2), and ABCC4 (MRP4), evidence for ABCG2 (BCRP) expression in the kidney has been somewhat confusing: according to one report, a moderate level of ABCG2 protein expression was detected despite no mRNA expression in the human kidney. Given its expression pattern (i.e., high levels in the liver and intestine), it is likely that the hypoactive variant of ABCG2 leads to decreased urate excretion into the intestine rather than (or as well as?) decreased uric acid excretion from the kidney.
Type I Na + -Dependent Phosphate Transporter Family SLC17A1 and SLC17A3
In 2000, Uchino et al. showed that human sodium-dependent phosphate transporter type 1 (NPT1) in the SLC17 family, present in renal apical membrane, mediates the transport of urate. Considering its chloride ion sensitivity, SLC17A1 (NPT1) was expected to function for secretion of organic anions from renal proximal tubular cells. Although Iharada et al. reported that SLC17A1 functions as a Cl – -dependent urate exporter only in proteoliposomes, we could not observe a voltage-dependency property for urate transport via SLC17A1 in Xenopus oocytes or in mammalian cell expression systems to date (P. Jutabha, N. Anzai, et al., unpublished observation).
In a genomewide study to determine genetic causes of hyperuricemia, Dehghan et al. found that SLC17A3 (NPT4) as well as ABCG2 (BCRP) are candidates in addition to SLC2A9 (URATv1/GLUT9). Human NPT4 (hNPT4) has two splice variants: hNPT4_L (long isoform) and hNPT4_S (short isoform). It has been reported that hNPT4_S lacking the fourth exon shows intracellular localization when expressed in COS cells. Recently, we found that the long isoform of the orphan transporter hNPT4 (hNPT4_L), expressed in the plasma membrane of Xenopus oocytes, mediated low-affinity transport of organic anions such as para -aminohippurate (PAH), estrone sulfate and urate in a voltage-sensitive manner. SLC17A3 mRNA expression was detected in the kidneys and liver. SLC17A3 was found to be localized at the apical side of renal tubules by immunohistochemistry, indicating that SLC17A3 functions as a voltage-driven urate efflux transporter. An in vivo role of SLC17A3 was suggested by two missense mutations in SLC17A3 with reduced urate efflux in vitro from hyperuricemia patients. These findings will complete a model of urate secretion in renal proximal tubular cells, in which intracellular urate taken up via SLC22A6 / SLC22A8 from the blood exits from the cells into the lumen via SLC17A3 .
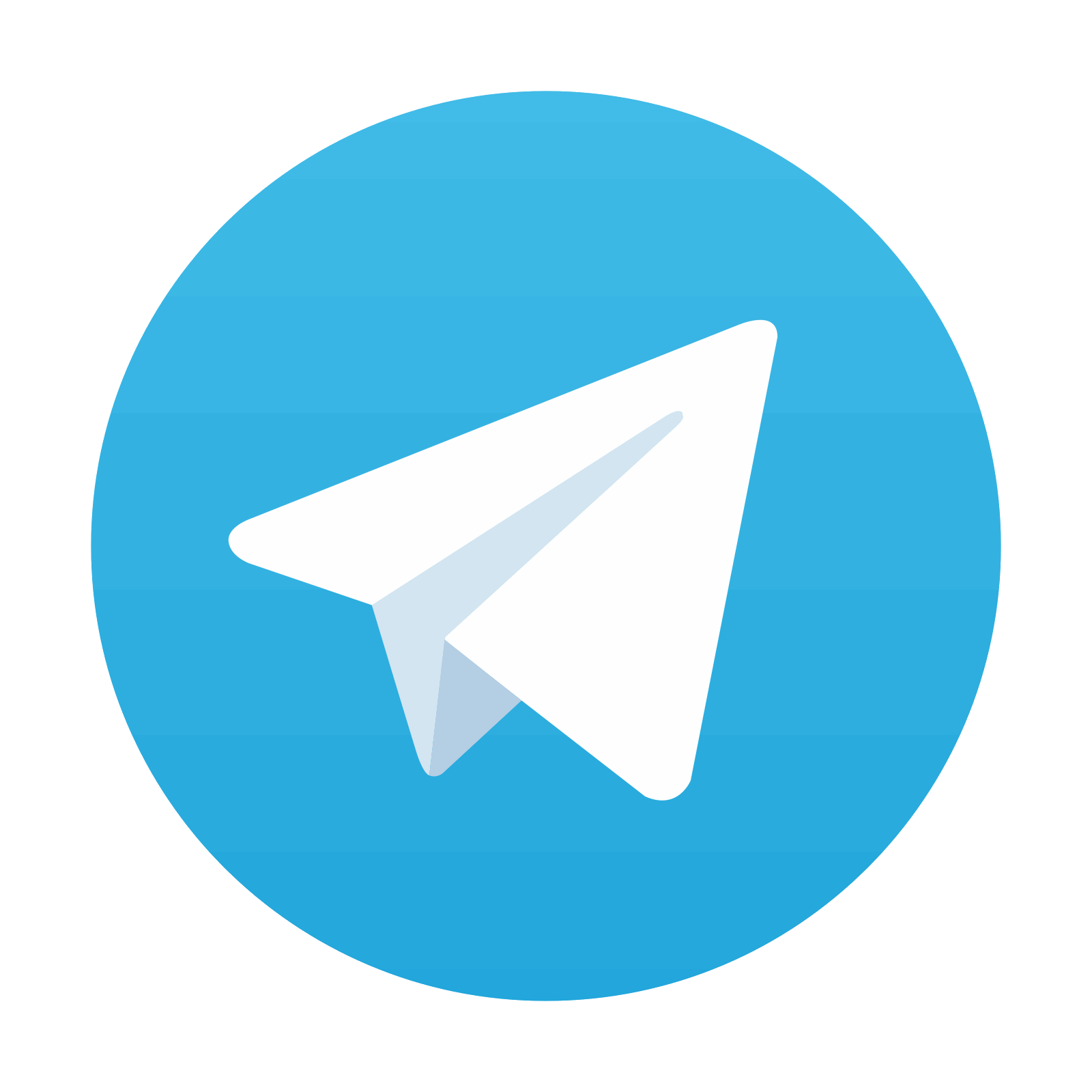
Stay updated, free articles. Join our Telegram channel

Full access? Get Clinical Tree
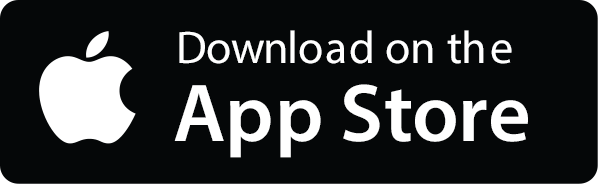
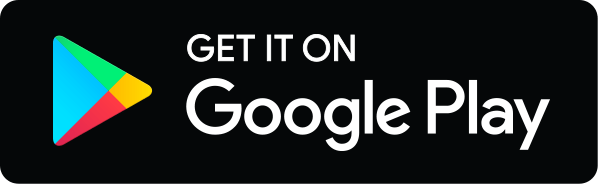