Patients with chronic pulmonary disease have increased rates of morbidity and mortality in addition to a lower quality of life. Pulmonary rehabilitation is a multispecialty comprehensive management program for these patients with the goal of improving pulmonary function, decreasing mortality and morbidity, and improving quality of life.
Key components of a pulmonary rehabilitation program include nutritional management, rehabilitation therapies, patient education, medication and oxygen delivery, and use of assisted ventilation. Common diseases that contribute to pulmonary dysfunction are reviewed in this chapter along with rehabilitation strategies. Finally, key outcome variables are reviewed.
The rehabilitation of asthmatics is a relatively new field. The Centers for Disease Control and Prevention (CDC) has reported the prevalence of asthma at 25.7 million in 20101 and increasing by 2.9% per year. Over 8% of children and adults are asthmatic. There were 1.8 million emergency department visits in 2011 and 10.5 million physician visits for it in 2012.2–4 However, the reversible episodes of wheezing, coughing, dyspnea, chest tightness, and deconditioning characteristic of asthma are amenable to medical and pulmonary rehabilitation (PR) interventions. Bronchodilators and corticosteroids are the mainstay of therapy5–8; these agents can reverse the inflammatory component9 and can significantly increase the forced expiratory volume in 1 second (FEV1) by 12% or more.10,11 Rehabilitation complements traditional management options and can significantly decrease exacerbation frequency and the need for frequent physician services and improve exercise tolerance and well-being.12,13
Asthmatics often require particular attention to nutrition to optimize body weight and to avoid the characteristic triggers of cold air and exposure to inhaled allergens (aeroallergens). Before beginning reconditioning exercise, inhaled glucocorticoids, often along with long-acting inhaled beta agonists, can increase exercise tolerance by preventing exercise-induced bronchospasm. Patients may also benefit from leukotriene modifiers, lipoxygenase inhibitors, theophylline, cromoglycates, anti-IgE therapy (omalizumab), and monoclonal antibodies (mepolizumab and reslizumab)14 (Fig. 50–1).
Fearing exacerbation of their asthma symptoms, asthmatics tend to become less physically active.15–17 The diminished activities of daily living (ADLs) decrease social interactions and quality of life and exacerbate deconditioning. Structured exercise programs can improve these and increase aerobic fitness and asthma control, as demonstrated in a 12-week supervised exercise program that included muscle strengthening and aerobic training including jogging, cycling, and elliptical/rowing machines.18 Additional evidence-based interventions, such as breathing retraining, can also improve quality of life, although further studies are needed to assess long-term benefits.19–21
Anxiety, depression, and feelings of loss of control are common in patients with poor asthma control.22 However, two randomized trials showed that exercise training for adults with moderate/severe persistent asthma improved asthma-specific health-related quality of life (HRQL) and reduced anxiety/depression and asthma symptoms for at least 3 months following training.23,24 A longitudinal study of over 2,800 female asthmatics demonstrated that greater regular physical activity can reduce the risk of asthmatic exacerbations independent of asthma severity.12 A prospective 12-month study of asthmatic adults performing outpatient-based weekly 60-minute sessions of moderate-intensity exercise resulted in improved cardiorespiratory fitness and HRQL.25 Use of bronchodilators and gradual warm-up before exercising were needed to minimize exercise-induced bronchospasm.26 Moderate exercise training protocols for patients with stable asthma but dyspnea during ADLs also conferred benefits, including on exercise tolerance.13
The often-reported decreased resting oxygen (O2) consumption and carbon dioxide (CO2) production may account for a significant decrease in dyspnea, increase in functional performance, and an improved sense of well-being. Cardiopulmonary exercise testing is therefore useful to establish baseline exercise ventilation requirements, O2 consumption, and cardiac fitness (oxygen pulse, or O2 consumed per heart beat) and for detecting exercise-induced bronchoconstriction.27 The measures of cardiovascular fitness such as VO2,max and anaerobic threshold, all significantly lower in asthmatic patients, can improve with training.28,29
Chronic obstructive pulmonary disease (COPD) is the second most common noninfectious disease in the world, leading to 2.75 million deaths annually.30–32 More than 120,000 people die from COPD per year in the United States alone, with COPD ranking third in the United States for causes of death.33,34 Sixty-seven sources from 28 countries revealed a prevalence of 7.6% that is projected to increase.33 COPD patients can experience a 45- to 75-mL decrease in FEV1 per year, a rate up to three times normal.35 These patients have frequent hospitalizations and numerous doctor’s visits and need ongoing attention.31
Unlike asthma, COPD airflow limitation is irreversible,36 but pulmonary function impairment does not predict functional impairment. However, clinical exercise testing can confirm the diagnosis, measure the functional reserve of all mechanisms taking part in O2 and CO2 transport, and yield information regarding the capacity to perform exercise, the factors that limit exercise, and the reasons for exercise-related symptoms37,38 (see Fig. 50–1).
