Rehabilitation After Spine Surgery—Relevant Anatomy
David Alex Stroh, MD
Bradley Moatz, MD
P. Justin Tortolani, MD
Dr. Moatz or an immediate family member has received nonincome support (such as equipment or services), commercially derived honoraria, or other non-research–related funding (such as paid travel) from Globus Medical and Vertebral Technologies Incorporated. Dr. Tortolani or an immediate family member has received royalties from Globus Medical; serves as a paid consultant to Globus Medical, Innovasis, and Spineology; has received research or institutional support from Spineology; and serves as a board member, owner, officer, or committee member of the Journal of Spinal Deformity, Medstar Union Memorial Hospital, and Surgical Neurology International. Neither Dr. Stroh nor any immediate family member has received anything of value from or has stock or stock options held in a commercial company or institution related directly or indirectly to the subject of this article.
Introduction
A thorough understanding of the anatomy of the spine, spinal cord, and surrounding structures forms the basis for sound surgical treatment, as well as successful postoperative rehabilitation. A stable axial skeleton is the cornerstone of ambulation and the support for the major organ spaces (head, thorax, abdomen, and pelvis). The particular structure and biomechanics of the vertebral column and musculature dictate what techniques and exercises will help patients achieve balance, correct posture, functionality, or accommodate postoperative difficulties in mobilization. While an extensive review is beyond the scope of this chapter, the reader is referred to classic works for further learning; the basic principles presented here will provide a framework for spine kinesiology as well as a rationale for what constitutes safe postoperative physical therapy.
Anatomy of the Adult Spine
Topical Anatomy
Pertinent topical anatomy starts with the occiput at the posterior base of the skull, from which a ridge of bone (the superior nuchal line) extends laterally. As one works inferiorly, the spinous processes increase in size, with the most easily palpable at C7 (the vertebral prominens). The thoracic spinous processes gradually angulate inferiorly from cranial to caudal, so that the vertebral body may be located at times superior to the palpable spinous process. Examination of the topical landmarks provides useful information. First, there should be no step-off between spinous processes, which otherwise may indicate spondylolisthesis. The posterior superior iliac spine (PSIS) may be palpated with the thumbs, and the fingers curled anteriorly to define the “version” (inclination in the sagittal plane) of the pelvis. On the Adams forward bending test, the spinous processes and ribs serve as landmarks in the evaluation of scoliosis. Various topical landmarks are useful in estimating the level of spinal vertebrae (Table 61.1).
Vertebral Elements
The spine is organized into cervical (C1–C7), thoracic (T1–T12), lumbar (L1–L5), fused sacral (S1–S5), and fused coccygeal (4 total) segments, as shown in Figure 61.1, with additional lumbar and cervical vertebrae or sacralized terminal lumbar vertebrae being common variations. Four transition zones are notable. The region from the occiput to the first and second cervical vertebrae is highly specialized, with C1 forming a flattened ring to both cradle the skull and rotate about the dens (or axis) of C2. Two junctions within the vertebral column itself accompany the transition from the lordotic cervical and lumbar regions to the kyphotic thoracic region. Finally, the lumbosacral junction provides a connection to the bony pelvis via the relatively immobile sacroiliac (SI) joints. These junctions are unique in that they are areas of transition from a relatively immobile spinal segment (thoracic and sacral) to a more mobile one (cervical and lumbar). As a result, these areas are under relatively higher stress and are particularly vulnerable to injury.
Table 61.1 TOPICAL LANDMARKS AND SPINAL LEVELS | ||||||||||||||||||||||
---|---|---|---|---|---|---|---|---|---|---|---|---|---|---|---|---|---|---|---|---|---|---|
|
All typical vertebrae share certain features (Figure 61.2): ventrally a cylindrical body, dorsally a vertebral arch, and paired pedicles connecting the two and completing the ring of the vertebral foramen. The body is composed of mostly cancellous bone with a thin cortical rim. On each side, three processes emanate from the region where the pedicle meets the remainder of the vertebral arch (the posterior elements). The transverse process lies between the superior and inferior processes, and serves as a bony lever arm for the attachment of ligaments and muscles. The bony isthmus between the superior and inferior processes is the pars interarticularis, an area prone to stress fracture with repetitive translational motion of adjacent vertebrae. Two flattened laminae spread dorsally from the isthmus, completing the vertebral arch and coalescing into an elongated posterior spinous process.
