Radiation Exposure in Minimally Invasive Spine Surgery
Christina Kane
Christian P. DiPaola
Minimally invasive spine surgery (MISS) procedures typically require increased use of intraoperative imaging, which may include x-ray or computed tomography (CT) scan. Reliance on smaller incisions and less exposure often means that the surgeon is trading off visual and tactile feedback offered by a standard open approach for increased intraoperative imaging. Imaging is often more heavily relied upon for the purposes of anatomic localization, instrumentation placement, and deformity correction, resulting in potentially increased radiation exposure to operating room personnel and patients. Spine surgeons should have a good understanding of strategies used to minimize radiation dose to both themselves, their OR staff, and patients. The goal of the surgeon and team should be to reduce the risk of short- and long-term complications associated with radiation exposure. There is no such thing as too little radiation exposure.
RADIATION PHYSICS
Radiation is the emission or transmission of energy in the form of waves or particles through space or a material medium. It can be further divided into two categories: ionizing and nonionizing radiation. Ionizing radiation occurs when these particles or waves carry enough energy to free electrons from atoms. Gamma rays, x-rays, and the higher ultraviolet part of the electromagnetic spectrum are ionizing, whereas the lower ultraviolet part of the electromagnetic spectrum, visible light (including nearly all types of laser light), infrared, microwaves, and radio waves are considered nonionizing radiation. Ionizing radiation is utilized in various types of imaging modalities including x-rays, CT, positron emission tomography (PET), angiography, and radionuclide imaging. In medical imaging, the amount of ionizing radiation deposited or absorbed per unit mass of tissue is classified as the absorbed dose and measured in grays (Gy). One gray is defined as the absorption of 1 J per kg of radiation to the target tissue.1 The equivalent dose, or the actual quantity of absorbed dose imparted to the target tissue to cause a biologic effect, is dependent on the type of radiation and amount absorbed. The risk of induction of cancer from an equivalent dose is highly dependent on the receiving organ, as different tissues and organs have unique radiation sensitivities. The tissue-weighted sum of all equivalent doses to the irradiated tissue/organs exposed is known as the effective dose. It is measured in sieverts (Sv). One sievert is defined as the absorption of 1 J per kg of biologic tissue. For reference, a single posteroanterior (PA) chest radiograph imparts an effective dose of 0.03 mSv to the lungs, breasts, esophagus, and stomach, whereas a CT scan of the chest delivers more than 200× that dose (7 mSv).2,3
EFFECTS OF RADIATION ON BIOLOGIC TISSUE
Radiation exposure can be harmful to human tissues in both small and large doses, and its effects are categorized into two groups: deterministic effects and stochastic effects. Deterministic effects (nonstochastic) are described by a cause and effect relationship between radiation dose and damage, whereby the severity of the deterministic effect increases as the exposure dose increases. A radiation dosage threshold exists and, when exceeded, cell damage and death may occur. Previous studies have shown radiation doses of 2 Gy and 12 Gy to the skin can cause deterministic effects of erythema and necrosis, respectively. A dose of 4 Gy to the eyes may cause cataracts if received over a period of less than 3 months.4
Stochastic effects occur when low doses of radiation result in damage or mutation at a molecular level in a linear fashion. No threshold exists, but rather the risk of detrimental effects increases as the radiation dosage increases. For example, the risk of cancer from a single spine CT scan imparting an effective dose of 6 mSv is approximately 1:3,300.5
IMAGING MODALITIES
Minimally invasive procedures have become more common as spine technology and techniques have evolved. However, limited direct anatomic visualization during MISS often warrants generous use of imaging modalities for incision localization, instrumentation placement, and deformity correction. Large C-arm fluoroscopy is the most commonly used imaging modality for most minimally invasive procedures. However, there are alternative options that may help reduce the cumulative radiation exposure to the surgical staff and patients.
Mini and Large C-arm Fluoroscopy
Use of a mini C-arm fluoroscopy has the potential to decrease the radiation dose imparted to patient and surgeon. Using dosimeters, Giordano et al. reported reduced radiation exposure to the surgeon with use of a mini C-arm during imaging of a cadaveric cervical spine specimen when compared with the large C-arm.6 However, similar to large C-arm fluoroscopy, radiation exposure to the specimen increases as it is positioned closer to the radiation source and further from the image intensifier.5 There are obvious limitations to the usage of the mini C-arm and its potential application to the C-spine.
