Introduction
Children with multiple injuries present unusually stressful challenges to their families and the multidisciplinary medical and surgical teams that care for them. The goal of the acute care of the multiply injured child is to allow the child to transition to the rehabilitation phase of care as rapidly as possible. Pediatric rehabilitation services have been shown to be beneficial to a broad spectrum of pediatric trauma patients (both with and without significant head injuries), and significant gains have been documented in mobility, self-care, and cognition. More rapid transitions from acute care to rehabilitation services have been correlated with better functional outcomes. The purpose of this chapter is to outline important aspects of both the inpatient and outpatient rehabilitation treatment of the child with multiple injuries.
Pediatric Polytrauma
Dedicated pediatric trauma centers have repeatedly demonstrated positive effects on the care of pediatric trauma patients. ∗
∗
Significant permanent deficiencies are common in these patients, up to half of whom have long-term sequelae from their injuries. The most likely type of major operative procedures that pediatric trauma patients will require are orthopaedic in nature. However, both short-term and long-term outcomes after pediatric polytrauma are driven largely by the severity of associated neurologic injuries. ††
Inpatient Rehabilitation
The goal of inpatient rehabilitation of the pediatric trauma patient is to enable him or her to make rapid progress toward functional recovery and transition to outpatient rehabilitation. Many factors determine the need for inpatient rehabilitation. The rehabilitation team should document the child’s previous level of function, the current level, and the potential to improve with intensive therapies. The rehabilitation team should ideally consist of a team leader, usually a physiatrist, a physical therapist, an occupational therapist, and a speech therapist. Patients who have deficits in mobility, function, cognition, and/or speech are good candidates for inpatient rehabilitation. Patients must be medically stable and able to participate in 3 hours per day of therapy. Usually, they must need at least two different therapies, such as physical therapy, occupational therapy, speech therapy, or cognitive therapy, for insurance companies to approve inpatient rehabilitation. Communication between the rehabilitation team and the orthopaedist is important. Weight-bearing status and the permissibility of passive range-of-motion (ROM) exercises versus active and active-assistive ROM exercises must be determined from the orthopaedics department before admission to inpatient rehabilitation because it could significantly affect therapies. Both upper and lower extremity weight-bearing status should be documented, even if no lower extremity orthopaedic injuries are present. This is because a patient may be able to compensate for weakness caused by a brain injury or spinal cord injury (SCI) by weight-bearing through the upper extremities with an assistive device. If a child is non–weight bearing on more than two limbs it may be worthwhile to delay rehabilitation until weight-bearing status has progressed. Patients do not have to be completely healed, but the more stable they are the more they can benefit from a full rehabilitation program. It is important that patients be able to follow at least simple commands to be able to fully participate in therapies. However, if there are exceptional family needs, sometimes a brief inpatient stay is beneficial for family teaching, even if the child is not able to fully participate.
Rehabilitation of Fractures
Therapeutic Exercise
Once the period of immobilization is over, the work of regaining ROM and strength and returning to full activity and participation begins. Table 20-1 lists the full ROM and the functional ROM of mature joints. They are listed with measurements standardized by the Committee for the Study of Joint Motion of the American Academy of Orthopaedic Surgeons. ROM is measured as degrees of deviation from the anatomic position. The ranges given in Table 20-1 are for mature limbs. Very young children should be evaluated by someone familiar with pediatric norms. For example, infants have a physiologic hip flexion contracture, normal hip extension that averages −30°, and increased external rotation at the hip. These “contractures” resolve slowly as the infant matures.
