Tendon and ligament injuries and degenerative conditions of these soft tissues have poor healing potential and healing is often incomplete. Biocellular and orthobiologic approaches including PRP and stem cell therapies are reviewed. A review of some of the regenerative medicine science and difficulties facing physicians exploring these methods is presented. A series of cases are reviewed demonstrating the application of these principles. Clinical experience with many of these biocellular interventions is outpacing validation in basic science studies. Clinical experience dictates the need for repeated clinical and imaging evaluation and the need for repeated intervention or change in strategies when needed.
Key points
- •
Healing of soft tissue injuries and lesions are often incomplete leaving the patient with residual pain, joint dysfunction, and functional disabilities.
- •
There are multiple types of platelet preparations which need to be properly selected depending on phases of healing.
- •
Stem cells derived from multiple sources may help modulate tissue repair.
- •
Extracellular matrix proteins and scaffolding may help facilitate tissue remodeling by providing a mechanical and biological environment for cells to migrate into, align and proliferate.
- •
Growth factors modulate tissue repair.
Introduction
Soft tissue pathology caused by acute injury, cumulative stress, or the sequelae of biomechanical faults and degenerative changes represent a significant portion of the practice of physical medicine and rehabilitation and sports medicine physicians. For many years, nonoperative management choices included rest, physical therapy, splinting and orthosis, medications, and physical modalities that often left patients with few other options for management of soft tissue injury. Healing of soft tissue injuries and lesions is often incomplete, leaving the patient with residual pain, joint dysfunction, and functional disabilities. Patients and physicians who manage these conditions are left searching for treatment options and alternatives. Intratendinous injections of corticosteroid may reduce stiffness and pain but also reduce the physical forces required to injury the tendon in the future. Injury to ligaments often results in joint effusion, altered movement, muscle weakness, reduced functional performance, and may result in significant loss of time out of play.
Over the past 2 decades, technological advancements are emerging that use a “tissue engineering” approach to these conditions. Langer and Vacanti first described 4 basic components required in tissue engineering: a structural scaffold, a cell source, biological modulators, and mechanical modulators. As various biologic and biocellular techniques have emerged, physicians have been trying to make the translational application to clinical practice where various treatment protocols are being developed. Unfortunately, the empirical experience with these techniques far exceeds the validation of these techniques in controlled studies.
The anterior cruciate ligament (ACL) is of particular importance, with some unique features that warrant discussion. Worldwide, young athletes experiencing anterior cruciate ligament injuries range from 17% to 61%. In the United States, 350, 000 ACL reconstructive surgeries are performed annually. The ACL is thought to have a poor healing capacity with a substantially higher rate of failure, which has led to a unanimous abandonment of suture repair and adoption of ACL reconstruction as the goal standard of care for ACL injuries, especially in younger athletes, whose primary goal is to return back to high-level sports activity. Because the ACL is an intra-articular, extrasynovial ligament, ACL injury and the disruption of the synovial sheath do not allow a local hematoma formation to take place, which is crucial for the onset of the inflammatory response that would stimulate primary healing. Despite ACL reconstruction, those athletes who successfully return back to play are at high risk for a second injury and will have a less favorable outcome. Importantly, ACL injuries are associated with long-term clinical sequelae despite ACL reconstruction, such as meniscus tears, chondral lesions, and the onset of posttraumatic osteoarthritis. Recent technological advancements in tissue engineering and regenerative medicine have demonstrated promising use of novel biologic/tissue engineering techniques that include growth factors, stem cells, and the use of bio-scaffolding to improve ACL healing and repair.
Primary disorders of tendons (tendinopathy), due to overuse or age-related degeneration, are common problems encountered in the day-to-day practice. Although there are no accurate figures specifically related to tendon disorders, studies from primary care show 16% of the general population suffer from rotator cuff shoulder pain and 21% in the elderly. The incidence of rotator cuff pain is even more common in the sports community. It has been stated that injuries to tendons are involved in 30% to 50% of all sports injuries. Rotator cuff disease is the most common upper extremity disability. Due to hypocellularity and hypovascularity in tendinopathy, the natural ability of tendons to heal is extremely low.
As biocellular techniques and tissue engineering protocols continue to emerge, we will need a more comprehensive classification system based on ultrasonography and clinical presentation to identify which type of tissue engineering strategy would be most appropriate for the category of tendinopathy. Developing both diagnostic and interventional skills using high-resolution ultrasonography will become increasingly important as regenerative medicine and tissue engineering techniques and protocols continue to emerge. Sensitivity figures of 0.98 are reported when using a 10-MHz or greater frequency ultrasound probe when detecting full-thickness rotator cuff tears. It is becoming more commonplace for non-radiologists to become experienced with ultrasonography who can develop similar high levels of accuracy when diagnosing partial-thickness tears as compared with musculoskeletal radiologists, which is statistically better, than general radiologists and sonographers. Unfortunately, ultrasonography still remains user dependent and will continue to place demands on practicing physicians to improve diagnostic ultrasonography skills. Once the proper skill level is achieved, it will help ensure appropriate assessment and categorization of ligament and tendon injuries in order select the appropriate intervention to improve outcomes. In addition, regenerative medicine and tissue engineering techniques currently being used remain, for the most part, empirical and will require validation through clinical trials. The purpose of this article was to discuss basic concepts and strategies using a tissue engineering approach to soft tissue injuries of tendons and ligaments.
Introduction
Soft tissue pathology caused by acute injury, cumulative stress, or the sequelae of biomechanical faults and degenerative changes represent a significant portion of the practice of physical medicine and rehabilitation and sports medicine physicians. For many years, nonoperative management choices included rest, physical therapy, splinting and orthosis, medications, and physical modalities that often left patients with few other options for management of soft tissue injury. Healing of soft tissue injuries and lesions is often incomplete, leaving the patient with residual pain, joint dysfunction, and functional disabilities. Patients and physicians who manage these conditions are left searching for treatment options and alternatives. Intratendinous injections of corticosteroid may reduce stiffness and pain but also reduce the physical forces required to injury the tendon in the future. Injury to ligaments often results in joint effusion, altered movement, muscle weakness, reduced functional performance, and may result in significant loss of time out of play.