Regional variations in vertebral morphology balance the need for both stability and range of motion (ROM). From cephalad to caudad, body size increases to support the increased weight borne by the lumbar spine. The orientation of facet joints slowly shifts from roughly 45° from the sagittal plane (semicoronal) to nearly parallel. This restricts the amount of permissible rotation in the lumbar spine. Pedicle diameter increases almost linearly from C3 to T1, decreases linearly from T1 to T4–T6, then increases almost exponentially from T6 to S1 (with the notable exception that the L1 pedicles are often smaller than T12). In the axial plane, their orientation also becomes more medially angulated in the inferior segments. These variations make pedicle screw placement more difficult in the cranial regions of the thoracic and cervical spine, to the point at which screws placed in the lateral mass are commonly used for posterior cervical fixation. Finally, the anterior lip of a cervical vertebra slightly overlaps the disc inferiorly, and from C3 to C7 there are superiorly directed uncinate processes on the lateral peripheries of the superior endplates that articulate with a groove (the uncus) on the inferior endplate of the body above.
Anterior to the transverse process exists some form of costal element. In the cervical spine, this fuses anteriorly to the body to form the transverse foramen for the vertebral artery. The anterior portions of the C7 transverse processes inconsistently form anomalous cervical ribs, a risk factor in the development of thoracic outlet syndrome. The costal element of the thoracic spine is a facet and its corresponding rib, which in some cases spans two vertebrae and serves to stiffen the thoracic segments. The costal element in the lumbar vertebrae is split to form the mammillary, accessory, and part of the transverse processes. In the sacrum, the costal elements fuse to the transverse processes above and below to present the contiguous articulating surface of the SI joint.
The vertebrae of the upper cervical and sacral regions are highly specialized. The atlas (C1) and axis (C2) lack formal vertebral bodies; instead, they comprise anterior and posterior arches. C1 has concave facets both superiorly and inferiorly on C1 to accept the curved occiput and the lateral mass (a fusion of the pedicle and the articular facets) of C2. The dens of C2 is held anteriorly against C1 primarily by the transverse, alar, and apical ligaments, as well as the tectorial membrane (a continuation of the posterior longitudinal ligament). The fused sacrum presents a continuous lateral articulation for the SI joint, a middle sacral crest representing the fused spinous processes, lateral crests which create a groove for the multifidus, and sacral foramina to transmit the sacral nerve roots.
Joints and Ligaments of the Vertebral Column
A variety of joints are found throughout the vertebral column. All typical vertebrae contribute superior and inferior articular processes to the facet or zygapophyseal joints, so named because they “yoke” together two adjacent vertebrae. Facet joints are relatively mobile and permit gliding motion to accommodate flexion–extension and some degree of rotation. From C3 to C7, the inferior vertebral body contacts the superior body at the lateral periphery via the uncovertebral joints, which permits flexion–extension but limits lateral flexion. In the thoracic spine, costal facets articulate with same-leveled ribs both on the vertebral bodies and on certain transverse processes to permit rib motion.
The intervertebral disc is an arthrodial joint that separates adjacent vertebrae and cushions axial load. It comprises an outer annulus (primarily type 1 collagen) and an inner nucleus pulposus (relatively high proteoglycan content). The hydrophilic proteoglycans draw in water and hydrate the nucleus, which allows it to resist compression. Injuries to the disc may include incomplete annular tears, full-thickness tears, and herniations of the nucleus pulposus. These are categorized into disc bulges (intact, but weakened, annulus expands broadly under pressure), protrusions (disc material breaks through the annulus), or extrusions (nuclear material breaks off into the vertebral foramen). The disc does not receive any blood vessels, and most of the nutrients necessary to the cells within the disc are delivered via diffusion from vessels in the endplate.
Ligamentous restraints in the spinal column (Figure 61.3) include the anterior longitudinal ligaments (ALL) and posterior longitudinal ligaments (PLL), facet joint capsule, ligamentum flavum, interspinous ligament, and supraspinous ligament (and its cervical continuation, the ligamentum nuchae). These ligaments restrict safe vertebral motion and stabilize the spine. Ligaments contacting the intervertebral or spinal foramina
(PLL, facet capsule, ligamentum flavum) may cause nerve compression if they become hypertrophic.
(PLL, facet capsule, ligamentum flavum) may cause nerve compression if they become hypertrophic.