JOINT | DEGREES NORMAL ROM (FUNCTIONAL ROM) |
---|---|
Shoulder | |
Abduction | 180 (120) |
Adduction | 45 (30) |
Flexion | 180 (120) |
Extension | 60 (40) |
Internal rotation (arm in abduction) | 80 (45) |
External rotation (arm in abduction) | 90 (45) |
Elbow | |
Flexion | 140–160 (130) |
Extension | 0–5 (−30) |
Supination | 80–90 (50) |
Pronation | 70–80 (50) |
Wrist | |
Flexion | 75 (15) |
Extension | 70 (30) |
Radial deviation | 20 (10) |
Ulnar deviation | 35 (15) |
Hip | |
Flexion | 125–128 (90–110) |
Extension | 0–20 (0–5) |
Abduction | 45–48 (0–20) |
Adduction | 40–45 (0–20) |
Internal rotation | 40–45 (0–20) |
External rotation | 45 (0–15) |
Knee | |
Flexion | 130–140 (110) |
Extension | 0 (0) |
Ankle | |
Plantar flexion | 45 (20) |
Dorsiflexion | 20 (10) |
Inversion | 35 (10) |
Eversion | 25 (10) |
Exercise to increase ROM may be active (movement generated by the patient) or passive if the child is too young, too weak, or unable to cooperate. Active-assistive ROM exercise consists of having the child actively move through as much of the range as possible and then passively completing as much movement as possible through the normal range. Active ROM exercises are generally preferred, if possible. The use of body weight can sometimes increase the effectiveness of ROM exercises, for example, with stretching of the gastrocnemius muscles. These powerful muscles are capable of lifting the entire body weight and are commonly shortened after a period of injury and immobility. Unless the child is small, passively ranging these muscles is difficult, and the best stretch is obtained when the patient is standing. Every effort should be made to encourage children to participate in their ROM exercise program. Older children can often complete their exercise programs quite independently. Even young children may be able to perform simple stretches if properly supervised.
Strengthening exercises may be indicated when bone healing is well established. These exercises usually involve extremely simple equipment such as a ball, putty, or exercise band. More formal strengthening programs may include isometric exercises, which maintain muscle length with increasing tension during contraction, or isotonic exercises with muscle shortening and steady tension. Isotonic exercises are further subdivided into open kinetic chain exercises, such as with free weights, or closed kinetic chain exercises, such as with pulley systems. Closed kinetic chain exercises are often favored in the early postinjury period because they provide a more predictable path of movement for the exercising limb. For athletes, evaluation by a therapist familiar with the specific motions involved in the sport (e.g., cutting or jumping) will help identify areas of weakness that may lead to reinjury. Return to play should not be allowed until full pain-free strength and ROM have been restored.
Evaluation of strength is by manual muscle testing, and muscle strength is graded on a 0 to 5 scale.
0 = No contraction
1 = Palpable or visible contraction
2 = Full ROM with gravity eliminated
3 = Full ROM against gravity
4 = Full ROM against moderate resistance
5 = Full ROM against full resistance
Interventions with Physical Agents
Heat and cold may be used in the rehabilitation of fractures. Generally, cold is used early to reduce swelling and inflammation. It is rarely indicated in a patient with severe trauma and is most appropriate for isolated, minor low-energy fractures. In the rehabilitation phase, direct heat from a warm water bath or hot packs warms the skin and may improve comfort. Other measures such as ultrasound, short-wave diathermy, and microwave diathermy are seldom used in children. Ultrasound is contraindicated over active apophyseal or epiphyseal regions.
Orthoses and Adaptive Equipment
Good communication between rehabilitation and acute care trauma specialists is essential for determining which orthoses are necessary for fracture healing and which are used solely to improve function and may be discarded if they are no longer useful. Generally, orthoses to improve function are not ordered until the end of a rehabilitation stay or even at outpatient follow-up, when the patient’s pattern of weakness and muscle tone are well established. Lower extremity orthoses are commonly prescribed for abnormal gait dynamics after brain injury or SCI. They are named according to the region of the body that they help control. For example, supramalleolar orthoses begin underneath the foot and wrap around the medial and lateral malleoli, allowing free ankle dorsiflexion and plantar flexion. Ankle–foot orthoses extend from underneath the foot to above the ankle to limit plantar flexion. Knee–ankle–foot orthoses extend from below the foot to above the knee. Generally, the more joints controlled by the orthosis, the bulkier it becomes and the greater the potential to interfere with balance and gait.