Over the past 2 decades, technological advancements are emerging that use a “tissue engineering” approach to these conditions. Langer and Vacanti first described 4 basic components required in tissue engineering: a structural scaffold, a cell source, biological modulators, and mechanical modulators. As various biologic and biocellular techniques have emerged, physicians have been trying to make the translational application to clinical practice where various treatment protocols are being developed. Unfortunately, the empirical experience with these techniques far exceeds the validation of these techniques in controlled studies.
The anterior cruciate ligament (ACL) is of particular importance, with some unique features that warrant discussion. Worldwide, young athletes experiencing anterior cruciate ligament injuries range from 17% to 61%. In the United States, 350, 000 ACL reconstructive surgeries are performed annually. The ACL is thought to have a poor healing capacity with a substantially higher rate of failure, which has led to a unanimous abandonment of suture repair and adoption of ACL reconstruction as the goal standard of care for ACL injuries, especially in younger athletes, whose primary goal is to return back to high-level sports activity. Because the ACL is an intra-articular, extrasynovial ligament, ACL injury and the disruption of the synovial sheath do not allow a local hematoma formation to take place, which is crucial for the onset of the inflammatory response that would stimulate primary healing. Despite ACL reconstruction, those athletes who successfully return back to play are at high risk for a second injury and will have a less favorable outcome. Importantly, ACL injuries are associated with long-term clinical sequelae despite ACL reconstruction, such as meniscus tears, chondral lesions, and the onset of posttraumatic osteoarthritis. Recent technological advancements in tissue engineering and regenerative medicine have demonstrated promising use of novel biologic/tissue engineering techniques that include growth factors, stem cells, and the use of bio-scaffolding to improve ACL healing and repair.
Primary disorders of tendons (tendinopathy), due to overuse or age-related degeneration, are common problems encountered in the day-to-day practice. Although there are no accurate figures specifically related to tendon disorders, studies from primary care show 16% of the general population suffer from rotator cuff shoulder pain and 21% in the elderly. The incidence of rotator cuff pain is even more common in the sports community. It has been stated that injuries to tendons are involved in 30% to 50% of all sports injuries. Rotator cuff disease is the most common upper extremity disability. Due to hypocellularity and hypovascularity in tendinopathy, the natural ability of tendons to heal is extremely low.
As biocellular techniques and tissue engineering protocols continue to emerge, we will need a more comprehensive classification system based on ultrasonography and clinical presentation to identify which type of tissue engineering strategy would be most appropriate for the category of tendinopathy. Developing both diagnostic and interventional skills using high-resolution ultrasonography will become increasingly important as regenerative medicine and tissue engineering techniques and protocols continue to emerge. Sensitivity figures of 0.98 are reported when using a 10-MHz or greater frequency ultrasound probe when detecting full-thickness rotator cuff tears. It is becoming more commonplace for non-radiologists to become experienced with ultrasonography who can develop similar high levels of accuracy when diagnosing partial-thickness tears as compared with musculoskeletal radiologists, which is statistically better, than general radiologists and sonographers. Unfortunately, ultrasonography still remains user dependent and will continue to place demands on practicing physicians to improve diagnostic ultrasonography skills. Once the proper skill level is achieved, it will help ensure appropriate assessment and categorization of ligament and tendon injuries in order select the appropriate intervention to improve outcomes. In addition, regenerative medicine and tissue engineering techniques currently being used remain, for the most part, empirical and will require validation through clinical trials. The purpose of this article was to discuss basic concepts and strategies using a tissue engineering approach to soft tissue injuries of tendons and ligaments.
Tendon injury and pathophysiology
Tendinopathy can be described by specific location, which can play a role in determining the type of tendinopathy involved. Tendinopathy can be found within the main body of the tendon, its insertion site on bone (enthesis), and the structures around the tendon (peritenon). These different forms of tendinopathy can coexist within the same tendon.
Histology-based studies of the rotator cuff tendon, as well as other tendon injuries, have demonstrated the absence of acute inflammatory cells. Other studies are not in agreement with this and have found histologic evidence of inflammation. A normal tendon is extensible, which participates in a stretch-recoil cycle. However, in tendinopathic tendons extensibility is lost and becomes more rigid, which may complicate the control of joint position. Alteration of extensibility affects the capacity to respond to tensile loads and plastic deformation of tendinopathic tendons can lead to micro-injury or complete tendon failure. Several classification systems have been proposed based on histopathology.
Various etiologic theories have been proposed in the literature that divide causes into extrinsic and intrinsic factors. Extrinsic factors would include impingement syndrome, overuse, or multifactorial causes. Intrinsic factors include hypoperfusion theory, degenerative, degeneration-microtrauma, apoptotic theory, and extracellular matrix (ECM) modification. Rotator cuff tendinopathy, resulting in a tear requiring surgical repair, is particularly problematic. Repaired tendon tissue rarely achieves functionality equal to that of the insertion site of the original tendon to bone and is not regenerated after repair. Instead, the injured tissue is replaced with a “reactive scar” rich in collagen type III rather than collagen type I, which is mechanically weaker than the original zone of calcified cartilage at the enthesis formed during embryogenesis. Studies have demonstrated a high rate of failure of tendon healing following repair. The high rate of failure to heal after rotator cuff repair has led to a surge of interest in the use of regenerative orthopedic medicine techniques to augment the tendon repair and healing process.
Tendon healing is organized into distinct stages. Following injury there is hemorrhage and clot formation with platelet degranulation with release of cytokines and growth factors heralding the inflammatory stage of tendon healing. At that time, neutrophils and macrophages enter the hematoma and begin phagocytosis of necrotic material and pieces of the ECM (3–7 days after tendon injury). The proliferative phase is heralded by extrinsic cells from the peritenon, soft tissues, such as tendon sheath, fascia, periosteum, and subcutaneous tissues, but also intrinsic cells from the epitenon and endotenon migrate and proliferate at the injured site. Vasoactive and chemotactic factors are released that promote angiogenesis and stimulation of tenocyte proliferation and migration to the site of injury. In this process, granulation tissue is formed by mainly type III collagen. Initial collagen fibers are not initially parallel but still contribute to biomechanical strength. The amount of collagen progressively increases and in week 4, the fibroblasts from the endotenon proliferate. After 40 days, these intrinsic fibroblasts play a critical role in tendon healing and producing new collagen by recycling collagen. The tendon tissues mature and the fibers become more fully oriented according to tensile forces. The proliferative phase lasts approximately 2 months and biomechanical and tensile strength is restored in the remodeling phase when collagen is aligned in the longitudinal axis and cross-linking is complete. The newly remodeled tendon remains hypercellular and type III collagen is gradually replaced by type I collagen, which is more cross-linked to withstand tensile loads. There are numerous growth factors and cytokines that modulate the various healing cascades during the tendon repair process ( Fig. 1 ).