Pulmonary function is characterized by air trapping, normal or increased lung compliance, low-maximum midexpiratory flow rates and increased midexpiratory times, and increased flow work. As a result, residual volume and total lung capacity are generally increased. Once the diagnosis is established by history, symptoms, possible alpha1-antitrypsin deficiency, smoking, pulmonary function testing, and chest imaging and other conditions such as heart disease and exercise-induced bronchospasm are ruled out,39,40 pulmonary rehabilitation (PR) is indicated. As for asthma, control of symptoms, decreasing exacerbations, and improving function and quality of life are the clinical goals.41
Early medical attention is important during intercurrent chest infections, which are common complications of COPD.42 Antibiotics and adjustment of glucocorticoids, bronchodilator prescriptions, and mucolytic agents may be indicated. N-Acetylcysteine at 1,200 mg/day was demonstrated in a randomized, double-blind, placebo-controlled study to normalize C-reactive protein levels, improve lung function, and relieve symptoms during acute exacerbations and possibly to prevent exacerbations43 (Fig. 50–2).
Figure 50–2
Stepwise pharmacologic therapy for asthma and COPD. (A) Reproduced with permission from Chronic Asthma and COPD. In: Kumar N, Law A, Choudhry NK, eds. Teaching Rounds: A Visual Aid to Teaching Internal Medicine Pearls on the Wards, New York, NY: McGraw-Hill. (B) Adapted from GOLD guidelines: Global strategy for the diagnosis, management, and prevention of COPD: Revised 2014. Global initiative for Chronic obstructive lung disease (GOLD). http://www.goldcopd.org (Accessed on June 4, 2014). (C) Data from GOLD guidelines: Global strategy for the diagnosis, management, and prevention of COPD: Revised 2014. Global initiative for Chronic obstructivelung disease (GOLD). http://www.goldcopd.org (Accessed on June 4, 2014.

Over 4,000 lung transplantations were performed in 2013 alone for COPD, restrictive lung diseases, alpha1-antitrypsin disease, cystic fibrosis, and idiopathic pulmonary hypertension.44 Median survival of bilateral lung transplantation is slightly better than single transplantation at 7 and 4.7 years, respectively, but worse with primary graft failure45 (Fig. 50–3). Potentially fatal complications in the first posttransplant year include graft dysfunction and infections. After that, chronic graft dysfunction due to bronchiolitis obliterans syndrome is the leading cause of death.45–47 Candidates must have a greater than 50% risk of death from lung disease within 2 years and a greater than 80% chance of surviving 5 years posttransplant considering comorbidities.48 Contraindications include malignancies, persistent infections, smoking, body mass index ≥ 35 kg/m2, history of medical noncompliance, and poor candidature for PR.48
Figure 50–3
Survival rate after lung transplantation as function of primary graft failure at the University of Minnesota. (Reproduced with permission from Nason KS, Maddaus MA, Luketich JD. Chest Wall, Lung, Mediastinum, and Pleura. In: Brunicardi F, Andersen DK, Billiar TR, Dunn DL, Hunter JG, Matthews JB, Pollock RE, eds. Schwartz’s Principles of Surgery, 10e New York, NY: McGraw-Hill; 2015.)

