This chapter will examine traumatic brain injury (TBI) in the contexts of rehabilitation outcomes, systemic medical manifestations, and complications. The natural history of TBI will be reviewed in addition to multiple factors that may affect outcome. Additionally appropriate outcome measures will be analyzed. Common medical complications that occur during the rehabilitation of the TBI population will also be discussed. Finally, common issues in the medical management of TBI, including difficulties in diagnosis, provision of care, and research issues, will be reviewed.
The natural history of TBI is broadly characterized by an initial period of impaired consciousness, followed by a period of post-traumatic confusion/amnesia and finally a period of recovery of function. Most patients with mild traumatic brain injury recover within weeks to months without intervention, but approximately 15% of patients may still have symptoms at 1 year.1 Prognostic indicators of TBI include patient demographics (age, gender, prior TBI), initial injury details (initial severity, coma length, Glasgow Coma Scale [GCS], injury etiology), and rehabilitation course (length, timing, and intensity of rehabilitation). Outcome measures are divided into several domains, such as movement, cognition, and function.
Studies have demonstrated that older patients have worse outcomes than younger patients, likely secondary to decreased capacity for neuroplasticity in the aged brain and increased number of comorbidities (e.g., cardiovascular to cerebrovascular disease). Additionally, older TBI victims generally have been found to have higher rates of mortality.2 Older age at the time of injury has been shown to be associated with poorer performance in multiple cognitive domains.).3 Some studies also suggest that patients afflicted with TBI are more likely to develop neurodegenerative disorders such as Alzheimer’s disease.4 Mild TBI patients afflicted with post-traumatic stress disorder (PTSD) or depression have been found to have higher rates of comorbidities with related physical health problems.5 An example includes soldiers deployed in Iraq with PTSD and depression; when followed for 3 to 4 months after return home, this cohort reported higher rates of physical health problems (self-report of poor general health, missed workdays, and headache) for 3 to 4 months. When adjusted for covariates, interestingly only headache remained statistically significant.
Hypoxia and hypotension are independently associated with significant increases in morbidity and mortality from severe head injury. Hypotension is profoundly detrimental, occurring in 34.6% of these patients, and is associated with a 150% increase in mortality.6 Severe trauma to an extracranial organ system is the primary etiology of hypotension-induced brain injury in this population. Hypoxia and hypotension are common and lead to detrimental secondary brain insults in patients with direct traumatic brain injury. Hypotension in particular was found to be a major determinant of outcome in severe TBI.
Patients involved in motor vehicle accidents (MVAs) typically suffer more severe injuries at the onset of injury. At one year post-injury these patients have been found to have better functional and psychosocial outcomes when compared to patients who suffered violence-related TBI. Patients with violence-related TBI have significantly higher rates of unemployment and lower Community Integration Questionnaire scores. Individuals with TBI related to falls or “other” etiologies had outcomes that fell somewhere between victims of MVAs and assaults.7
TBI patients with penetrating brain injuries resulting from gunshot wounds (GSWs) have been found to have a high early mortality rate yet still have demonstrated good functional improvement after acute inpatient rehabilitation.8
Hospital admission GCS score positively correlated with functional outcome, whereas length of coma and duration of post-traumatic amnesia (PTA) correlated with both functional and occupational outcome. The highest functional recovery rates were found in the 8 to 16 and 17 to 40 age groups, while the poorest chance for a recovery was found in the youngest (<7) and oldest (>40) age groups.9
The Berg Balance Scale (BBS) is a 14-item scale designed to quantitatively monitor different aspects of balance (static and dynamic) in older patients.10 Scores less than 45 represent impaired balance.11 A weakness of this scale is that while there is a cutoff for impairment, there are no standards for interpretation of varying BBS scores.
The Community Balance and Mobility Scale (CBMS) is a 13-item scale designed by physical therapists to assess dynamic balance and mobility in patients with mild to moderate TBI.12 The CBMS may have increased sensitivity in the interpretation of variable scores.
The Agitated Behavior Scale (ABS) is a 14-item scale divided into three subscales (Disinhibition, Aggression, and Lability) designed to assess agitation in TBI patients.13 The ABS has been shown to demonstrate high internal consistency and inter-rater reliability.