Nervous System Anatomy and Pathoanatomy
The spinal cord is divided into longitudinal tracts (Figure 61.4) well organized by function (ascending sensory vs. descending motor/inhibitory), region (cervical, thoracic, lumbar, and sacral), and cell type (gray matter vs. white matter). The white matter tracts of the spinal cord are located peripherally, compared to the central gray matter of motor neuron bodies. Paired dorsal (ascending axons of sensory neurons in the dorsal root ganglion) and ventral roots (descending axons of motor neurons) emanate from the spinal cord, coalescing into the proper spinal nerve before exiting the vertebral foramen and splitting into dorsal and ventral rami. Various motor, sensory, and reflex physical examination findings are attributed to specific nerve roots, as shown in Table 61.2.
In considering disc herniations, two facts help the investigator correlate imaging findings with expected radicular symptoms to determine the spinal nerve affected. First, from C3 to C7, the nerve roots are located above the pedicle bearing their name (e.g., C7 exits above the C7 pedicle), a pattern that changes at T1 since no C8 vertebra exists for the C8 nerve root (T1 exits below the T1 pedicle). Second, the exit angle of the nerve roots is shallow in the cervical spine (nearly horizontal) and becomes steeper caudally. Because of this, cervical disc herniations will almost always affect one nerve root (usually the higher-numbered nerve), while lumbar herniations may affect either the exiting/lower-numbered nerve root (if sufficiently lateral to the foramen) or the traversing/higher-numbered nerve root (more central). The spinal cord ends in most at the L1 to L2 level, terminating in a swelling known as the conus medullaris. Stenosis of the vertebral foramen from any cause in levels above this carries a risk of spinal cord compression, which can lead to myelopathy. Below L2, only nerve roots can be compressed, leading to radiculopathy. In cases of complete occlusion of the vertebral foramen beneath L2, cauda equina syndrome may develop.
Vascular Anatomy
The blood supply to the spinal cord is derived from two anterior and one posterior spinal arteries. These receive other vessels throughout the length of the cord (segmental medullary and intercostal arteries) from the subclavian/vertebral arteries, or directly from the aorta. The largest of these segmental medullary arteries, the artery of Adamkiewicz, enters in the midthoracic spine and can be interrupted by endovascular grafts, retroperitoneal dissection, or thrombosis leading to spinal cord ischemia. Traveling within the vertebral foramina are anterior and posterior radicular branches from the dorsal branches of the intercostal arteries, which may be a source of bleeding with aggressive dissection in this area. Venous drainage is achieved through a convoluted internal vertebral plexus that spans the length of the spinal cord. These vessels also receive venous drainage from the pelvis and thorax via the Batson venous plexus, a valveless system that has been implicated in the spread of metastatic tumors and infections to the vertebral column.
The course of the vertebral artery is especially important in dissection and instrumentation of the cervical spine. The vessel travels through the transverse foramen at all cervical levels except C7. It then makes a sharp curve after exiting the foramen of C1 and travels medially, before turning superiorly to enter the foramen magnum. Surgical dissection any more than 2 cm lateral to the vertebral body risks damage to the vertebral artery.
Spinal Musculature
The muscles of the neck are arranged into anterior groups (superficial, suprahyoid, infrahyoid, and deep) and posterior
groups (superficial, suboccipital triangle), as seen in Table 61.3. These muscle groups are separated by the superficial, pretracheal, and prevertebral fascias. The typical anterior approach to the spine utilizes a transverse, left-sided incision (to avoid the more vulnerable recurrent laryngeal nerve on the right) that splits the platysma and longus colli, leaving all other muscles intact. The midline posterior cervical approach, like all midline spine incisions, passes through the fasciotendinous junction of the paraspinal muscles, then continues beneath the periosteum once bone is reached. As in the posterior lumbar approach, dissection laterally from the spinous process potentially disrupts the dorsal rami, innervating the paraspinal musculature. Since these muscle tracts are segmentally innervated, no significant paresis or deficit occurs.
groups (superficial, suboccipital triangle), as seen in Table 61.3. These muscle groups are separated by the superficial, pretracheal, and prevertebral fascias. The typical anterior approach to the spine utilizes a transverse, left-sided incision (to avoid the more vulnerable recurrent laryngeal nerve on the right) that splits the platysma and longus colli, leaving all other muscles intact. The midline posterior cervical approach, like all midline spine incisions, passes through the fasciotendinous junction of the paraspinal muscles, then continues beneath the periosteum once bone is reached. As in the posterior lumbar approach, dissection laterally from the spinous process potentially disrupts the dorsal rami, innervating the paraspinal musculature. Since these muscle tracts are segmentally innervated, no significant paresis or deficit occurs.