Computed Tomography
Some minimally invasive procedures utilize three-dimensional image-guided technologies in an effort to improve accuracy of instrumentation placement. Intraoperative cone-beam CT represents one such method. One study demonstrated that cone-beam CT scans of the wrist, when compared with multidetector CT scans, have the potential to decrease the CT dose index (a radiation dose measurement) by a factor of 7.5. Lange et al. assessed the effective radiation dose of cone-beam CT (Medtronic O-arm) scan based on a thoracolumbar spine model. The overall calculated dosages for full-length procedures (1 to 3 scans) using the O-arm standard mode, or “small-patient” setting, fell within the range of published effective doses of abdominal CT scans (1 to 31 mSv). The radiation dose increased with patient size, and more than three cone-beam scans in larger patients (24.27 ± 0.69 mSv) may exceed the radiation dose of a single standard abdominal CT scan. While a single-level instrumented posterior spinal fusion may require two scans for both navigation and placement of instrumentation, the difference in maximum imparted radiation effective dose in a “small-patient” setting is 8.24 mSv, compared with 20.54 mSv in a “large-patient” setting. Therefore, Lange et al. conclude that usage of the O-arm in most full-length posterior instrumented spinal procedures in smaller patients will not exceed the maximum effective radiation dose of 20 mSv per individual per year recommended by the International Commission on Radiological Protection guidelines. Unfortunately, the same does not hold true for larger patients.7,8,9
While the O-arm has the potential to reduce OR team radiation exposure when standing in a substerile room, exposure to the patient can be higher than with both C-arm and plain x-ray.10 O-arm imaging should be used with discretion, especially in patients who are at a higher annual and lifetime risk of radiation exposure. Trauma patients who undergo many CT scans in a short time frame can easily exceed 20 mSv per individual per year.8 Salerno et al. identified 249 trauma patients over a 4-year period who underwent more than one CT scan in a median of 4 days. Patients had a mean age of 29 years, and a mean estimated effective dose of 27 mSv.11 Similarly, it can be deduced that patients with scoliosis who receive many surveillance x-rays over the course of their life will have a higher lifetime radiation exposure. With such patients, extra care should be taken to minimize the use of imaging modalities that require multiple intraoperative scans.
SURGICAL TECHNIQUES
Lumbar diskectomy has been traditionally performed through an open midline surgical approach using either loupes or a microscope. Intraoperative fluoroscopy or plain film x-ray is often utilized to determine the correct operative level. The senior author typically uses two radiographs, one of which is used for determination of the incision and the other for confirmation of the level after the exposure is complete. During this process, the surgeon and the operative team can stand either behind a lead shield or in a separate substerile room to minimize radiation. In contrast, MIS approaches using tubular retractors often require the surgeon to be present holding the dilator or retractor during fluoroscopic imaging. Mariscalco et al. compared the radiation exposure between the surgeons who performed open versus MIS diskectomy and found the mean exposure to the thyroid, chest, and hand were 10 to 20 times higher in the MIS group. For example, while a single-level open microdiskectomy had a mean radiation exposure of 0.16 ± 0.22 mR to the thyroid and 0.21 ± 0.23 to the chest, the MIS counterpart had mean exposures of 1.72 ± 1.52 mR and 3.08 ± 2.93 mR to the thyroid and the chest, respectively.12 It should also be noted that during open cases, surgeons who were standing in the substerile room were exposed to radiation as well, with an average exposure of 0.2 mR per case.12 Given the cumulative effect of radiation exposure on overall cancer risk, surgeons should be aware that localization imaging alone is not inconsequential.