The Functional Independence Measure (FIM) is composite measure comprising 18 items across six areas of function (self-care, sphincter control, mobility, locomotion, communication, social cognition) and provides an assessment of disability for individual patients, as well as in the context of medical reimbursement.14 Each item is scored on a 7-point scale that describes the degree of assistance required to perform the task, from total assistance (7) to independence (1). While widely used in rehabilitation centers in the United States, some disadvantages of this measure include the use of a single raw score that may give the false impression of the measure being a continuous, rather than ordinal, scale. Another is that the FIM only has five items devoted to assessing cognition and may be suboptimal for assessing TBI patients in whom cognitive impairments predominate.
The Functional Assessment Measure (FAM) was created to improve the relevance of the FIM described earlier for TBI patients.15 Twelve additional items focusing on cognitive, communicative, and psychosocial function were created.16 These items are orientation, attention, reading, writing, speech intelligibility, swallowing, emotional status, adjustment to limitations, employability, community access, car transfer, and safety judgment. Higher scores are associated with greater functional independence.17
The Glasgow Coma Scale (GCS) is a widely used 15-item scale with three categories (motor, verbal, and eye-opening) that was developed as an assessment of level of consciousness and coma (Fig. 21–1).18
A GCS score between 13 and 15 represents mild injury, a score between 9 and 12 represents moderate injury and a score of 8 or less represents severe injury.19 With regard to the domains of the GCS, it has been demonstrated that the motor response has the greatest influence on the summary score.20 The ability of the GCS score to predict survival is most highly associated with the motor response category.21 A significant limitation of the GCS includes limited assessment due to factors unrelated to primary brain pathology such as intubation, chemical sedation/intoxication, paralysis, etc.
The Glasgow Outcome Scale (GOS)/Extended GOS (GOSE) is one of the most widely accepted measures of outcome following TBI (Fig. 21–2).22
This scale uses a series of structured interviews to assign patients to a limited number of categories (dead, persistent vegetative state, severely disabled, moderately disabled, good recovery).23 In the Extended GOS, each of the categories higher than persistent vegetative state is expanded into an upper and lower band.24 The discrete number of categories simplifies administration and makes the scale broadly applicable. Conversely, individuals may make large improvements within a category that are not detected by the scale, as it is insensitive to more granular improvements.25
The Disability Rating Scale is an 8-item scale developed to better provide a quantitative overall assessment of impairment, disability, and handicap for TBI patients than the Glasgow Outcome Score, giving a “snapshot” of a patient’s general disability status.26 Four categories of function are assessed: (1) arousal, awareness, and responsivity; (2) cognitive ability for self-care activities; (3) dependence on others; and (4) psychosocial adaptability. No disability is scored as 0, and death is scored as 30. While simple to administer, the DRS is limited by suboptimal assessments of cognition and therefore poses a problem in the assessments of populations with very mild or severe TBI.
The Fatigue Severity Scale is a nine-question self-report questionnaire developed to assess fatigue by specifically probing for the connection between fatigue intensity and functional disability.27 Lower scores indicate less fatigue and higher levels of function; the efficacy and use in TBI patients have been validated.28
The Hospital Anxiety and Depression Scale (HADS) is a 14-question self-assessment scale that was developed to detect depression, anxiety, and emotional distress.29 Each question is scored on a scale of 0 to 3, with 3 indicating a higher symptom severity. The scale is divided into depression and anxiety subscales, with scores within the subscales divided into mild (8 to 10), moderate (11 to 14), and severe (15 to 21). Originally designed for use in hospitalized populations, the HADS has also been demonstrated to be a reliable measure of emotional stress following TBI, though impairments due to TBI may confound scoring.29
The Quality of Life after Traumatic Brain Injury (QOLIBRI) is a 37-item scale spread across six domains (cognition, self, daily life and autonomy, social relationships, emotions, and physical problems) that has been specifically designed to measure the quality of life of TBI patients.30 The total score is a quotient of the sum divided by the actual number of responses.