Insertional tendinopathy represents another unique pathophysiologic state in tendons. Typically, the superficial tendon layer is healthy and the deep layer is where the enthesopathy or insertional tendinosis exists. The superficial portion of the tendon is consistently under a greater strain than the deeper layer because the superficial portion is a further distance from the central axis of rotation of the joint. Insertional tendinopathy results from the differential effects of mechanical stimulation. Some orthopedic researchers have proposed a “stress-shielded” model in which an incision of the superficial layer redistributes the load equally from the deep to superficial portions of the tendon, thus the re-stressing causes a recovery of the tendon’s mechanical properties.
The presence of different strain patterns within a tendon opens up the potential for altering mechanical loads provided in certain joint positions that are more likely to stress a specific area of the tendon commonly affected by tendinopathy. Understanding the sequential progression of loading strategies applied to a tendon post regenerative injection procedure may be an important component of the “regenerative medicine” paradigm that can apply a controlled stress or mechanical load that can progress during the different phases of healing to improve outcomes. Significant work will need to be done in the future to prove which specific movement patterns or rehabilitation protocols are more efficacious to improve tendon and ligamentous healing.
Ligament injury and pathophysiology
A normal ligament is primarily composed of type I collagen, which is responsible for the tissue strength and resilience. This collagen is densely packed and cross-linked, which accounts for the stability and strength of the ligament. Following injury similar to what has been described in tendon injury, healing fibroblasts during the proliferative phase synthesize type III collagen rather than type I, which is produced in a much smaller quantity during this phase of tissue healing. During the healing process, the production of abnormal cross-linked collagen and smaller-diameter collagen fibers, as well as the reduction of tightly packed collagen fibers together produce a ligament with reduced stiffness and strength thereafter. Studies of experimental medial collateral ligament (MCL) injuries have demonstrated the injured ligament exhibits greater stress relaxation. This makes the healing ligament less efficient in maintaining tensile loads than a normal ligament and it ends up with abnormal “creep properties.” This pathophysiological processes allows the ligament to elongate more under tensile loads. Following ligamentous injury and subsequent laxity of the joint leads ultimately to osteoarthritis of the joint. Ligament injuries in athletes often lead to the inability of the injured athlete to participate at the preinjury level of activity, even after a decade from the date of injury. In addition, a common sequelae of ligament injury is a reported early onset of posttraumatic osteoarthritis.
Approximately two-thirds of patients with ACL injury are treated with ACL reconstruction. Although the objectives of undergoing ACL reconstruction surgery is to reestablish function and prevent subsequent damage to the articular cartilage and other soft tissues, there is mounting evidence that articular cartilage degeneration progresses despite surgery and/or different surgical technique. The primary factor responsible for osteoarthritis progression after ligamentous injury is joint instability and the associated increased shear motion between the articular cartilage surfaces. ACL injury, in particular, the envelope of joint motion becomes greater producing a tibio-femoral offset increasing shear stress at the cartilage-bone interface. This in turn may increase the external adduction moment and increase the load in the medial compartment, which accelerates arthritic progression. The loss of function, stability, and performance leads to the onset of osteoarthritis progression. This has led us to search for methods of treatment that restore the properties of normal ligament function and prevention of posttraumatic osteoarthritis.
The use of diagnostic and interventional ultrasound
Diagnostic musculoskeletal ultrasonography is becoming a commonly used office-based procedure and diagnosing tendon and ligament injury and pathology. This imaging modality is quickly becoming a critical diagnostic and interventional tool for physicians specializing in regenerative injection therapies. Precision diagnosis is a critical component of therapeutic intervention and it is important for physicians deploying biocellular interventions, tissue growth factors, ECM and scaffolding, and other interventions within a ligament or tendon. Diagnostically, ultrasound has particular value in demonstrating the pathophysiologic state of a tendon or ligament. Modern diagnostic ultrasonography, especially with high-resolution capacity, can display the entire physiologic spectrum of pathologic states in soft tissue, such as ligaments and tendons in all phases of healing. The pathophysiologic state of the tissues is translated into detectable echodensity and echogenic changes easily detected by high-resolution ultrasonography. In addition, diagnostic ultrasound also has proven value to be able to perform dynamic examination of the integrity of ligaments and soft tissues during motion testing. We have discussed previously the change in “stiffness” of tendinopathic tendons. Tissue elastography provides a means for the modern ultrasonographer to identify changes in “tissue stiffness,” identifying areas of focused tendinopathic changes within the tendon or specific tissue that may escape detection during a static 2-dimensional (2D) examination of gray-scale differences. The use of shear-wave elastography and the more recent development of strain elastography and 3D elastography in assessing tendon pathology is still being defined. This new technology shows promise further evaluating tendon and soft tissue pathology and may become a means to monitor outcome of regenerative interventions in the future. The higher the resolution and processing power the more definition and resolution is achieved in evaluating fibrocartilage structures in the knee, such as the meniscus. The meniscus fibrocartilage is composed of densely packed collagen fibers interposed with chondrocytes. This cellular architecture is responsible for the homogeneous hyperechoic appearance seen on ultrasonography. Although ultrasound cannot penetrate deep into the joint proper to directly visualize the entire meniscus structure, there are multiple ultrasonography findings that would suggest underlying cartilage injury. Para meniscal cysts can be easily identified and are frequently seen as a hypoechoic and occasionally anechoic structure adjacent to the meniscus. Our empirical experience has involved the use of ultrasound to evaluate the meniscotibial, meniscal capsular ligament attachments, peripheral tears in the meniscus, and dynamic examination of the ligamentous attachments of the meniscus to evaluate meniscus extrusion and instability with valgus and varus stress maneuvers. These various findings provide a classification scheme for different interventions based on pathophysiology and clinical findings.
