Rehabilitation and regenerative medicine therapies has shown improved outcomes for tissue regeneration. Regenerative rehabilitation guides protocols regarding when to start therapy, types of stimuli administered, and graded exercise programs, taking into account biological factors and technologies designed to optimize healing potential. Although there are currently no evidence-based guidelines for rehabilitation, fundamental physical therapy principles likely apply. Immobilization tends to have deleterious effects on musculoskeletal tissues; mechanical loading promotes tissue healing and regeneration. Common physical therapy interventions may provide beneficial effects after the application of regenerative therapies. Research is needed to determine optimal rehabilitation protocols to enhance tissue healing and regeneration.
Key points
- •
Regenerative rehabilitation pairs exercise principles with regenerative therapies to facilitate the regeneration and repair of bone, muscle, cartilage, ligaments, tendons, nerves, and other musculoskeletal tissues.
- •
Mechanotherapies form one of the largest groups of interventions prescribed by physical therapists with nearly every intervention used introducing mechanical forces.
- •
A basic understanding of mechanotransduction and the impact of mechanical loading on cellular biology can guide the development of appropriate rehabilitation programs after regenerative therapies.
- •
Regenerative rehabilitation guides protocols for when to start therapy, types of stimuli administered, and graded exercise programs, taking into account biological factors and technologies designed to optimize healing potential.
Introduction
Regenerative medicine is an emerging, interdisciplinary field that combines advances in molecular biology, gene therapy, cellular therapy, and tissue engineering to replace or regenerate human cells, tissues, or organs. The goal of regenerative medicine is to restore or establish normal function after loss from any cause, including congenital defects, injury, disease, or aging. Given that physical rehabilitation shares the same goal, the combination of the 2 approaches may serve to enhance the desired treatment outcomes and could be transformative for individuals with previously untreatable injuries or disorders.
Regenerative rehabilitation is defined by the American Physical Therapy Association as “the integration of principles and approaches from rehabilitation and regenerative medicine, with the ultimate goal of developing innovative and effective methods that promote the restoration of function through tissue regeneration and repair.” Regenerative rehabilitation pairs exercise principles (eg, loading, intensity, frequency, duration), with regenerative therapies to facilitate regeneration and repair of bone, muscle, cartilage, ligaments, tendons, nerves, and other musculoskeletal tissues. This concept requires an interdisciplinary approach between scientists, clinicians, and physical therapists (PTs). In addition, it requires PTs to develop an understanding of the impact of exercise on cellular and molecular biology.
After tissue injury, PTs use targeted exercise therapy to enhance the efficiency of the body’s innate healing potential. Properly designed rehabilitation programs can play a critical role in optimizing the incorporation of regenerative therapies into the native tissues. The role of PTs should not be confined to restoring function after tissue regeneration or repair has occurred, because we should also play an active role in facilitating regeneration and repair during the healing process.
Introduction
Regenerative medicine is an emerging, interdisciplinary field that combines advances in molecular biology, gene therapy, cellular therapy, and tissue engineering to replace or regenerate human cells, tissues, or organs. The goal of regenerative medicine is to restore or establish normal function after loss from any cause, including congenital defects, injury, disease, or aging. Given that physical rehabilitation shares the same goal, the combination of the 2 approaches may serve to enhance the desired treatment outcomes and could be transformative for individuals with previously untreatable injuries or disorders.
Regenerative rehabilitation is defined by the American Physical Therapy Association as “the integration of principles and approaches from rehabilitation and regenerative medicine, with the ultimate goal of developing innovative and effective methods that promote the restoration of function through tissue regeneration and repair.” Regenerative rehabilitation pairs exercise principles (eg, loading, intensity, frequency, duration), with regenerative therapies to facilitate regeneration and repair of bone, muscle, cartilage, ligaments, tendons, nerves, and other musculoskeletal tissues. This concept requires an interdisciplinary approach between scientists, clinicians, and physical therapists (PTs). In addition, it requires PTs to develop an understanding of the impact of exercise on cellular and molecular biology.