The Galveston Orientation and Amnesia Test (GOAT) is a 10-item measure that tests multiple components of orientation (time, place, person), as well as extent of post-traumatic and retrograde amnesia with a total possible score of 100. Patients with a score of less than 75 are considered to still have PTA.31 A patient is considered to have emerged from PTA once they score over 75 on two consecutive assessments.32 PTA is a significant independent predictor of functional outcome as assessed by DRS and FIM scores, and the length of PTA is significantly associated with employment status at one year postinjury.33 While providing objective measures for orientation, the requirement for oral or written expression may suboptimally characterize patients with impairments of communication.34
The Orientation Log (O-LOG) is a 10-item scale designed to quickly assess orientation at the bedside and was also designed to address some concerns with the GOAT; namely, that the GOAT was too heavily geared toward brain injury of traumatic etiology, as well as criticisms with regard to the differential weighting of items. The 10 items of the O-Log are city, kind of place, name of hospital, month, date, year, day of week, clock time, etiology/event (“What brought you to the hospital?”), and pathology/deficits (“What kind of injuries did you have?”), and each of these items is scored from 0 to 3, with 3 being the highest score.34
The JFK Coma Recovery Scale Revised (JFK-CRS) is a measure used to determine when a patient enters into and progresses beyond the minimally conscious state. The scale consists of six subscales that investigate the auditory, visual, motor, oromotor, communicative, and arousal functions of a patient with an altered level of consciousness. Evidence of purposeful activity with testing at any time indicates a patient has entered into the minimally conscious state35,36 (Fig. 21–3).
The Mini-Mental Status Examination (MMSE) is a widely used screening tool that provides a quick assessment of cognitive impairment. The MMSE consists of a 30-point test spread across multiple cognitive domains: orientation to time, orientation to place, registration of three words, attention, calculation, recall of three words, language, and visual construction. A total score indicating intact cognition is 30, with scores of 18 to 24 denoting mild cognitive impairment and 0 to 17 denoting severe cognitive impairment.37 Some concerns have been raised with regard to its sensitivity for patients with mild cognitive impairment, as well as its lack of items assessing visuospatial function.38
The Montreal Cognitive Assessment (MoCA) is a 30-point test designed as a rapid screening assessment for mild cognitive dysfunction.39 Compared to the MMSE, the MoCA notably has more items assessing visuospatial function (trails, cube copy, clock drawing) and fewer assessing linguistic function. It is also more difficult than the MMSE and more sensitive to mild cognitive impairments than the MMSE.40 It has been validated for detecting mild cognitive impairment (MCI) and early Alzheimer’s disease.
The Cognition Log (COG-LOG) is designed to be a quick quantitative measure of cognition for patients who have achieved consistent accurate orientation, as measured by scales like the O-LOG.41 The COG-LOG is a 10-item scale that encompasses domains ranging from orientation and attention to time estimation and rule learning. All items are scored from 0 to 3 for a total possible score of 30.
The Mayo-Portland Adaptability Inventory (MPAI-4) is a 35-item scale that is designed to evaluate the functional sequelae of TBI. The MPAI-4 is divided into three subscales (Ability, Adjustment, and Participation indices). Items within the scale are generally scored from 0 to 4, with 0 representing the most favorable outcome.42
The Neurobehavioral Functioning Inventory (NFI) is a 70-item scale divided across six functional domains: depression, somatic, memory/attention, communication, aggression, and motor.43 The scale was designed to assess the neurologic, behavioral, and psychological effects of brain injury.44 There has not been much work verifying construct validity for the inventory, with Asad demonstrating an inability of the scale to distinguish between TBI and controls.45
The Rancho Los Amigos Levels of Cognitive Functioning Scale (RLAS LCFS; Table 21–1) is a widely used, 10 (originally 8)–level scale that describes the typical course of cognitive recovery that TBI patients progress through.46 While not initially developed as a scale and not generally considered an outcome measure, it does provide a widely recognized index of awareness, interaction, and behavior and is used to classify outcomes in TBI patients.47,48
Ranchos Los Amigos Scale Level I: No Response Level II: Generalized Response Level III: Localized Response Level IV: Confused-agitated Level V: Confused-inappropriate Level VI: Confused-appropriate Level VII: Automatic-appropriate Level VIII: Purposeful-appropriate |
Trauma to the brain can result in significant pathology. Typically, individuals identified as having experienced a TBI are initially evaluated in an emergency room and, along with a clinical examination, undergo immediate neuroradiological assessment (usually with a noncontrast head computerized tomography [CT] scan). Those with identified brain pathology on CT scans are typically admitted to a trauma or neurologic/neurosurgical unit for close observation and needed interventions. Common pathologies associated with TBI are discussed in this section.