Even prior to transplant, patients may have skeletal muscle impairments including muscle weakness and atrophy with decreased type 1 muscle fibers leading to impaired exercise capacity. This can be addressed by a pretransplant exercise program including education, muscle strengthening, and endurance exercises.49 Posttransplantation, while immunosuppressive therapy addresses organ rejection,50,51 rehabilitation focuses on symptoms, exercise tolerance, and quality of life.49
Pneumonia is the eighth leading cause of death in the United States.52–59 For patients requiring critical care for pneumonia, daily physical therapy along with optimal attention to nutrition is needed to avert critical care neuromyopathy (deconditioning),60 a condition principally caused by prolonged bedrest, poor nutrition, sepsis, and intravenous glucocorticoid administration.61–65 Prospective studies show that this occurs in up to one-third of COPD and status asthmaticus patients and 7% of lung transplant patients.61,66,67 Creatine kinase levels are often elevated,68 and vital capacity (VC) is greatly diminished. The VC can be simply measured by placing an intubated patient on “CPAP” mode without pressure support and having him or her exhale a deepest possible breath into the ventilator, with the tube cuff inflated, to read the expiratory volume.
When ventilatory failure persists, such that when intubated patients fail spontaneous breathing trials and ventilator weaning parameters, intensivists often mistakenly think that they need tracheotomy tubes to permit safe extubation.60,69,70 However, most cooperative patients can be extubated to continuous noninvasive ventilatory support (CNVS) and have airway secretions expulsed by mechanical insufflation-exsufflation (MIE) without recourse to tracheotomy. They need not be ventilator weaned to be extubated but can wean after extubation from CNVS with better nutrition and physical therapy.71,72
Both obesity and unexplained weight loss, which seems to be linked to skeletal muscle atrophy and dysfunction, are common in chronic lung disease. Indeed, depression has been reported in 50% of COPD patients and can lead to both obesity and undernutrition. Psychotherapy and antidepressant medications can improve quality of life, cooperation with rehabilitation,73,74 and help resolve disorders of nutrition.
Negative energy balance, which is due to elevated energy requirements and/or reduced dietary intake, is the cause of weight loss and fat wasting.75 In one study, between 20 and 70% of patients were underweight.76 In another, body weight <90% of ideal (IBW) was seen in 25 to 43% of COPD patients. The muscle atrophy has a direct negative impact on exercise capacity. Undernutrition can also lead to impaired immunity and increased colonization and adherence of bacteria in the upper and lower airways, thus increasing susceptibility to infection77–79 and hampering lung repair, surfactant synthesis, water homeostasis, control of ventilation, and response to hypoxia.80 Other long-term sequelae can include cor pulmonale, increased rate of hospitalization, and hypercapnic respiratory failure.81–83 Thus nutritional status must be optimized.84
A 6-month intervention consisting of dietary counseling and food fortification resulted in significant weight and fat mass gain and maintenance of lean body mass by comparison with a control group.85 Patients are advised to take small mouthfuls, eat slowly, and take smaller, more frequent meals to prevent bloating due to a low diaphragm. They are taught not to swallow during inhalation. If O2 desaturation occurs while eating, supplemental O2 is used or increased, but if desaturation is due to aspiration, MIE may be required to clear the aspirate if cough flows are poor. For those with hypercapnia, a dietary regimen high in calories derived from fat can decrease hypercapnia. Although short-term refeeding can be beneficial, refeeding programs lasting more than 2 weeks have not consistently resulted in increased body weight. Growth hormone has not been shown to be useful. Anabolic steroids as adjuncts to nutritional support and exercise have been reported to increase lean body mass and promote weight gain.79,86,87 Multimodal interventions such as integrating nutrition and anabolic steroids into PR improve body weight, fat-free mass, exercise tolerance, and even survival for compliant individuals.88 A 4-month intervention consisting of exercise and standardized nutritional supplements followed by a 20-month maintenance program for mild COPD patients was equally beneficial and suggests that early intervention should be considered.89
Smoking cessation is facilitated, O2 therapy optimized, vaccinations provided, and optimal use of inhalers is taught. This includes the proper use of “spacers” and nebulizers90 along with administration of beta-agonists and anticholinergics. Orally administered beta-agonists and inhaled glucocorticoids can be added to a patient’s regimen with instructions on how to limit tongue deposition of the medications. Patients need to be cautioned to follow the prescribed regimen, that is, to avoid overutilization when ill and underutilization when feeling better. Thirty-three double-blind, randomized, placebo-controlled studies revealed that bronchodilator therapy can improve exercise capacity.91
Anxious patients commonly exhibit shallow, rapid breathing that leads to an increase in dead-space ventilation, airflow through narrowed airways, and increased work of breathing. An altered pattern of ventilatory muscle recruitment also occurs with chronic airflow obstruction. Breathing retraining including diaphragmatic and pursed-lip breathing decreases respiratory rate primarily by prolonging expiration88 (Fig. 50–4).