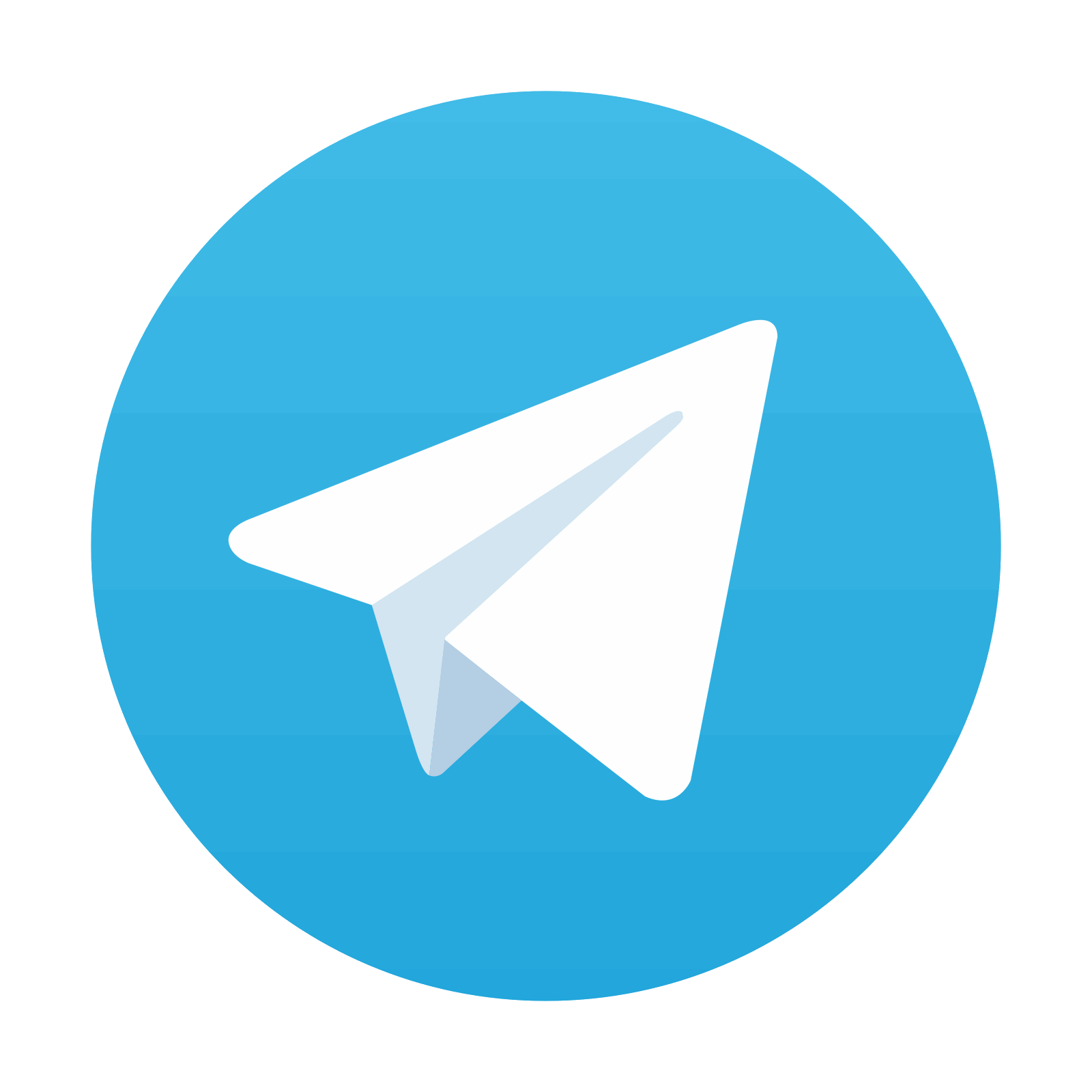
Stay updated, free articles. Join our Telegram channel

Full access? Get Clinical Tree
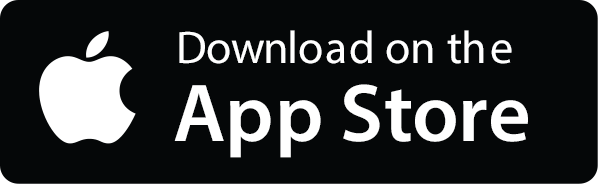
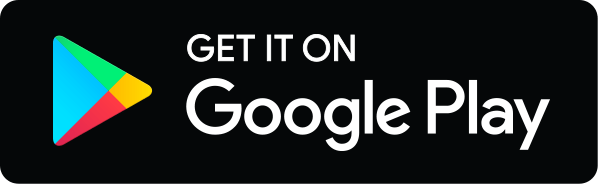