Brain contusions, or bruises of the brain, occur due to the impact of the brain against the bony ridges of the skull and are detected on head CT and magnetic resonance imaging (MRI) as areas of localized hemorrhages and/or different attenuation as compared to the rest of the brain. Two types of injury leading to contusions are coup and contre-coup injuries: coup injuries refer to damage caused by the initial impact of the brain against the skull; contre-coup injuries occur at the opposite side of the brain as a result of the brain rebounding against the skull. These contusions manifest in a wide variety of neurologic and behavioral dysfunction, depending on the area of the brain affected. The most common area affected in TBI is the lower frontotemporal region of the brain due to the bony anatomy at the base of the skull.
Diffuse axonal injury (DAI) results in shearing forces on the brain from acceleration-deceleration and rotational forces that are often associated with high-velocity impact, such as those occurring in a motor vehicle accident and/or blast injury. These shearing forces disrupt nerve cells in the brain, especially where the more freely moving portion meets the more fixed portion of the brain. DAI is a common underlying reason for the abrupt onset of neurologic deficits in a significant proportion of patients with TBI. Patients can present with variable severity of cognitive deficits, from those detectable only under stressful situations to altered levels of consciousness, depending on the severity of injury. CT and MRI of the brain may reveal microhemorrhages in select areas of the brain (i.e., the corpus callosum, central white matter, and midbrain), but many times there are no discernible findings on imaging. The diagnosis of TBI is therefore often based on clinical examination and subjective complaints of new-onset neurologic symptoms, especially in those with mild injuries.
Intracranial hemorrhages or hematomas are caused by bleeding underneath the skull from ruptured blood vessels. They are most commonly described by the layer of the brain within which the bleeding occurs. The brain is lined by three layers of tissue: the dura mater, pia mater, and arachnoid mater.
Epidural hematomas are collections of blood between the skull and the outermost layer of the brain called the dura mater. This type of hematoma grows rapidly in size and can lead to death within a matter of hours if untreated. The presence of a lucid interval, where the patient appears to recover from the initial trauma for a short period soon after the event, often confuses the clinical presentation. In these situations, the patient’s level of arousal rapidly declines after this brief lucid time interval.
Subdural hematomas are collections of blood between the dura and pia mater layers of the brain. These bleeds are usually slower growing, and if they grow slow enough, they can increase for weeks or even months before obvious clinical symptoms of dysfunction are noted.
Subarachnoid hematomas occur between the pia mater and the arachnoid membrane, the closest lining surrounding the brain. They often develop as a result of blood vessel abnormalities (i.e., rupture of an arteriovenous malformation or saccular aneurysm) but also occur as a result of trauma. The classic presentation is that of the patient describing “the worst headache of my life” followed by a sudden loss of consciousness.
Intracerebral or intraventricular hematomas are collections of blood within the brain or ventricles of the brain itself. As with the other hemorrhagic injuries, the patient can develop subsequent neurologic deficits and can present with symptoms of increasing headaches, visual changes, nausea, vomiting, dizziness, confusion, weakness, and difficulties with balance, and ultimately loss of consciousness and death. Although intracranial hemorrhages can lead to ischemia due to decreased blood supply to parts of the brain, pressure on the brain as the hematoma grows is usually the more life-threatening and devastating cause of neurologic decline. In cases of ischemia where there is cell death, treatment is aimed at reducing the extent of surrounding swelling and inflammation in an attempt to preserve nerve function.
Skull fractures themselves do not usually cause neurologic deficits, but the potential complications associated with certain fractures can be significant. Depressed skull fractures are associated with worse neurologic deficits and outcomes as opposed to nondepressed fractures; any open injury will increase the risk of infection, as mentioned earlier. Temporal bone fractures pose an increased risk of epidural hematomas due to the vascularity of the area. Basilar skull fractures can damage the facial, acoustic (i.e., hearing), and vestibular (i.e., balance) nerves.