Many articles have been published defining the criteria for partial and full-thickness tears of the rotator cuff. The sensitivity and specificity of diagnostic ultrasound in rotator cuff pathology is also well established, as well as the pitfalls associated with use of ultrasound in this regard. Although ultrasonography has demonstrated that sensitivity can be as high as 100% for full-thickness tears similar to MRI, ultrasound has a lower sensitivity for diagnosing partial-thickness tears. It is the process of stratification of ligamentous and tendon pathology by clinical examination and ultrasonography that provides a basis for therapeutic intervention using regenerative injection procedures. The use of ultrasonography and stratifying or classifying specific tendon pathology or ligamentous pathology requires a significant skill level and is still user dependent.
Strategies for regenerative injection therapies and augmented tendon and ligament healing
There have been an increasing number of physicians in various disciplines turning to the use of platelet-rich plasma (PRP) or other cell-based injections for soft tissue injuries or degenerative pathology within tendons and ligaments. The authors having spent many years performing such interventions and are beginning to deemphasize the importance of stem cells or specific cellular intervention and emphasize the importance of a “tissue engineering” approach to tendon and ligamentous pathology. This approach is multifactorial, as noted in Fig. 1 . A tissue engineering approach requires the physician to match a precision diagnosis with the biocellular intervention. This requires not only the selection of the specific cell type and source that is most appropriate for a specific tissue pathology or injury, but the selection of appropriate adjuvant growth factors and ECM proteins to more effectively augment healing. Physicians using a tissue engineering approach should be readily aware of when and when not to use specific biocellular intervention based on a given pathologic state in the tissue. They can then select specific adjuvant ECM proteins, bioactive scaffolding, cellular preparations and growth factors to enhance healing and promote cell differentiation to a targeted tissue. It is also critically important that the regenerative orthopedic medicine practitioner develop a keen understanding of physical rehabilitative medicine principles that should be used after their intervention. Specific gradual soft tissue loading strategies following an intervention can have a profound effect on tissue healing. Physical medicine and rehabilitation principles also require an understanding of the use of orthotics, bracing, and splinting and physical modalities that may be beneficial for short-term recovery from an intervention before and/or after the procedure.
Platelet-rich plasma and autologous platelet products
PRP is the most commonly used autologous source of a variety of growth factors used in regenerative orthopedic procedures in an attempt to augment tendon and ligament healing. Both plasma and platelets are rich sources of bioactive proteins, including growth factors, ECM proteins, and other signaling molecules. Platelets contain granules: α, δ, and λ. Alpha (α) granules play a pivotal role in platelet function. There are approximately 50 to 80 α granules per formed platelet and contain more than 30 proteins, including platelet-derived growth factor (PDGF), transforming growth factor (TGF-β, β1 and β2 isomers), platelet factor 4 (PF4), interleukin-1 (IL-1), platelet-derived angiogenesis factor (PDAF), vascular endothelial growth factor (VEGF), epidermal growth factor (EGF), platelet-derived endothelial growth factor (PDEGF), epithelial cell growth factor (ECGF), and insulinlike growth factor (IGF). The properties of PRP are based on the production and release of these factors when the platelets are activated. Dense granules within platelets contain neuromodulators and inflammatory modulators, such as histamine and serotonin. By exposure to collagen, thrombin, or calcium, platelets release growth factors and cytokines.
PRP has been used for years as a source of growth factors to augment soft tissue healing, but this has not been without problems. Physicians using high-resolution ultrasound and PRP injections to evaluate and treat tendinopathic tendons and tendon can attest to the fact that tendons are difficult to heal and tendons can fail to heal with a single intervention. It is commonplace that patients need to be reevaluated to determine if there has been interval resolution of tendinopathy or healing of a tear. Careful documentation under ultrasound examination may demonstrate the need for either multiple interventions or a change in strategy to augment tendon healing. We remain cognizant of the fact that in some studies a single PRP injection compared with placebo controls has found no significant difference between the study and control groups. Similar results were reported by Rodeo and colleagues. Despite some negative studies, there continues to be evidence to support the use of platelet products in clinical practice. For example, surgical repaired flexor tendon injuries in the forelimb of horses demonstrated a higher failure strength with greater elastic modulus 24 weeks postoperatively after being treated with PRP compared with the control group with only saline injection. Histologically, the PRP group demonstrated better collagen organization and increased metabolic activity. It was noted in this study, that the PRP group demonstrated better collagen organization and increased metabolic activity.
Inconsistency reported in various studies could prove that there is not a clear dose-response relationship using PRP. High PRP concentration may support wound healing, yet some investigators think that overdosing PRP can lead to poorly differentiated scar tissue by overstimulating cells and the release of growth factors in such a high concentration may have negative effects causing increased inflammation. Another natural source for a variety of growth factors, including anti-inflammatory cytokines, would be autologous conditioned serum (ACS), which contains anti-inflammatory cytokines or interleukins (IL-4, IL-10, IL-13) and IL-1 receptor antagonist in addition to growth factors basic fibroblast growth factor (bFGF), hepatocyte growth factor (HGF), and TGFb. It was noted that there was a positive effect of ACS in Achilles tendon healing with accelerated organization of repaired tissue, increased collagen mRNA, increased collagen deposition reflected by tendon thickness, and increased mechanical strength. Despite this, the repaired tendon remained biomechanically inferior to native tendon controls.
No doubt, growth factors play an important role in the healing course of tendon tissue. Clinicians using growth factors as an attempt to augment tendon or ligament healing face a number of problems, such as which specific growth factors could be used as standalone therapy or what combinations of growth factors should be used appropriately for a given clinical presentation. This has not been established in various research protocols. In addition, the short duration of action of many growth factors limits the potential long-term effects using injection therapy, especially in lieu of the fact that healing tendons can take months or years to heal. The next conceivable step in research protocols would be to establish a specific protocol or model by using a combination of growth factors, biocompatible scaffolds, and specific biocellular intervention. In vitro studies have demonstrated that the addition of PRP to human tenocytes resulted in cell proliferation, collagen deposition, and improved gene expression for matrix-degrading enzymes and endogenous growth factors. We can also stimulate tendon healing by a well-ordered angioneogenesis. These investigators highlight the importance of understanding the timing of an administration of growth factors and their dosage for designing effective growth factor therapy. It is of critical importance that we continue to perfect specific protocols and to create selection criteria for interventions for the future of regenerative musculoskeletal medicine.