After tissue injury, PTs use targeted exercise therapy to enhance the efficiency of the body’s innate healing potential. Properly designed rehabilitation programs can play a critical role in optimizing the incorporation of regenerative therapies into the native tissues. The role of PTs should not be confined to restoring function after tissue regeneration or repair has occurred, because we should also play an active role in facilitating regeneration and repair during the healing process.
Mechanotherapy
The term “mechanotherapy” was initially coined in the 19th century, and defined in the Oxford English Dictionary as “the employment of mechanical means for the cure of disease.” In 2009, Khan and Scott proposed to update the definition to “the employment of mechanotransduction for the stimulation of tissue repair and remodeling” to highlight the cellular basis of therapeutic exercise prescription for tissue healing. This definition also recognized that injured and healthy tissues may respond differently to mechanical loads.
In 2016, Thompson and colleagues proposed to once again update the definition to “any intervention that introduces mechanical forces with the goal of altering molecular pathways and inducing a cellular response that enhances tissue growth, modeling, remodeling, or repair.” This definition highlights the responsiveness to mechanical signals at a multisystem level (ie, molecular level, cellular level, and tissue level) and recognizes the influence of mechanical forces on the processes responsible for tissue development, maintenance, healing, and regeneration.
Mechanotherapies form one of the largest groups of interventions prescribed by PTs. Nearly every intervention used in the practice of physical therapy introduces mechanical forces. Such interventions, including but not limited to, exercise prescription, joint mobilization, soft tissue mobilization, muscle stretching, and even neuromuscular electrical stimulation, provide mechanical stimulation at both the cellular and tissue levels. Developing insight into the molecular and cellular responses to the forces used in daily practice will allow PTs to increase understanding of therapeutic dosing and potentially improve clinical outcomes in patients undergoing regenerative therapies.
Mechanotransduction
Mechanotransduction refers to the physiologic process by which cells convert mechanical stimuli into cellular responses. These cellular responses will, in turn, promote structural adaptation. Mechanotransduction consists of 3 distinct phases: (1) mechanocoupling, (2) cell–cell communication, and (3) the effector response. Mechanocoupling refers to a mechanical stimulus or load that causes physical perturbation to cells. The perturbation may be direct or indirect and can trigger a variety of cellular responses depending on the type, magnitude, frequency, and duration of the load. Cells may be exposed to an array of mechanical forces, including tension, compression, shear, hydrostatic pressure, vibration, and fluid shear. The tissue in which a cell resides, as well as the location of the cell within that tissue, will influence the types of mechanical stimuli to which the cell is exposed. The mechanical stimuli are sensed by various mechanosensitive molecular mechanisms that transduce the signal intracellularly. These mechanisms may include cell surface receptors, integrins, focal adhesion complexes, stretch-activated ion channels, growth factor receptors, and the extracellular matrix, to name a few.
The cell–cell communication phase, sometimes referred to as the signal propagation phase, involves the recruitment of cell signaling pathways and other means of signal spread (eg, cytoskeleton tension) for the biochemical conversion and propagation of the transmitted mechanical signal. Just as there are multiple mechanosensitive mechanisms available to sense the mechanical stimulus, there are also multiple signaling pathways that a cell may use to create a biochemical response. The cell signaling pathways are composed of a cascade of multiple potential cytosolic mediators that will transmit the biochemical signal from the cell surface to the effector endpoint.
The final phase of mechanotransduction is the effector response. Once the biochemical signal is transmitted to the effector endpoint, changes in cellular biology occur. If the endpoint is the nucleus, the signal may induce expression of mechanosensitive genes. If the endpoint is effector cells, changes may include an increase or decrease in intracellular tension, changes in adhesive properties, cytoskeletal reorganization, and/or modulation of cellular proliferation, differentiation, migration, and apoptosis.
Eccentric exercise provides an excellent example of the potential impact of mechanotransduction on muscle repair or regeneration. Muscle regeneration depends on a functioning population of satellite cells. Eccentric exercise has been shown to stimulate an array of cellular responses that have the potential to optimize the regenerative process in muscle by stimulating the activation and proliferation of satellite cells. Although the underlying mechanisms are still under investigation, recent studies demonstrate that interstitial nonmyogenic, nonsatellite stem cells, including pericytes, may play an important role in the regenerative process.