Cranial nerve damage can occur following more severe TBIs. The type of injury, such as compressive versus direct insult to the nerve, will also affect the nature of the deficit, the most appropriate treatment, and the prognosis for recovery. The most common cranial nerve injury after a TBI is to the olfactory nerve (cranial nerve I) leading to anosmia (i.e., a loss of the sense of smell and possible alterations in taste).49 A lack of awareness of this deficit can potentially lead to significant safety risks (e.g., the patient fails to detect smoke or a gas leak in the home). Depending on the nerve injured, cranial nerve damage can result in other deficits such as monocular blindness, diplopia, visual field deficits, blurring, blind spots, paralysis of the eyes, ptosis of the eyelid, abnormal dilation of the pupil, numbness of the face, decreased salivation, corneal drying, paralysis of the face, hypersensitivity to sound, ringing in the ears, hearing loss, positional vertigo, autonomic system dysfunction, tongue dysfunction, swallowing and speech difficulties, and shoulder muscle dysfunction.
TBI patients are often prone to circulatory, digestive, and respiratory problems and are commonly diagnosed with neurologic issues such as endocrine problems, seizures, and swallowing difficulties.50
Increases in intracranial pressure (ICP) due to cerebral edema, intracranial fluid collection, or bleeding may cause compression of brain structures, reduce cerebral blood perfusion leading to cerebral ischemia, or cause brain herniations through the skull (Fig. 21–4).
Figure 21–4
Neuroimaging and intracranial compliance. Head imaging does not replace ICP monitoring; however, some estimates of intracranial compliance can be obtained. The head CT (A and B taken at 48 hours post-event) identifies right middle cerebral artery ischemic infarction with local mass effects; however, there are remaining compressible CSF spaces (ventricular system, basal cisterns) visible indicating reduced but not exhausted intracranial compliance. With further mass effects and tissue shift (C and D; CT taken at about 96 hours) almost complete compression of neighboring CSF spaces and exhausted intracranial compliance is identified in addition to evolving herniation-induced (right to left subfalcine herniation) led to new right anterior cerebral artery infarction (arrowhead in D). The estimated, relative intracranial compliance for the obtained head CT scans is delineated on the graph (E).

Physicians can detect elevated ICPs by finding papilledema on exam, evidence of brain compression on head CT scans, or elevated pressures with a lumbar puncture or ICP monitoring device. Clinically, a reduction in the patient’s level of consciousness may occur, with an elevated ICP ultimately resulting in death if untreated. Establishing an airway for breathing or mechanical ventilation and restoring adequate blood flow to the brain are the first steps in medical management, with intracranial surgery often necessary. In cases where neurosurgical intervention is not needed immediately, declines in cognitive or neurologic functioning on repeat screening or evidence of worsening findings on serial head CT imaging may still occur. These findings are suggestive of delayed neurologic compromise, and intracranial surgery may ultimately be needed to limit secondary brain injury.51
Post-traumatic hydrocephalus (PTH) is caused by blockage of normal cerebrospinal fluid (CSF) flow, overproduction of CSF, or insufficient absorption of CSF back into the body. If PTH is left untreated, there is an increased risk of morbidity and mortality.52 Symptoms associated with PTH consist of incontinence, ataxia, gait disturbances, and dementia. The first symptoms of PTH can also be intermittent headache, vomiting, confusion, and/or drowsiness. A doll’s eye maneuver may demonstrated bilateral sixth nerve palsies, which is seen in hydrocephalus (Fig. 21–5).