For patients with extremity fractures, adaptive devices can be helpful in extending reach, providing grasp, reducing force, and improving safety. Such devices can provide increased independence in basic activities of daily living, including feeding, dressing, bathing, grooming, and toileting. Examples include reachers and sock aids ( Fig. 20-1 ), a long-handled sponge or shoe horn, grooming aids, built-up feeding utensils, a rocker knife, a raised toilet seat, tub chair, and built-up door handles. Velcro or elastic shoe closures may be helpful. Evaluation by an occupational therapist for problem solving and individualized recommendations may prove invaluable in increasing independence and improving quality of life during recovery for both injured children or adolescents and their parents and other caregivers.

Gait aids may be useful for a child with weight-bearing restrictions on the lower extremity. When used with a “swing-through” gait pattern, crutches can completely eliminate weight-bearing on the affected limb. Some initial instruction with crutches is needed to prevent axillary trauma and to ensure that these children are able to understand their limitations. A child who requires additional support because of instability or reduced lower extremity strength may require a walker. A walker or crutches can be modified with an upper extremity platform support to distribute the weight through the elbow and along part of the forearm, and such support should be considered in children with concurrent wrist or hand injuries. Wheelchair use should be encouraged if both lower extremities are involved or the patient cannot comply with weight-bearing restrictions. Gait aids should be prescribed by someone familiar with not only the child’s function but also any cognitive or balance limitations.
In the case of a child with multiple fractures but no central nervous system involvement, a short stay in a rehabilitation unit may still be helpful for provision of adaptive equipment to increase independence in activities of daily living and instruction of the patient and family in safe transfer techniques and wheelchair safety skills. Devices such as a sliding board may be helpful in increasing a child’s independence in transfers. At least two limbs must be able to bear weight for patients to participate in their own transfer. If a patient is not weight-bearing on three or four limbs, caregivers should be instructed on safe transfers and the use of a lift, if needed.
Pain Control
Adequate pain control in children after a traumatic injury is an issue that is gaining increasing attention. The subject of most investigations is acute pain control during procedures such as fracture reduction in the emergency department. However, for a multiply injured child, pain may be an ongoing problem, even during the rehabilitation phase. Patients who are uncommunicative because of age or neurologic insult and in whom unexplained agitation or vital sign changes such as tachycardia or hypertension develop should be carefully evaluated for evidence of additional painful injuries before ascribing the changes to other factors, including the natural history of concurrent brain recovery or autonomic instability. Neuropathic pain is common in SCIs, especially incomplete injuries, but can also occur after trauma or surgical procedures. Gabapentin can be very helpful in controlling neuropathic pain. Dosing is based on the dose for seizures; however, the dose often does not need to be as high to control pain. In a child 3 to 12 years of age, it is recommended that 10 to 15 mg/kg/day, divided three times a day (tid), be started initially and increased every 3 days to a maximum of 50 mg/kg/day. In a patient older than 12 years, the maximum dose is 3600 mg/day. Gabapentin may cause sedation and also has abuse potential. Abrupt withdrawal of the medication should be avoided because of the risk of seizures. If the medication is to be discontinued, it should be tapered over 7 days. The package insert should be reviewed for complete details.
Observational scales such as the Children’s Hospital of Eastern Ontario Pain Scale and the Objective Pain Scale are primarily used in infants and very young children. For children 3 to 6 years of age, visual progression of happy to sad faces is frequently used. Examples include the McGrath Facial Affective Scale and Beyer’s Oucher Scale.
Providing a description of their pain is difficult for preschool-age children who do not possess the language ability or the understanding to correctly identify the location and characteristics of the pain. Older children have improved abstract and numeric reasoning and may be able to use more adult measures such as the visual analog scale.
In the rehabilitation setting, the goal is to slowly wean the child from opioid analgesics while managing side effects such as constipation and urinary retention. When a patient has been taking opioid analgesics for a long period, weaning is often better tolerated when the medication is first changed to a longer-acting agent, which is then slowly tapered.
Peripheral Nerve Injury And Electrodiagnosis
Screening for evidence of peripheral nerve injury is part of routine care after skeletal injuries in children. Nerve injury can occur both as a consequence of the initial injury and as a complication of fracture stabilization.