In clinical practice, physicians specialized in diagnostic and interventional ultrasound in musculoskeletal (MSK) medicine have the capacity to repeat evaluations and monitor clinical outcomes. By repeated clinical evaluation and ultrasonography, examination can make appropriate changes to the treatment regimen as necessary, which may include repeat interventions to improve clinical outcomes. This is difficult to duplicate in research protocols and therefore empirical experience and utilization of regenerative procedures continue to outpace research evidence for the use of various biocellular and biologic intervention.
Plate-Rich Plasma Classification and Terminology
Because there are many protocols for preparing PRP and the products of these preparation methods differ in both cellular and molecular composition, it is becoming increasingly important for physicians to classify the type of PRP or platelet preparation that is being used for a specific intervention. Several classification systems exist, but more importantly regardless of the classification system used, it is critically important to select the appropriate platelet preparation for the pathophysiology or condition being selected to treat. The initial classification was published in 2006 by Mishra. Mishra and colleagues proposed a new classification system. This classification system included identifying type A PRP with platelet concentrations less than 5 times blood concentration and type B PRP with platelet concentrations 5 times normal blood concentration. Simply speaking, PRP categories were noted as pure PRP (P-PRP) and leukocyte-rich PRP (L-PRP). This classification was later noted to be insufficient because different PRP centrifuge devices create platelet products with different platelet cell counts and leukocyte counts, which has potential repercussions on the biologic effect and clinical outcomes. Not long after Mishra’s classification system was published, Dohan and colleagues published their classification system. However, this was really aimed at the surgical community and divided PRP and platelet-rich fibrin products that at the time were more important in creating surgical grafts to be used in the surgical application of tissue repair in the operative setting. Then the platelet activation white blood cell (PAW) classification system was published by DeLong in 2012 leading to 32 PRP categories. This system requires a platelet concentration range reflecting a close proximity to the actual platelet quantity, the activation mode in the presence of white cells, and so forth. With the advent of the “Double-Spin Suspension” method of creating a pure PRP, we now have a PRP product that is high in platelet concentration with few to no red blood cells (RBCs) and an elevated or baseline leukocyte count with a significantly reduced neutrophil count. This is in contrast to the P-PRP, which has a low platelet count of 1 to 3 times concentration and no leukocytes or RBCs. Most of the research to date has included L-PRP versus P-PRP. To date there has not been a peer-reviewed human study featuring a double-spin suspension method pure PRP yet. However, this method has gained popularity in clinical practice and the investigators have extensive experience using this method as their go-to product with good clinical experience, anecdotal to date. One of the investigators participated in a new PRP classification article published in the spring of 2015 that took into account all known factors that may be important to differentiate when studying the PRP literature and more importantly when designing future PRP research studies. In this latest classification article, the investigators pointed out it is not enough to report the concentration of the PRP used to treat a subject as “times × baseline platelet count” because a 5 times baseline platelet count in a subject with a baseline platelet count of 160,000 is much different from a subject with a baseline platelet count of 340,000 platelets per microliter. Therefore, the new recommendation for classifying a platelet treatment when evaluating an article or designing the next PRP study is to adopt the PLRA system. “P” stands for platelet concentration as expressed in platelets per microliter and the volume of PRP delivered to the target should be recorded as well. That way, an actual dose of platelets can be determined and compared between similar studies. Next the presence or absence of “L” for leukocytes should be recorded and if leukocytes are present the concentration of neutrophils should be recorded as well. The next symbol is “R” for RBCs should be recorded as present or not, and finally “A” for activation should be recorded if the sample was exogenously activated or not.
Without the research details regarding the biochemical features and qualities of the double-spin suspension method of the newest pure PRP system available, we can make some observations of the well-studied L-PRP versus the P-PRP systems available to date. L-PRP can be detrimental to healing of injured tendons because it induces catabolic and inflammatory effects on tendon cells and may prolong the effects of healing tendons. On the other hand, when considering P-PRP may not have the desired effect on acutely injured tendons and may result in the formation of excessive scar due to the potential of P-PRP eliciting a cellular anabolic effect. L-PRP elicits more extensive catabolic response in differentiated tenocytes and induces a higher level of inflammatory response. L-PRP induces the expression of type III collagen, which as we have already discussed involves early granulation tissue formation. P-PRP elicits the expression of type I collagen, which is more desired in mature ligament and tendon tissue. Thus, the utilization of L-PRP may be deleterious to chronically injured tendons or chronic degenerative tendinosis, but may be beneficial during the acute phase of healing. P-PRP may be more appropriate for induction of type I collagen in the proliferative phase or remodeling phase of healing. Because L-PRP induces inflammatory responses and differentiated tenocytes, using L-PRP to treat already-inflamed tendinopathic tendons may only exacerbate the tendon disorder by prolonging the inflammatory phase and thereby impairing the healing process. It may be more appropriate to use P-PRP to augment the repair of tendinopathic tendons because of its anabolic properties and low inflammatory effects. Because of the anabolic effect of P-PRP, its use in acutely injured tendons may elicit fibrosis or scar formation because of induction of tenocyte stem/progenitor cells to produce too much collagen at the injury site. Thus, one needs to exercise caution in using P-PRP in acutely injured tendons. Gene expression of IL-1 beta, IL-6, and TNF-alpha is upregulated by L-PRP; thus, induction of catabolic effects on tendon cells could be detrimental to effects on injured tendons and impair healing. Because P-PRP elicits anabolic effects, its probably best suited use would be in late-stage healing, thus enabling it to augment and accelerate tendon healing. The effects of leukocyte-rich versus leukocyte-poor and its effect on the side occurring cascade may explain, in part, the variable outcomes of PRP treatment in clinical trials.
Platelet lysates and platelet releasates use in augmented tendon and ligament healing
Platelet Lysate
A platelet lysate involves the preparation of a platelet concentrate in which platelets are centrifuged into a pellet and exposed to freezing temperatures, thereby lysing the platelet cell membrane, releasing platelet growth factors from the alpha granule and reconstituting in plasma. The plasma is then filtered and a growth factor–rich plasma is created. This has been widely used in cell cultures, especially mesenchymal stem cell (MSC) cultures, as a replacement for fetal bovine serum. The authors discontinued the use of lysates when it was discovered the number of unaffected platelets by the cryolysis was a variable percentage even with triple freeze thaw cycles. We empirically appreciated platelet releasates affected 100% of the platelet population as verified with our own laboratory-based platelet counters and thus the release of much more growth factors. This will need to be documented in further studies.