Although nonmyogenic, pericytes have been shown to secrete a variety of beneficial growth factors and antiinflammatory cytokines (eg, insulinlike growth factor-1, interleukin-6, vascular endothelial growth factor, and hepatocyte growth factor) that activate satellite cells upon extraction from exercised muscle. Valero and colleagues demonstrated an increase in the accumulation of pericytes and an increase in satellite cell number in muscle after acute eccentric exercise in mice. Results of the study suggest that the pericytes upregulate the expression of stem cell markers when subjected to mechanical strain, potentially accounting for the significant increase in the accumulation of satellite cells in muscle after exercise. In addition, the authors demonstrated that pericytes indirectly stimulate new fiber synthesis after injection of muscle-resident mesenchymal stem cells, particularly when recipient mice are exercised immediately before injection. The results of this study provide evidence that coordinated communication between pericytes and satellite cells positively influence muscle regeneration after eccentric exercise.
Clinical integration of mechanotransduction
Recent advances in the field of regenerative medicine, including the use of platelet-rich plasma (PRP), stem cell therapy, and tissue engineering provide promising strategies to enhance tissue repair after musculoskeletal injury. The success of these regenerative medicine technologies ultimately depends on the therapies being incorporated into the native tissue and creating a musculoskeletal tissue with enhanced mechanical characteristics. Physical therapy has foundations in the use of targeted mechanical stimuli designed to enhance the intrinsic healing potential of tissue. A basic understanding of mechanotransduction and the impact of mechanical loading on cellular biology can help to guide the development of appropriate rehabilitation programs after the application of regenerative therapies.
Eccentric exercise, as discussed, is 1 form of mechanotherapy that may serve to facilitate tissue healing in conjunction with regenerative therapies. A more recent strategy involving low-load resistance training with blood flow restriction (BFR), may potentially play a role in regenerative rehabilitation. This form of exercise, also referred to as strength training with vascular occlusion, has been shown to increase muscle hypertrophy and strength similar to heavy-load resistance training. BFR training involves decreasing blood flow to a muscle using a wrapping device, such as a blood pressure cuff or specially designed restrictive straps. Muscle hypertrophy has been demonstrated during resistance exercises with intensities as low as 20% of 1 repetition maximum with moderate vascular restriction (∼100 mm Hg). In some studies, BFR training has been applied with high frequency (1–2 sessions per day) and short duration (1–3 weeks), resulting in significant increases in muscle size and strength. This form of training could potentially be quite beneficial to patients after regenerative therapies that are unable to tolerate high-intensity resistance training (≥70% 1 repetition maximum).
Although the underlying cellular mechanisms responsible for the adaptive changes in muscle size and strength are largely unknown, recent studies show increased protein synthesis after acute bouts of BFR training, accompanied by posttranslation regulation in the AKT/mammalian target of rapamycin pathway. Nielsen and colleagues demonstrated marked proliferation of satellite stem cells and increased number of myonuclei per myofiber with a short-term, high-frequency BFR training program using low-load resistance.
Platelet-Rich Plasma
The use of PRP injections is one of the most common applications of regenerative therapies for musculoskeletal injury. Although originally introduced in the 1970s, the use of PRP injections has increased significantly in recent years, especially for the treatment of sports-related ligament and tendon injuries. Rehabilitation protocols after PRP therapy have not been well-outlined in the literature. Although physical therapy is often used after PRP injections, there is little clinical evidence regarding the optimal program design. Virchenko and Aspenberg examined the relationship between mechanical stimulation and tendon healing after PRP injection in the transected Achilles tendons of rats. Results of this study indicated that although PRP improved the material properties of the tendon callus, the effects were lost when the tendon was unloaded mechanically. The authors concluded that mechanical stimulation may be necessary for PRP therapy to be successful. In addition, the study demonstrated that only the early phases of tendon regeneration were influenced by the platelets, stressing the importance of mechanical stimulation early in the process of healing.