Clavicular fractures may be associated with brachial plexus injuries. Brachial plexus injuries are subdivided into upper plexus injuries (C5–C6), or those above the clavicle, and lower plexus injuries (C8–T1), or those below the clavicle. The mechanism of injury may give a clue to the location of the lesion even before the physical examination is performed. For instance, the upper plexus is vulnerable in neck traction injuries. The classic example is a newborn with shoulder dystocia in whom Erb palsy develops because of traction on the neck. The lower plexus may be injured by extreme abduction of the arm, which produces a classic Klumpke palsy. Sports injuries may include both mechanisms, and weakness is produced at multiple root levels. Generally, recovery from a traction injury is good, but the presence of cervical nerve root avulsion leads to a much poorer prognosis.
Lumbosacral plexopathy is a concern after pelvic fractures. The lumbosacral plexus is usually well protected from trauma because of its posterior location and proximity to the pelvic bones. However, trauma severe enough to produce an unstable pelvic fracture, retroperitoneal hematoma, or fracture–dislocation of the hip joint may also affect the plexus. The injury may be subtle, so a high index of suspicion for peripheral nerve damage needs to be maintained in pelvic trauma.
A supracondylar fracture of the humerus, particularly if displaced, can lead to injury to the anterior interosseus, radial, median, or ulnar nerves alone or in combination. The estimated incidence of nerve injury after a supracondylar fracture varies from 6% to 16%. Because the anterior interosseous nerve lacks sensory distributions, injuries to this nerve may be overlod. Median nerve injury is a rare complication of a distal radial fracture. An estimated 5% of children with traumatic fracture or dislocation of the hip sustain nerve injury, usually to the peroneal branch of the sciatic nerve.
Peripheral nerve injury does not always occur immediately. Damage to the growth plate or angulation at the level of the joint may lead to a delayed nerve injury. An example is the angulation deformity at the elbow leading to later ulnar nerve injury.
Greater long-term disability may result from the complicating nerve injury during skeletal trauma than from the skeletal injury itself. Peripheral nerve injuries are divided according to Seddon’s classic work in neurapraxia, axonotmesis, and neurotmesis. Neurapraxia is nerve compression or ischemia with subsequent segmental demyelination. No disruption of the axon occurs, and the prognosis for complete return of function is excellent. Recovery usually occurs rapidly within weeks, except in the case of chronic entrapment, which leads to permanent damage. Axonotmesis involves disruption of the axon with maintenance of intact supporting structures. Wallerian degeneration of the distal segment ensues. Resolution of the injury is variable and slow and depends on nerve sprouting and regeneration. Neurotmesis is the most severe injury, with disruption of both the axon and supporting structures. Without intact supporting structures, nerve sprouting and regeneration are inefficient and often misdirected, which sometimes leads to inappropriate innervation. The prognosis for recovery is poor, and surgical intervention may be indicated.
Recovery after peripheral nerve injury in children is faster and more complete than in adults. In adults after complete resection and anastomosis, peripheral nerves regenerate at a rate of 1.5 to 2.0 mm/day. Maximal recovery can take up to 40 months. Perhaps because of a combination of the shorter length of children’s limbs and faster healing, pediatric recovery is maximal in 13 to 19 months. Nerve injuries that fail to demonstrate healing after 5 months may require surgical intervention. In cases in which the location or severity of injury is uncertain, electrodiagnostic studies may provide valuable diagnostic and prognostic information.
Most electrodiagnostic studies include nerve conduction studies and electromyography (EMG). In nerve conduction studies, an electric impulse is transmitted across a sensory or motor nerve, and the resulting response is picked up by recording electrodes. In motor studies, the response is called the compound motor action potential (CMAP), whereas in sensory nerve conduction studies, the sensory nerve action potential (SNAP) is recorded. Timing is important. In the first week after a nerve injury, wallerian degeneration has not occurred. If a nerve is stimulated proximal to the lesion and the recording electrode is placed distal to it, the CMAP or SNAP decreases significantly. However, if the nerve is stimulated distal to the lesion, the CMAP and SNAP are within normal limits. This phenomenon is referred to as conduction block. It can be very useful for localization of a nerve injury. However, distinguishing the type of nerve injury electrodiagnostically is difficult in the first week after injury.