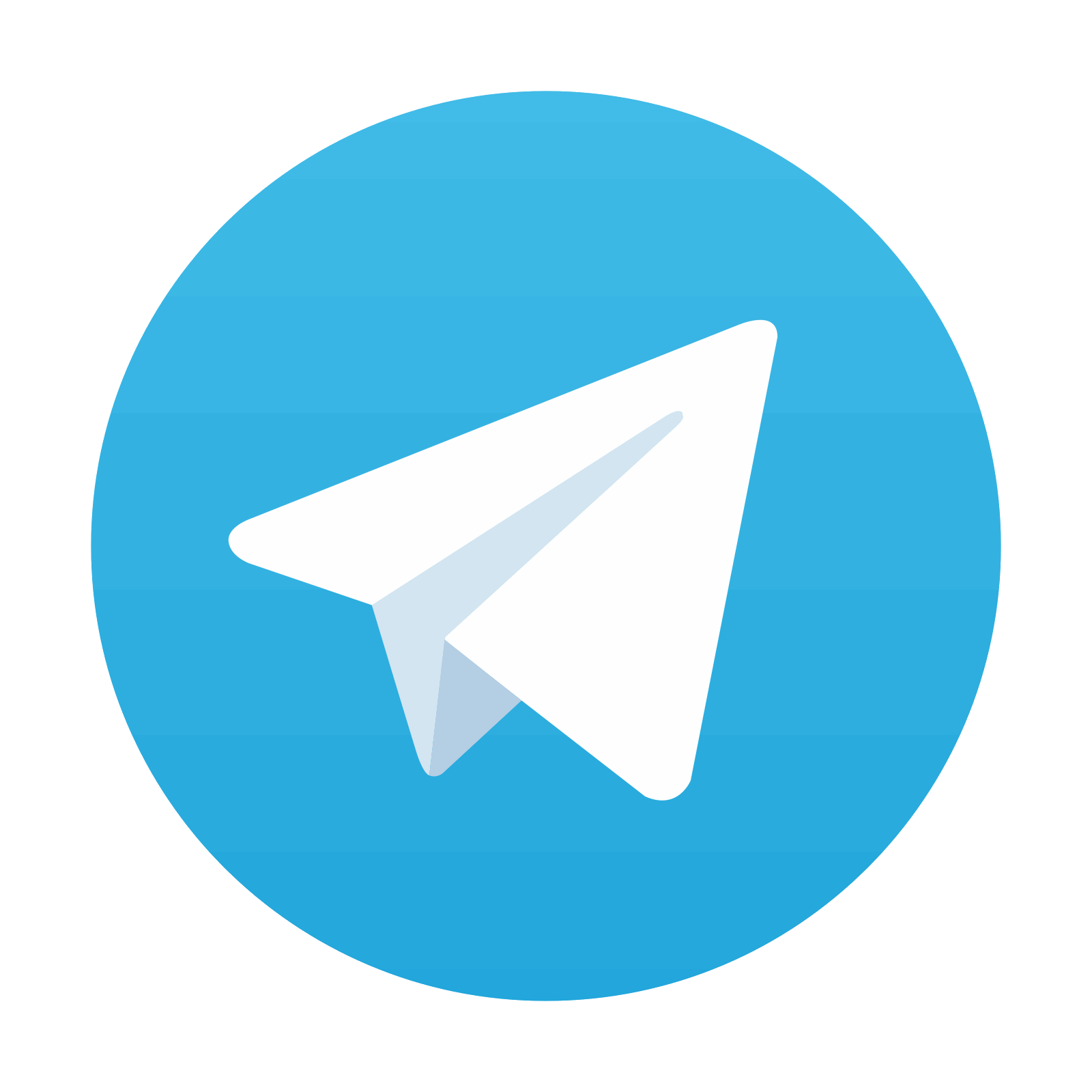
Stay updated, free articles. Join our Telegram channel

Full access? Get Clinical Tree
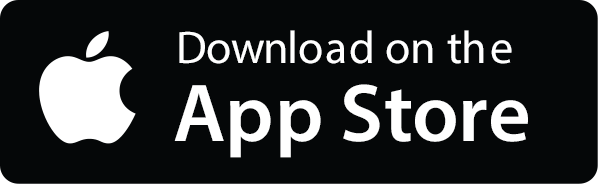
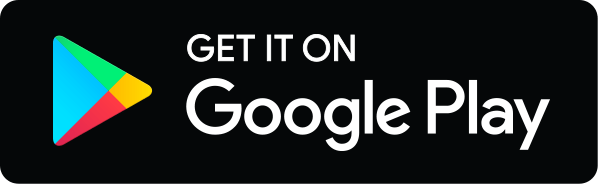