The results of the Virchenko and Aspenberg study have led to most rehabilitation protocols endorsing early controlled loading of the tissue after PRP therapy for tendinopathy. Although several studies investigating the efficacy of PRP injection to promote tissue healing have demonstrated improvements in various outcome measures, few have described the rehabilitation program or activity level of the involved subjects after PRP therapy. Those that provide a detailed description of post-PRP rehabilitation are often case reports or case series versus randomized controlled trials. Although a common recommendation after PRP injection for the treatment of tendon pathology is the use of eccentric exercise, the timing of exercise implementation varies widely.
Kaux and colleagues described a standardized rehabilitation program based on submaximal eccentric exercise after PRP injection for patients with patellar tendinopathy. The protocol used 1 week of relative rest before initiating a progressive regimen of closed kinetic chain eccentric quadriceps strengthening performed 3 times per week. Progression of eccentric exercise was based on pain threshold as recommended by Stanish. Results of this case series indicated significant improvements for pain and function, as measured by the visual analog scale, the International Knee Document Committee scale, and the Victorian Institute of Sport Assessment scale.
van Ark and colleagues described a 5-phase rehabilitation program after PRP injection for patients with patellar tendinopathy. The goal of the initial 3 phases was to create conditions to optimize recovery. The final 2 phases focused on loading to optimize return to sport/activity. Gradual progression of the intensity of the exercise program was based on correct execution of exercises and a visual analog scale score of 50 or less on a scale of 0 to 100. Eccentric exercises were implemented in the third phase of the program and were initially performed only twice a week to allow the tendon to adapt to the load. The protocol described by van Ark and colleagues incorporates both concentric and eccentric training, because the combination of both types of training may promote collagen synthesis. In addition, the protocol integrated core stability exercises, because core muscle strength has been found to be important in the load distribution on the patellar tendon. Results of this case-series demonstrated improvements in both pain and function as measured by the visual analog scale and Victorian Institute of Sport Assessment. The results also indicated a potential relationship between patient compliance and improvement in pain and function; the 3 patients with the highest self-reported program compliance demonstrated the greatest improvement in Victorian Institute of Sport Assessment scores, and the 1 patient who did not improve had the lowest self-reported compliance.
Based on the study by Virchenko and Aspenberg, it seems that tendon healing requires a combination of biological and mechanical factors. Rehabilitation after PRP therapy is necessary to provide the mechanical loading component. There are a number of important factors to consider when designing a rehabilitation program after PRP injection. In addition to addressing specific impairments the patient may have, interventions should facilitate the potential synergistic effects of mechanical loading and PRP therapy. Factors to be considered include timing of the initiation of physical therapy after PRP injection, type of exercise (eg, concentric vs eccentric loading), intensity, duration, and frequency. Although treatment should be individualized for each patient after PRP therapy, general guidelines are provided in Table 1 for rehabilitation after PRP or stem cell therapy for tendon, ligament, and/or articular cartilage. In addition, the rehabilitation protocol after PRP injection for patellar tendinopathy as described by van Ark and colleagues is detailed in Box 1 .