Wallerian degeneration occurs within 7 to 11 days. Determination of the location of the lesion becomes more difficult, but estimation of the severity of the nerve injury becomes easier. Neurapraxic lesions (without any wallerian degeneration) may demonstrate a conduction block if segmental demyelination is significant, or nerve conduction studies may be normal. In axonotmesis and neurotmesis, the distal nerve segment, which has been severed from the axon, degenerates. CMAP and SNAP are uniformly low in affected nerves, regardless of the location of stimulation.
By 2 to 3 weeks after injury, EMG shows evidence of denervation in affected muscles, along with muscle membrane instability in the form of positive waves and fibrillations. The distribution of denervation may be useful for localization of the lesion. For instance, footdrop after hip surgery may be due to damage to the peroneal branch of the sciatic nerve at the hip or compression palsy of the common peroneal nerve at the fibular head. Differentiation between the two sites on clinical grounds may be difficult in a postsurgical patient. The short head of the biceps femoris is innervated by the peroneal nerve before it crosses the fibular head. Involvement of this muscle is more consistent with sciatic nerve injury.
Electrodiagnostic studies can be difficult in young and uncooperative children. Children younger than 2 years have different normal values for nerve conduction studies because of the immaturity of the myelin sheath. For these reasons, young children should be evaluated by an electromyographer familiar with the special issues related to electrodiagnosis in children.
Spinal Cord Injury
SCI is an uncommon complication of pediatric skeletal trauma and occurs in only 2% of reported cases of pediatric trauma. Although this complication is rare, it can be devastating. It is also easily overlooked because of a phenomenon that occurs primarily in children—spinal cord injury without radiographic abnormality (SCIWORA). This phenomenon is possible because of the flexibility of the pediatric spinal canal, which can stretch up to 2 inches in infants. However, the spinal cord will tear after being stretched just 0.25 inches. Children with this condition will have no abnormalities on plain radiographs, but their injuries may be detectable on magnetic resonance imaging. An estimated 16% to 19% of SCIs in children are classified as SCIWORA.
Because almost one in five children with SCIs have no abnormalities on plain radiographs, careful clinical examination in any child with a suspected neck injury is indicated. The American Spinal Injury Association (ASIA) has published a guide to the examination of persons with SCIs. Grading involves strength testing in key muscle groups and evaluation of sensation to pinprick and light touch in a dermatomal distribution, including the sacral segments. If any abnormalities are found, they are used to determine the sensory and motor levels of the SCI. The lowest intact motor level is the level at which the strength grade on manual muscle testing is at least a 3 (antigravity) and the level above has a strength of 5 (normal). Any sensation felt on the rectal examination indicates that the patient is sensory-incomplete. The ASIA grading scale (which is modified from the original Frankel scale) is as follows:
A = Complete. No sensory or motor function is preserved in the sacral segments S4–S5.
B = Incomplete. Sensory but not motor function is preserved below the neurologic level and includes the sacral segments S4–S5.
C = Incomplete. Motor function is preserved below the neurologic level, and more than half the key muscles below the neurologic level have a muscle grade less than 3.
D = Incomplete. Motor function is preserved below the neurologic level, and at least half the key muscles below the neurologic level have a muscle grade greater than or equal to 3.
E = Normal. Sensory function and motor function are normal.
This grading should be determined once the patient is out of spinal shock, which is defined by return of the bulbocavernosus reflex. The level and completeness of SCIs have prognostic value for both eventual neurologic recovery and functional independence.
Venous thromboembolism risk is greatly increased in individuals with SCIs, and consideration should be given to mechanical or medication prophylaxis. Particular consideration should be given to children who are showing signs of pubertal development or who are 14 years or older.