Figure 50–4
Change in facial appearance during the development and resolution of acute respiratory failure resulting from congestive heart failure and exacerbation of COPD. Top left: The patient is dyspneic and her mouth is open on inhalation. Top right: The patient exhibits pursed-lip breathing on exhalation. Over the ensuing 24 hours, the patient developed hypercapnic and respiratory failure and failed a trial of noninvasive ventilation (not shown). Bottom left: The patient is intubated and receiving mechanical ventilation. Bottom right: The patient is successfully extubated 4 days after institution of mechanical ventilation. (Reproduced with permission from Laghi F, Tobin MJ. Chapter 4. Indications for Mechanical Ventilation. In: Tobin MJ, eds. Principles and Practice of Mechanical Ventilation, 3e New York, NY: McGraw-Hill; 2013.)

Diaphragmatic breathing has been reported to improve blood gases and, along with breathing via pursed lips, decreases dyspnea and improves walking distance as well as gas exchange.92 For diaphragmatic breathing, one hand is placed over the abdomen and the other on the thorax just below the clavicle, while breathing deeply through the nose. The abdomen is distended forward, while movement of the rib cage is kept to a minimum. During exhalation through pursed lips, the patient gently presses on the belly, thus pushing the diaphragm up to facilitate expiration.93 This equalizes pleural and bronchial pressures, decreases collapse of smaller bronchi, and decreases air trapping. It is used during routine ADLs, and it improves exercise performance by relaxing accessory muscles and improving breathing efficiency. Persons with moderate-to-severe COPD and marked lung hyperinflation with minimal diaphragm movement may be poor candidates.94
Air-shifting techniques can be used several times per hour to decrease microatelectasis. A deep inspiration is held with the glottis closed for 5 seconds, during which time the air shifts to less-ventilated lung regions. Expiration is via pursed lips. Computer-aided breathing feedback assists in reducing dynamic hyperinflation of asthma and COPD, thereby improving quality of life. It can be used along with yoga, Buteyko breathing, and standard breathing retraining.20,88
Trapping of airway secretions is principally responsible for exacerbations of COPD. Such patients may also have frequent bouts of ineffective coughing. While coughing can generate pressures that create flows to expulse secretions, this can also exacerbate air trapping. The primary site of secretion accumulation must be determined because clearance techniques can target peripheral or central airways as well as specific lung regions.95 When coughing is ineffective, following a deep breath rapid, short, expulsive bursts of air without glottis closure (“huffing”) can be an effective and comfortable alternative to coughing. Chest percussion and postural drainage target secretion accumulation in the peripheral airways. There are nine body positions that facilitate drainage of all lung areas. Chest percussion, vibration, and/or oscillation is ideally used in conjunction with postural drainage.95 Autogenic drainage involves breathing using small tidal volumes below functional residual capacity to mobilize secretions in small airways. Then the patient takes increasingly larger tidal volumes and huffs to transport mucus to the mouth.96
Positive expiratory pressure (PEP) and oscillatory PEP therapy (OPEP) are also used to facilitate airway clearance. It is theorized that PEP stents open the airways to prevent collapse, increasing intrathoracic pressure distal to retained secretions via collateral ventilation, or by increasing functional residual capacity. OPEP decreases the viscoelasticity of mucus and creates short bursts of increased expiratory airflow to mobilize mucus up the airways. Studies of clinical benefits of PEP and OPEP for cystic fibrosis and COPD have been inconclusive.97