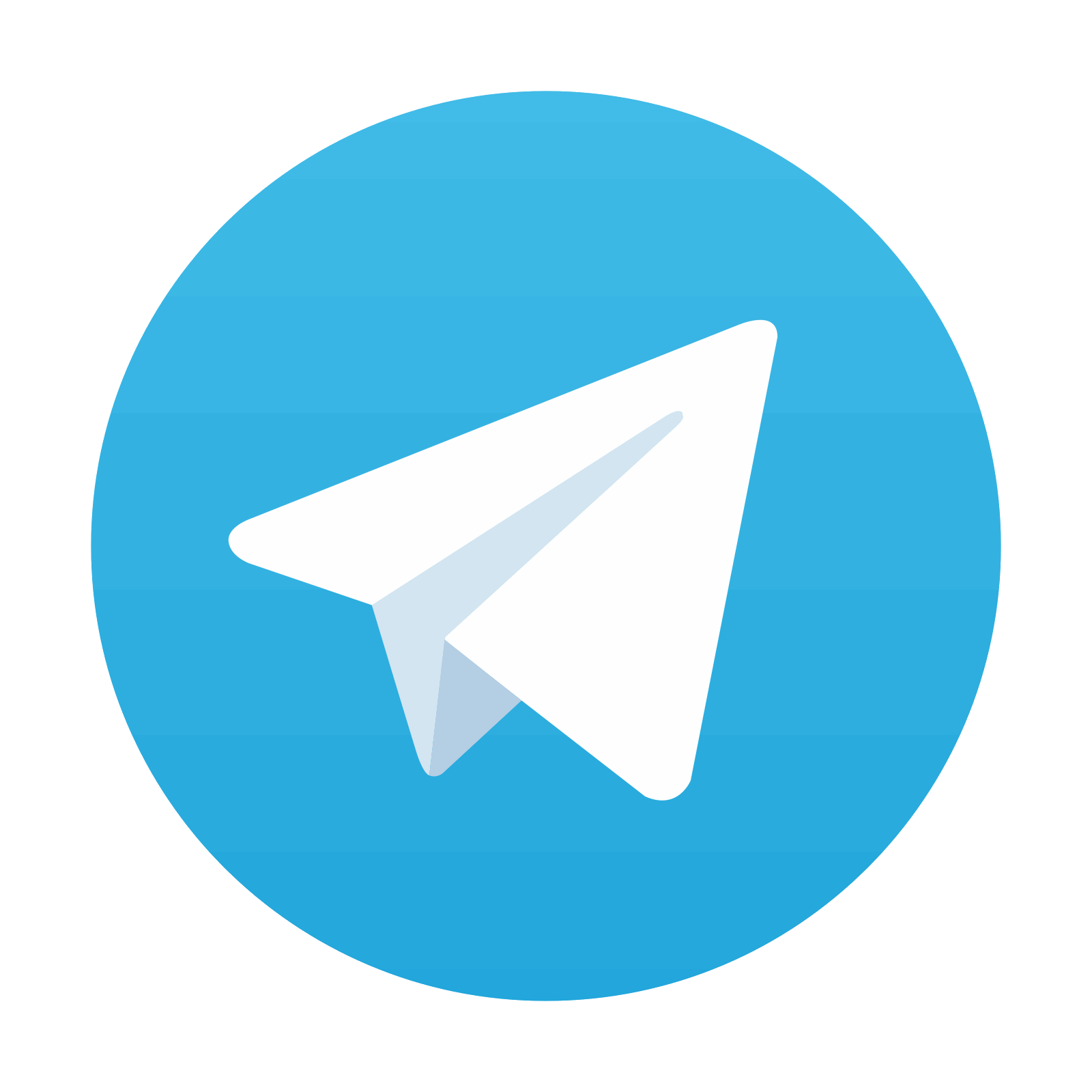
Stay updated, free articles. Join our Telegram channel

Full access? Get Clinical Tree
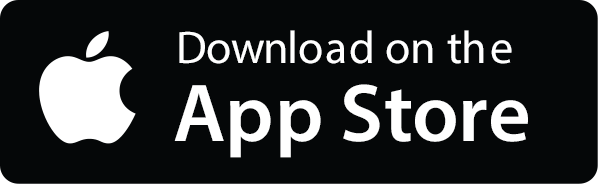
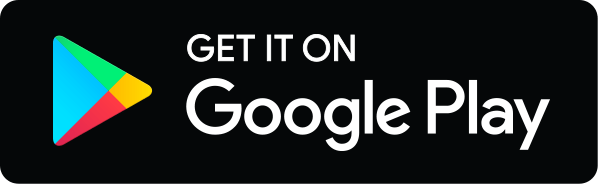