The long-term complications of SCI in children are myriad. They can prolong the rehabilitation process and persist long after discharge from inpatient rehabilitation. Children with lesions at the thoracic and cervical levels need aggressive pulmonary management ranging from assisted ventilation (invasive and noninvasive) to instruction in quad cough techniques. Insensate skin is vulnerable to pressure ulceration. Hypercalcemia may occur as an early complication as bones demineralize from immobility. The clinician should be aware of signs of hypercalcemia, which include the sudden onset of abdominal pain, nausea, vomiting, malaise, lethargy, polyuria, polydipsia, and dehydration. The patient may also exhibit behavioral changes or acute psychosis. Serum calcium levels above 10.8 mg/dL in a child and above 10.2 mg/dL in an adolescent are abnormal. Treatment should include fluids and furosemide to enhance the excretion of calcium.
In lesions above high thoracic (T5–T6) levels, the sympathetic nervous system is often affected. Early low sympathetic tone with orthostatic hypotension, which warrants management with abdominal binders and elastic stockings, may give way to later life-threatening autonomic dysreflexia. At the time of discharge, patients at risk should be given a card outlining the symptoms and treatment of autonomic dysreflexia to take with them to a clinic or emergency department that may be unfamiliar with the special health care problems of individuals with SCIs. It is imperative that the patient be treated immediately so that blood pressure is normalized and a potential stroke is prevented. The first line of treatment is to identify and remove any noxious stimuli such as an overdistended bladder or rectum, pain-inducing area, or skin issue while taking measures to decrease the blood pressure ( Table 20-2 ).
Autonomic Dysreflexia |
---|
A sudden increase in blood pressure, 20 to 40 mm Hg systolic higher than usual, resulting from painful stimuli applied below neurologic levels in persons with spinal cord injuries. The condition is caused by massive unopposed sympathetic discharge, occurs primarily in those with an injury above thoracic level T6. If left untreated it can lead to stroke, seizures, and even death. Autonomic dysreflexia is a medical emergency. |
Common Signs and Symptoms | |
---|---|
ABOVE LEVEL OF INJURY | BELOW LEVEL OF INJURY |
Hypertension Bradycardia or tachycardia Pounding headache Apprehension/anxiety/uneasy feeling Changes in vision Nasal congestion Sweating Flushed skin Goosebumps Tingling sensation | Nausea Chills without fever Clammy Cool Pale |
Common Causes |
---|
Distended bladder Constipated bowel Pressure ulcers Fractured bones Skin burns Urinary tract infections Ingrown toenails Any condition or procedure that may cause pain or discomfort but that is located below the neurologic injury level |
What to Do |
---|
Sit up. Take off or loosen any restrictive clothing. Check blood pressure every 5 minutes until at baseline. Check bladder; empty/catheterize. If indwelling catheter, check for kinks and blockages. Check bowel; disimpact, if needed, using anesthetic jelly. Check skin. Assess for any other sources of painful stimuli or irritant. If systolic blood pressure is more than 150 mm Hg, give antihypertensive agent with rapid onset and short duration while cause of autonomic dysreflexia is being investigated. Monitor symptoms and blood pressure at least 2 hours after the resolution of an autonomic dysreflexia episode. |
Neurogenic bladder must also be evaluated in a child with SCI. Some of the highest pressures in the bladder can be generated by bladder–sphincter dyssynergia after an SCI. Urodynamics are important because bladder patterns can be hard to predict; even if it appears that the patient is voiding normally, it could be due to high bladder pressures. Early bladder monitoring, clean intermittent catheterization, and the use of anticholinergics appear to prevent upper tract deterioration, improve continence, and decrease infections. It is important that the patient maintain a regular catheterization program to keep volumes at or below the maximal bladder volume for age. The volume can be roughly calculated with the following equation: (age + 2) years = bladder volume in ounces.
Neurogenic bowel involvement should not be overlooked. Bowel programs often include timing, stimulation, dietary recommendations, and medications, with the goal of age-appropriate continence. Children should be involved in their own bladder and bowel management as soon as appropriate for their age.