The effects of mechanical chest percussion and vibration appear to be frequency dependent.98–100 In most animal studies, frequencies between 10 and 15 Hz appear to best facilitate mucous transport.98,100,101 Side effects of percussion and vibration can include increasing obstruction to airflow102,103 and atelectasis, as seen in an animal model.104 Some studies show decreased hospitalization rates in patients using the THAIRapy Vest (Hill-ROM Respiratory Care, Minneapolis, MN) on a daily basis, and it has become popular among cystic fibrosis (CF) and familial dysautonomia patients despite inconsistent outcome studies.105
The Percussionator provides percussive high-flow minibursts of air at rates of 2.5 to 5 Hz while also delivering aerosolized medications. In the treatment of COPD patients with postoperative atelectasis and secretion mobilization, studies support intrapulmonary percussive ventilation as better than chest percussion and postural drainage.106,107
Clearing secretions from the central airways requires effective flows. These can be provided by MIE, which has been documented to be extremely effective for patients with severe respiratory muscle dysfunction. A recent study demonstrated that it can also be important for preventing postextubation respiratory failure for debilitated COPD and other lung disease patients as well.108
Relatively minor changes in breathing patterns or respiratory muscle loading can trigger acute respiratory muscle fatigue and respiratory failure. Hypercapnia is an indication of limited reserve before overt fatigue and can indicate the need for periods of respiratory muscle assistance or rest before considering strengthening exercises.109 Despite high ventilation rates in COPD, there is reduced ventilatory response to hypercapnia and hypoxia that is exacerbated during sleep. Although long-term O2 administration can improve COPD survival, it can exacerbate CO2 retention and acidosis when used alone. Pulmonary vascular resistance, a result of pulmonary tissue hypoxia, can be exacerbated by acidosis and may lead to right ventricular failure when severe. Interspersing periods of exercise and muscle rest to prevent fatigue is a basic principle of rehabilitation.
Diaphragm rest can be achieved by nasal ventilation usually delivered by bilevel machines.110 Noninvasive intermittent positive-pressure ventilation and high spans of bilevel positive airway pressure unload respiratory muscles to reduce work of breathing and can provide up to full noninvasive ventilatory support (NVS) and even be used to decrease dyspnea during exercise. Reducing dyspnea, improving gas exchange, increasing minute ventilation, and greater exercise tolerance are possible benefits of NVS, making it a useful adjunct to PR.88 Some patients with primarily lung/airways diseases require continuous ventilatory support and can use continuous NVS rather than undergo tracheotomy (Fig. 50–5).
The majority of hypercapnic lung disease patients, however, benefit sufficiently from nocturnal bilevel positive airway pressure (PAP) rather than CNVS (Fig. 50–6).
Nocturnal respiratory muscle rest can normalize arterial blood gases; improve quality of life, 12-minute walking distance, and respiratory muscle endurance; and decrease dyspnea,111 as well as splint open the airway to prevent sleep apneas and airway collapse112 (Fig. 50–7).
Figure 50–7
Continuous positive airway pressure (CPAP) can splint open the airway to prevent sleep apneas and airway collapse. (Reproduced with permission from Espiritu JD, Matuschak GM. Management of Sleep-Related Breathing Disorders. In: Lechner AJ, Matuschak GM, Brink DS, eds. Respiratory: An Integrated Approach to Disease, New York, NY: McGraw-Hill; 2012.)