Platelet Releasates
A platelet releasate involves the production of a platelet concentrate or PRP. Platelets are then activated with thrombin, calcium chloride, or collagen. Platelet lysates and releasates have been used for years for their growth-promoting effects in cell cultures. Releasates are used for the alpha-granule–derived growth factors, such as PDGF, TGF-beta, IGF, VEGF, FGF-2/b, and EFG. A platelet releasate was investigated with regard to its effect on TSC (tenocyte stem and progenitor cells). This involved activating platelets with calcium chloride rather than thrombin. It was noted that the platelet releasate promoted TSC proliferation and differentiation into active tenocytes as well an increase in collagen production.
The use of stem cells in ligament and tendon injury
In 1994, adult MSCs were discovered to play an active role in connective tissue repair. Since that time, multiple sources of stem cells have been identified and studied, including tenocyte-derived stem cells, adipose-derived stem cells, amniotic-derived cells, dermal fibroblasts, and bone marrow–derived MSCs. The theoretic basis for current clinical application of stem cells is to use these cells as a reservoir for musculoskeletal tissue repair. The most common clinical sources of stem cells are from bone marrow aspiration or from lipoaspirates. These cells delivered to a precise location, typically with ultrasonography, rely on local growth factor signaling or the addition of exogenous factors to drive the stem cells to differentiate into the target cell line. Most adult bone marrow consists of blood cells in various stages of differentiation. These components can be divided into plasma, RBCs, platelets, and nucleated cells. The adult stem cell fraction is present in the nucleated cells of the marrow. Bone marrow concentrate (BMC) is produced by density gradient centrifugation of bone marrow usually aspirated from the iliac crest. However, actual stem cell numbers from bone marrow are less than 0.01% or 1/100,000 nucleated cells. Its major function is to deliver MSCs to the injury site. Like PRP, BMAC is also rich in platelets and therefore growth factors. With the ease of marrow blood aspiration it can be readily accessed by surgeons intraoperatively or within the clinical setting in a timely fashion. Because of the ease of bone marrow aspirate techniques, there has been clinical utility and various strategies used to influence the cells down a tenogenic line, including the application of growth factors. Some investigators feel there is mounting evidence to show that bone marrow stem cells (BMSCs) used with specific protocols to differentiate into a tenogenic line can produce tendon tissue when exposed to the appropriate environmental cues. There is evidence that bone marrow–derived MSCs only act as a signaling cell to the local tissue niche and do not affect tissue healing by differentiating into a specific cell lineage but rather induce healing by a cell-signaling mechanism that affects the local resident stem cells in the niche and do not differentiate into the repaired tissue.
An intriguing study was published by Hernigou and colleagues, in which they followed 90 patients following rotator cuff repair. Forty-five patients received bone marrow aspirate concentrate injections with rotator cuff repair intraoperatively and 45 patients received rotator cuff surgical repair only. These patients were followed for 10 years with ultrasound and MRI. At 6 months’ follow-up, the study group that received the combined BMC and surgery were 100% healed versus 67% of the control group. At 10-year follow-up, the study group that received the combined BMC and surgery demonstrated that 87% of the study group maintained an intact tendon versus 44% of the control. Several other studies have also demonstrated the ability of MSCs to improve healing of tendons or ligaments. Despite these positive findings, there are studies that have failed to show the application of bone marrow–derived, culture-expanded MSCs to augment the healing process of rotator cuff tendon tears despite evidence that they are present and metabolically active. Laboratory studies of injured tendons in rats demonstrated the addition of MSCs to a rotator cuff injury model did not improve the structure, composition, or strength of the healing tendon attachment site.
Adipose tissue–derived stem cells (ASCs) have shown promise in animal models of tendon repair. Like bone marrow, adipose tissue is also derived from embryonic germ layer mesoderm. Adipose tissues contain a relatively large number of undifferentiated cells, which include stem cells and progenitor cells capable of producing tissues arising from embryonic mesoderm, such as cartilage, ligament, tendon, muscle, and bone. ASCs have been shown to have an increased angiogenic capacity compared with bone marrow. Despite the excellent availability of MSCs in adipose tissue, isolating the cells from adipose stroma (stromal vascular fraction), typically requires a process of enzymatic digestion. This process has come under scrutiny of the Food and Drug Administration (FDA) and at present is considered a violation of the “more than minimal manipulation” rule set forth by the FDA. It is anticipated that multiple techniques will emerge that will satisfy FDA regulations and yet provide physicians with a safe means to extract MSCs from adipose tissue in sufficient numbers to have clinical utility in a same-day surgery setting.
ASCs have been of significant interest in musculoskeletal orthopedic application. Some researchers have questioned the utility of ASCs in ligament tissue engineering and were unable to demonstrate expression of ligament markers with ASC placation. ASCs have been shown to be as effective as other MSCs by their multipotency and proliferation capacity. It is believed that ASCs present an ideal cell source for experimental and clinical research on tendon engineering. Experimental studies of tendon repair have shown ASCs to exhibit a significant increase in tendon cell strength, direct differentiation toward tenocytes and endothelial cells, and an increase in angiogenic growth factors. These findings suggest that ASCs may have a positive effect on primary tendon repair and may be useful for future cell-based therapy, although most existing clinical information to date has been generated using culture-expanded marrow-derived MSCs. There is information to suggest that MSCs from fat, placenta, umbilical cord, and muscle have similar, but not identical functional potential. Despite this, the question of which exogenously supplied MSC source might be optimal for a given clinical situation has not yet been established. In addition, researchers still need to sort out which biocellular intervention and tissue engineering strategy is most efficacious. It will also be necessary to define and characterize tendinopathy and tendon tears, and which of these lesions is the most amenable to a specific cellular medicine intervention protocol.