Fractures in children with SCIs can occur either immediately as a result of the same trauma that caused the SCI or later as chronic immobility leads to osteopenia. A recent review of the National Spinal Cord Injury Database revealed that 28% of individuals at the time of admission for acute SCIs also had extraspinal fractures. The most common locations of these fractures were, in decreasing order, the chest, lower extremity, upper extremity, head, other, and pelvis. Children with new SCIs should have a thorough evaluation for evidence of swelling, pain, and unusual mobility, with particular attention paid to insensate areas.
Individuals who sustain an SCI in childhood are at risk of long-term musculoskeletal complications. Long-term immobility may lead to osteoporosis in adulthood, particularly in individuals with higher level lesions. ∗
∗
In a recent study of adults who had sustained SCIs as children, young adults (median age, 31 years) showed decreased bone mineral density in the femoral region an average of 19 years after their injuries. Risk factors associated with osteoporosis after an SCI include immobilization for surgical treatment and complete injury. Osteoporosis may lead to low-velocity fractures, particularly of the supracondylar distal end of the femur (paraplegic fracture). Traditional approaches to fractures below the level of the SCI in paraplegic and tetraplegic individuals have included closed reduction with traction or a well-padded cast or splint that can be removed to inspect insensate skin. Evaluation of interventions aimed at reducing the loss of bone mineral density, including exercise, supported standing, and functional electric stimulation, has revealed mixed results. They may, however, have other benefits, such as improved strength, reduced hypercalcemia, and better cardiovascular fitness.Traumatic Brain Injury