Although nasal and oronasal NVS has been used to avert intubation and tracheostomy for COPD patients in acute exacerbation, nasal bilevel PAP has the additional benefit of countering auto–positive end-expiratory pressure (auto-PEEP) in these patients who trap air to decrease the work of breathing. Nocturnal nasal bilevel PAP was also reported to improve sleep efficiency and total sleep time for hypercapnic COPD patients.113
Dyspnea during even high-intensity exercise has been reported to be reduced by using proportional assist ventilation or NVS for severe COPD.114–117 Those who benefit from proportional assist ventilation achieved 15% higher exercise levels at 6 weeks than those exercising without it. At similar workloads, patients had significantly reduced concentrations of plasma lactate after training.
Incentive spirometers encourage deep breathing to decrease atelectasis. Inspiratory resistive exercises such as inspiratory threshold loading, inspiratory resistive loading, and maximum sustained ventilation can improve respiratory muscle strength and endurance for lung disease patients.118–121 Adjustable devices are set at increasing levels of difficulty as the patient improves.122,123 Resistive loading requires the patient to breathe through a variable-diameter orifice; the smaller the orifice, the greater is the resistance. When executed correctly and consistently, resistive loading improves muscle function, but patients must be monitored during this exercise because it is hard for them to maintain adequate intensity. As patients become fatigued, they reduce their flow rates and lengthen their inspiratory time to decrease the load severity.118 With threshold loading, patients must achieve a predetermined pressure to generate flow through the device. Although resistive and threshold loading can be effective, threshold loading does not require monitoring.124
A meta-analysis of 32 randomized, controlled trials in COPD suggests that exercise capacity, inspiratory muscle strength/endurance, dyspnea, and quality of life are improved by respiratory muscle training.125 Its benefits may not be limited to COPD patients because older unaffected patients have also been reported to have improvements in HRQL, dyspnea, and maximal inspiratory pressures using it.126 A study by Petrovic et al suggested that it also has a beneficial effect on dynamic hyperinflation, which is thought to be the principal cause of exertional dyspnea in COPD.127,128 Although protocols often involve both inspiratory and expiratory muscle training, inspiratory muscle training is better studied and has been shown to be slightly more effective than expiratory muscle training for decreasing dyspnea.124
Long-term oxygen therapy (LTOT) improves mortality and quality of life in COPD patients with severe resting hypoxemia.129,130 In addition, O2 therapy can decrease heart rate and blood pressure.131 Indications for LTOT are a PaO2 ≤ 55 mm Hg or SpO2 ≤ 88%, cor pulmonale, and erythrocytosis with a PaO2 ≤ 59 mm Hg or SpO2 ≤89%. Overnight oxygenation is indicated in patients whose PaO2 decreases to 55 mm Hg during sleep or by more than 10 mm Hg compared with daytime O2 levels.132
Another consideration for supplemental O2 therapy is for exercise hypoxemia, especially when PaO2 decreases below 55 mm Hg or SpO2 below 88% during exercise. Short-term O2 therapy may also be indicated for patients who have dyspnea without decreases in O2 levels as long as it increases exercise tolerance.133,134 Ambulatory O2 therapy can significantly improve walking distance when compared with controls.135 A recent study found significant improvements in endurance without gains in strength for nonhypoxemic COPD patients during high-intensity exercise training.136
Reconditioning exercise has been shown to prolong survival for typically sedentary COPD patients independent of LTOT.137 Besides increasing exercise tolerance, 6-minute walk distance, and functional activity scores,138 improvements in exercise tolerance also predict increased survival better than PaO2, PaCO2, FEV1, and nutrition status.139 Ongoing rehabilitation is important because improvements often decline after cessation.140
COPD patients have a high ventilation requirement and a rapid increase in respiratory rate during exercise due to inefficiency of gas exchange. This can cause hypoxia and hypercapnia.141 It is sometimes difficult for patients to reach 60 to 70% of predicted maximum heart rate and the minute O2 consumption needed for aerobic exercise training.142 Their reduction in heart rate variability is also associated with greater morbidity and mortality.143,144 However, the greater exercise capacity achieved by high-intensity programs145–147 can improve heart rate variability at rest and orthopnea for COPD patients.148 Thus, exercise regimens should be maximally intense for durations of 30 to 45 minutes for maximal benefits145,149 (Fig. 50–8). Even severely debilitated patients’ O2 consumption and exercise tolerance can benefit from submaximal therapies such as 6-minute walking150 (Fig. 50–8).