Phase I—(0–3 d) | |
Goals |
|
Precautions |
|
Suggested interventions |
|
Criteria for progression | Minimal pain/discomfort (<50 on VAS) with AROM |
Phase II—(3–14 d) | |
Goals |
|
Precautions |
|
Suggested Interventions |
|
Criteria for progression |
|
Phase III—(2–8 wk) | |
Goals |
|
Precautions |
|
Suggested interventions |
|
Criteria for progression |
|
Phase IV—(≥8 wk) | |
Goals |
|
Precautions |
|
Suggested interventions |
|
Criteria for return to sport/work |
|
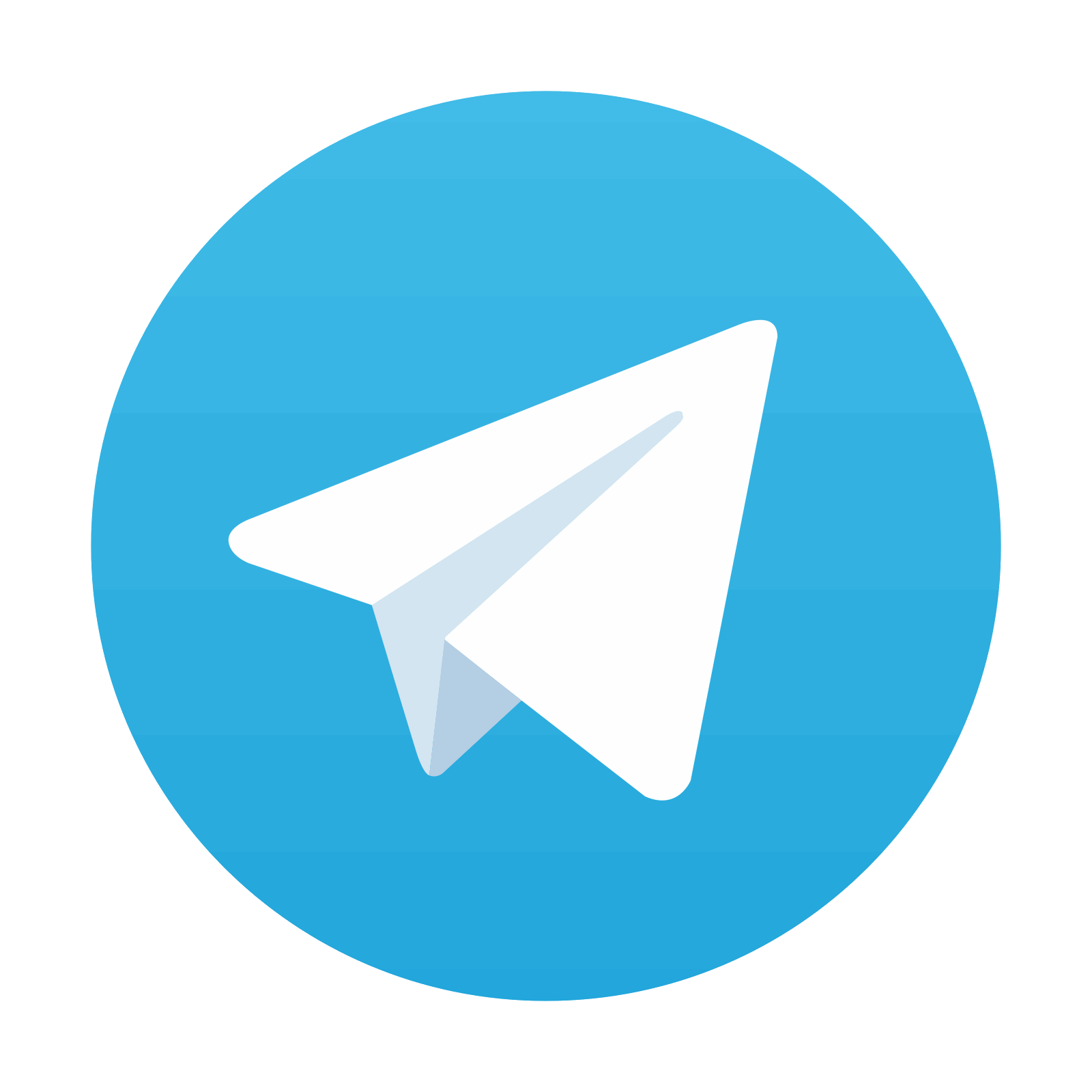
Stay updated, free articles. Join our Telegram channel

Full access? Get Clinical Tree
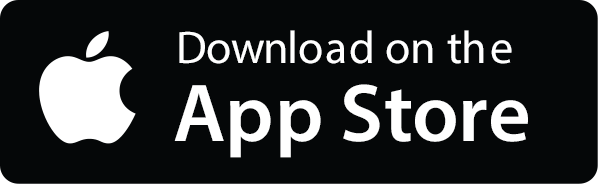
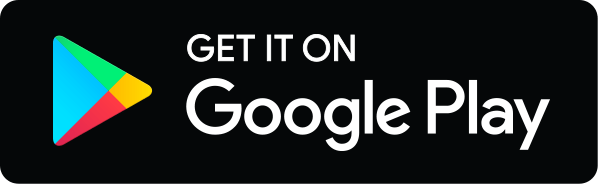