Traumatic brain injury (TBI) is a major cause of acquired disability in children and has an annual incidence of 200 per 100,000. The Glasgow Coma Scale (GCS) is widely used in the classification of TBI severity. After resuscitation, a GCS score of 8 or less indicates severe brain injury, 9 to 12 represents moderate injury, and 13 to 15 indicates mild injury. A minimum score is no less than 3 ( Table 20-3 ). These criteria must be used with some caution, however, because nonneurologic factors may influence the GCS score. Other indicators of brain injury include loss of consciousness, length of posttraumatic amnesia, and changes on imaging studies of the brain. Some neurologic deficits may be detected on physical examination; other deficits are subtle and can be more fully evaluated with formal neuropsychologic testing. Prompt recognition is essential for proper monitoring and follow-up. Children who have had multiple traumatic injuries—even those without a clear head injury—should be assessed for the possibility of a concussion or mild TBI; return to play guidelines should be followed. For current information on concussions visit http://www.cdc.gov/concussion/HeadsUp/youth.html . These children may also need school accommodations. Children with moderate and severe TBIs continue to show deficits in multiple areas of functioning even 3 years after injury. Other delayed complications after brain injury include infection, seizures, pulmonary complications, hypercalcemia, deep venous thrombosis, endocrinologic changes, heterotopic ossification, and hydrocephalus.
Best Eye Response | Spontaneous: open with blinking at baseline | 4 |
Opens to verbal command, speech, or shout | 3 | |
Opens to pain, not applied to the face | 2 | |
None | 1 | |
Best Verbal Response | Oriented | 5 |
Confused conversation but able to answer questions | 4 | |
Inappropriate responses, words discernible | 3 | |
Incomprehensible speech | 2 | |
None | 1 | |
Best Motor Response | Obeys commands for movement | 6 |
Purposeful movement to painful stimuli | 5 | |
Withdraws from pain | 4 | |
Abnormal (spastic) flexion, decorticate posture | 3 | |
Extensor (rigid) response, decerebrate posture | 2 | |
None | 1 |
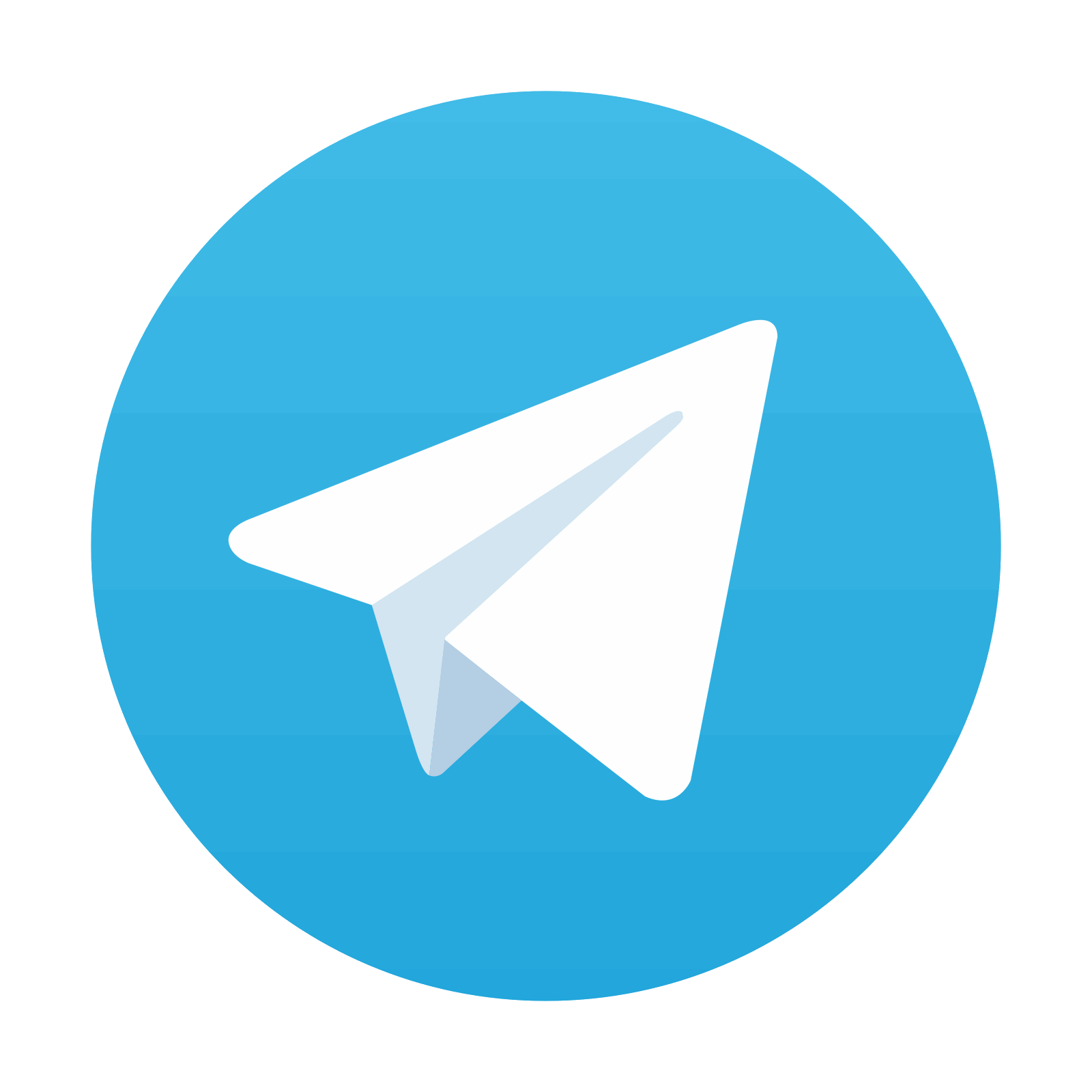
Stay updated, free articles. Join our Telegram channel

Full access? Get Clinical Tree
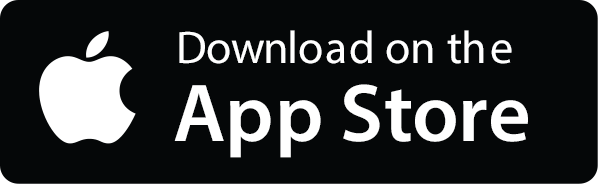
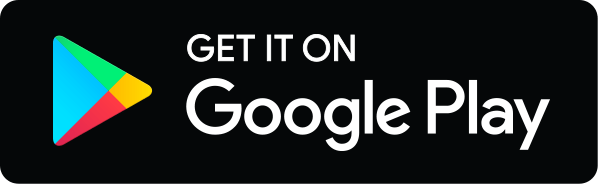