Figure 50–8
Outcomes of maximally intensive exercise reconditioning. Mean values of VO2,max, ventilatory anaerobic threshold (VAT), maximum tolerated power (TMW45), and 12-minute walk before and after maximally intensive square-wave endurance exercise training (SWEET) (●) or O2 cart pushing group (○) (*p < 0.05;**p < 0.01).145 (Used with permission from the American Journal of Physical Medicine and Rehabilitation.)

Reconditioning exercises can include pool activities, calisthenics, bicycling, and stair climbing.151 One study compared endurance and strength training from calisthenics and breathing exercise training and found significant improvements in quality of life and functional status for both groups, but only the endurance and strength-training group showed significant improvements in exercise capacity and muscle force.152 Moreover, quadriceps weakness is prevalent among COPD patients, often even in the absence of breathlessness or severe airflow obstruction.153 To address this, use of a stationary bicycle can be especially beneficial and relatively inexpensive for home use. Some programs include 12-minute walking and 15 minutes of inspiratory resistance training daily and have patients keep daily logs to encourage progress. The logs can also be reviewed weekly for the exercise parameters to be modified as warranted by improvement. Supervised peer group sessions are useful to encourage compliance.154
Arm and shoulder muscles are very active accessory respiratory muscles in lung disease patients. Patients are often dyspneic when performing upper limb ADLs. Thus, comprehensive PR programs include upper limb reconditioning.155–160
Unsupported arm exercises decrease O2 consumption during exercise more than supported arm exercises (i.e., arm cycle ergometer).157–161 Unsupported upper limb exercises include eating, grooming, reaching, carrying, and typing. These increase diaphragm work more and lead to earlier fatigue.157 When comparing PR with and without upper limb exercises, the former group showed significantly greater improvements in functional exercise capacity.162 However, one study found that upper limb exercises with diagonal movements similar to ADLs caused more dynamic hyperinflation than lower limb exercises at the same metabolic demand.163
Evidence-based support for PR of respiratory patients continues to accumulate.164 A recent retrospective analysis of 229 COPD patients who underwent PR indicated significant improvements in dyspnea, quality of life, and functional capacity regardless of baseline disease burden.165 In other reviews of over 50 PR exercise reconditioning studies, it was concluded that exercise tolerance improved significantly in all, including patients with mild or advanced (hypercapnic) disease.166–168 Also, PR has consistently been reported to decrease ventilatory equivalent and the ventilation/O2 consumption ratio, increase work efficiency (external work per unit of O2 consumed), increase exercise tolerance, reduce dyspnea, and improve ambulation capacity and HRQL. Patients develop better performance strategies and have greater confidence. Decreases in blood lactate levels can be observed along with higher VO2,max, implying a physiologic training effect (Fig. 50–9).
Figure 50–9
Results of maximally intensive endurance exercise training. Comparison of dyspnea, arterial lactate, and respiratory equivalent of O2 (VE/VO2) before and after training in the maximally intensive square-wave endurance exercise training (SWEET) group (●) and the O2 cart pushing group (○) at 40 watts of exercise (*p < 0.05;*** p < 0.001).145 (Reprinted from Gimenez M, Servera E, Vergara P, Bach JR, Polu JM. Endurance training in patients with chronic obstructive pulmonary disease: a comparison of high versus moderate intensity. Arch Phys Med Rehabil. 2000;81:102–109.)