The use of extracellular matrix proteins and tissue scaffolding
The use of ECM proteins and scaffolding to augment tendon and ligament healing is becoming an important strategy in tissue repair. In tendon injury and repair for example, the bone-to-tendon interface after healing has little resemblance to the native tendon fibro-osseous insertion site; instead, the normal 4 distinct zones of the tendon insertion are joined by a layer of fibrovascular scar tissue composed of predominately type III collagen. This tissue is significantly weaker than the original insertion and contributes to the substantial number of failures through both natural and surgical repair. Therefore, a scaffold is one particular strategy that may provide a suitable mechanical and biological environment for cells to migrate into and provide a bridge between the tendon and bone or the tendon defect to facilitate the alignment of cellular proliferation and collagen deposition. There are many scaffold-based and biological approaches that have been investigated in an effort to improve tendon and ligament healing and provide mechanical integrity. This would include natural and synthetic matrices for cell and tissue in-growth. One of the problematic issues using allogeneic ECMs is induction of inflammatory responses in the host. Therefore, it is important to select the proper ECM or scaffolding strategy for the type of target tissue, phase of healing, and extent of injury. Fibrin gel, for example, offers a few advantages in these situations because of the properties of fibrin, such as controllable degradation rates, that can match tissue regeneration, good biocompatibility, adhesive properties, and nontoxic degradation byproducts. Fibrin gel self-assembles into a scaffold by mimicking the last step of blood clotting to support cell, proliferation, differentiation, and tissue regeneration. It can also be used as a cell carrier to protect cells from the forces produced during preparation and delivery processes. Fibrin gel also provides high cell-seeding efficiency and uniform cell distribution. The degradation rate of fibrin gel can be regulated with aprotinin and tranexamic acid (trans-4-aminomethyl-cyclohexane-1- carboxylic acid) to precisely match tissue regeneration.
Decellularized adipose tissue (DAT) as a bioactive matrix within a hydrogel is also being explored as a scaffold and means of regenerative cell delivery within an ECM matrix. An injectable composite scaffold incorporating DAT as a bioactive matrix within a hydrogel phase capable of in situ polymerization would be advantageous for ASC delivery in the filling of small or irregular soft tissue defects. Nonoperative musculoskeletal practitioners are beginning to become more interested in injectable scaffolding to fill tissue defects with combination with platelet, stem cell, and other biocellular interventions.
There is significant work being done with regard to implantable bio-scaffolds that are either now approved for operative tendon and ligament repair or being used in animal models to evaluate their potential use. In the near future, implantable scaffolding membranes will be available using minimally invasive techniques that will be placed under ultrasound guidance. Amnionic membranes in vivo and in vitro studies are beginning to demonstrate that the biochemical properties of amniotic membranes can help reduce inflammation and enhance soft tissue healing.
Hyaluronic acid (HA) is demonstrating increased cell viability and proliferation, in a dose-dependent manner. Importantly, HA also stimulates the synthesis of type I collagen without an increase in type III collagen. Because of tenocyte apoptosis and decreased collagen synthesis, there is an increased risk of tendon rupture. HA in different concentrations can have a modulation effect on cell migration, adhesion, and proliferation.
Another novel ECM that has become available for use in orthopedic regenerative injection procedures is a flowable tissue matrix allograft derived from human placental connective tissue. The human placenta is a rich source of biological tissue and the ECM components contain collagens (types I, III, IV, V, and VI), fibronectin, nidogen, laminin, proteoglycans, and hyaluronan, as well as growth factors. Another important advantage to placental tissue sources of ECM proteins is that they are nonimmunogenic, which elicits little to no immune response. We present several case examples in this article in which this novel tissue matrix was used to treat ligament and tendon pathology.
Implantable collagen matrix for tendon healing
The composition of collagen is altered in injured tendons as well as upregulation of proinflammatory cytokines, which ultimately effects the tensile strength of the healing tendon and thus perpetuating potential future failure. Implanting a collagen matrix graft can initiate the healing cascade, which can facilitate tendon healing and alter the cytokine environment in which the matrix graft is placed. Orthopedic literature has demonstrated that healing of the rotator cuff following rotator cuff repair is problematic. Implantation of bio-scaffolding is currently being explored as an important component of the biologic approach to successful rotator cuff tendon repair. It has been proposed that the use of a bio-inductive purified bovine collagen implant can be applied over the bursal surface of a partial rotator cuff tear using arthroscopic deployment ( Fig. 2 ). The application of a collagen tissue matrix graft applies a small amount of tension along the tendon, which functions as a bioreactor rather than a full tendon anchor. Mild tension applied by the tissue graft causes induction of tissue healing. The membrane composed primarily of collagen and implanted under arthroscopic technique initiates bleeding and therefore the activation of platelets in the clotting cascade secondary to exposure of the bare collagen within the implant. The resulting clot encompasses the implanted membrane activating a cytokine cascade that is thought to promote healing. Orthopedic researchers reviewing data from sheep tendon models and computer remodeling help demonstrate that new tissue reduces peak strain at the partial tear site and may help with the balance of biologic activity and the biomechanical aspect of healing tendons. They demonstrated when using the collagen matrix graft that in clinical trials of 24 partial rotator cuff tears that the implant consistently induced new tendon tissue in all 24 subjects. Healing was verified by serial MRI demonstrating new tissue that was well integrated within the host tissue and progressive tissue maturation.
The use of tissue growth factors in augmented tendon and ligament healing
The tendon healing process is a complex orchestration of cellular biology that is heavily influenced by numerous secreted molecules. Table 1 on the right is a brief summary of just a few of the growth factors and biocellular processes that are involved in the phases of healing a tendon and ligament. We provide only a cursory review of growth factors that influence tendon and ligament healing that are currently under investigation as a potential means to augment the healing cascade.
Transforming Growth Factor β 1
Transforming growth factor beta 1, for example, is likely to be involved in the mechanism of scar tissue formation in the presence of recruited macrophages in the inflammatory phase of healing. However, its application to injured tendons, although yielding increased tissue proliferation, did not contribute to increasing tendon strength.
Bone Morphogenic Protein-12
Bone morphogenic protein (BMP) 12 and 14 are expressed in active fibroblasts and are present throughout the healing process of bone-to-tendon insertion. BMP-12 induces the formation of tendon and ligament like tissue and is involved in the differentiation of stem cells to tenocytes.
Bone Morphogenic Protein-14
BMP-14 plays a role in tendon collagen organization. It has been shown when introduced into rat Achilles tendon to induce ECM synthesis and cellular proliferation and increase expression of ECM and cell adhesion–related genes.
Other Bone Morphogenic Proteins
BMP-2 and BMP-7 were shown to induce collagen production when added to cultured tenocytelike cells derived from samples of human rotator cuff (RC) tendons. For these reasons, the ability of BMPs to augment RC repair is currently under investigation. Studies in sheep and rat models have shown BMP therapy alone is beneficial for RC repair.
Insulinlike Growth Factor-1
Insulin growth factor-1 (IGF-1) is an anabolic polypeptide that directly affects all major cell types involved in soft tissue healing. IGF-1 not only affects muscle cells but also tenoblasts, ligament fibroblast, and other fibroblastic cell types and also induces angiogenesis required for wound healing. The singular loss of IGF-1 can have drastic effects on wound cell proliferation, decreased collagen, and hydroxyproline concentrations. No other growth factor is known to singularly affect positive wound-healing parameters to the same degree as IGF-1. IGF-1 stimulates protein synthesis and cell proliferation while decreasing swelling and has been observed in the healing of the bone-to-tendon junction.
Basic Fibroblast Growth Factor
Basic fibroblast growth factor (bFGF) increases proliferation and expression of collagen type III and can facilitate BMSC proliferation and differentiation into tenocytes. bFGF has been shown to be a potent stimulator of angiogenesis, cellular migration, and proliferation in both in vivo and in vitro studies.
Platelet-Derived Growth Factor
PDGF-b has been proposed to be a critical cytokine in the repair of tendons and ligaments. PDGF-b has been shown to promote chemotaxis, cell proliferation, ECM production, surface integrin expression, and revascularization in fibroblasts. In a sheep RC repair model, scaffolds infused with PDGF-b demonstrated improved histologic scores and ultimate load-to-failure compared with repairs using empty scaffolds alone.
Vascular Endothelial Growth Factor
The potent angiogenic effect of VEGF has been well documented. This may be important because impaired blood supply in tendons leads to apoptosis in tendons. VEGF activity rises after the inflammatory phase. VEGF in RC repair has been shown to improve tensile strength in animal tendon healing models with VEGF augmentation.
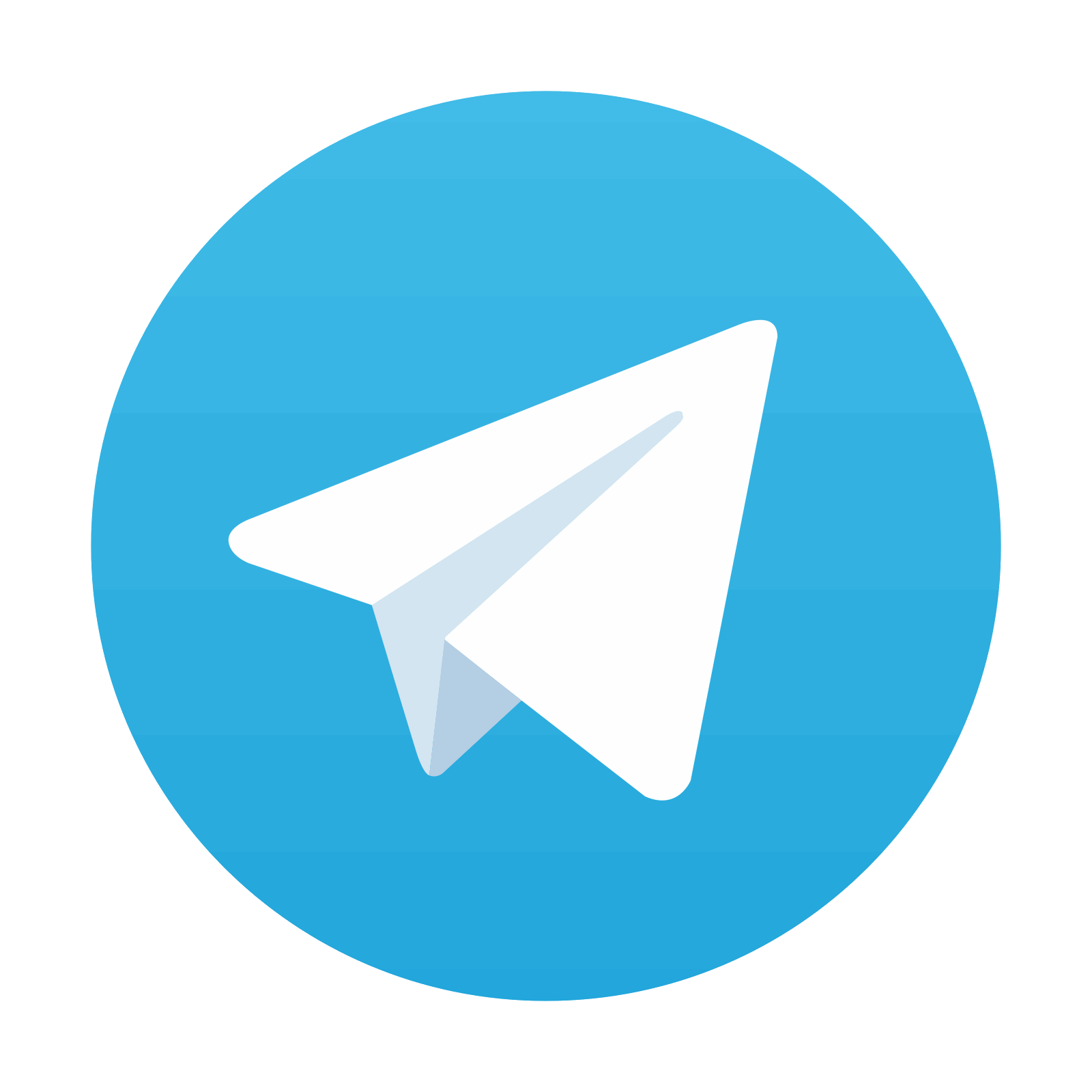
Stay updated, free articles. Join our Telegram channel

Full access? Get Clinical Tree
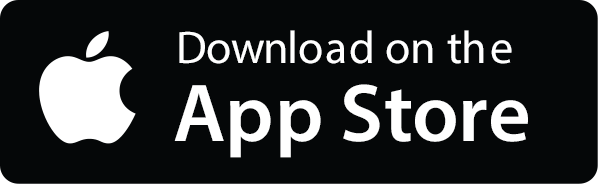
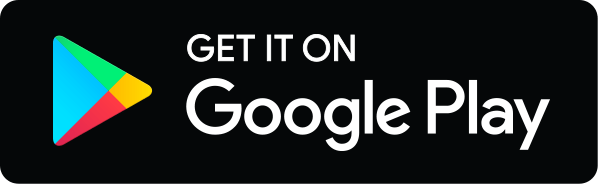