Besides tolerating greater exercise intensity, 20 rather than 10 sessions of comprehensive PR also yielded better outcomes for multiple parameters. Short-term, intensive programs (20 sessions condensed into 3–4 weeks) were also effective.164 Improvements in quality-of-life measures,166,169–171 hospitalization rates, postoperative pulmonary complications,172 and physical functioning are seen in both inpatient and outpatient programs. Specifically, following acute exacerbations of COPD, maximally intensive PR programs resulted in decreased hospital admissions according to a Cochrane Review of randomized trials,173 even though pulmonary function parameters, such as FEV1, did not significantly improve in 31 of 35 of the studies.166 Some studies suggest that there is a decline in PR benefits toward baseline after 6 to 12 months of cessation, but subjects remained improved compared with controls after 1 year.164
In summary, a Cochrane meta-analysis of PR in COPD174 concluded that “rehabilitation relieves dyspnea and fatigue, improves emotional function, and enhances patients’ control over their condition. These improvements are moderately large and clinically significant. Rehabilitation forms an important component of the management of COPD.”
Patients with neuromuscular disorders (NMDs), spinal cord injury (SCI), obesity hypoventilation syndrome (OHS), and severe restrictive chest wall disorders such as from kyphoscoliosis develop ventilatory pump failure. Typically, they have both inspiratory and expiratory (cough) muscle weakness and may have bulbar-innervated muscle (BIM) impairment as well. They are either untreated until they present in cor pulmonale from hypercapnic ventilatory failure, or they develop acute respiratory failure (ARF) principally due to an inability to clear their airways because they are unable to generate effective cough flows. They may also develop ARF from aspiration pneumonia due to BIM impairment. At that point, they present to emergency services and are treated with supplemental O2. This diminishes their hypoxic ventilatory drive and can drive up their PaCO2 by over 100 mm Hg in only a few hours.175 As a result, they become obtunded and are intubated. When they fail ventilator weaning parameters and spontaneous breathing trials, they are invariably informed that tracheotomy is their only option for survival because there is little general experience in how to extubate and decannulate continuously ventilator–dependent individuals to CNVS and use MIE to expulse their airway secretions.
Should the patient present with symptomatic hypoventilation before developing ARF or being treated with supplemental O2, he or she is then typically sent for polysomnography and treated like patients with “sleep-disordered breathing,” which is some combination of central and obstructive sleep apneas and hypopneas. Unfortunately, polysomnograms were never programmed to interpret apneas and hypopneas due to respiratory muscle weakness, overwork, or dysfunction due to central or obstructive events.
“Sleep doctors” then invariably attempt to titrate away the apneas and hypopneas by using continuous positive airway pressure (CPAP) or low spans of bilevel PAP. The CPAP is useless when the problem is respiratory muscle weakness, and the low-span bilevel PAP minimally rests dysfunctional muscles and is inadequate for the full ventilatory support that they may eventually require. In addition, physicians often add supplemental O2, which renders any possible noninvasive ventilatory support (NVS) from higher bilevel spans ineffective176 and makes oximetry useless as a gauge of alveolar ventilation, airway secretion congestion, and lung disease.177 Often the titrations are made by increasing both the inspiratory (I) and expiratory (E) PAPs to unbearable levels, such as the IPAP 23 cm H2O and EPAP 19 cm H2O that one of our patients presented using. We solved his problem by switching him to pressure preset assist/control ventilation of 23 cm H2O with no EPAP or PEEP.
Physical medicine respiratory muscle aids are manual methods and devices that apply positive and negative pressures to the airways or body to enhance inspiratory and expiratory muscle function. They include the use of intermittent positive-pressure ventilation via noninvasive interfaces for NVS and MIE. They can prolong survival for patients with little or no VC or any ability to cough and can permit extubation and tracheostomy tube decannulation of ventilator-unweanable patients with ventilatory pump failure.71,72,178
The noninvasive interfaces for ventilatory support include simple 15-mm angled mouthpieces, suction catheter tubing, scuba mouth pieces, and so on. Ventilatory support by mouthpiece was first described in 1953 to get poliomyelitis patients out of iron lungs. It has been used in a regimen of CNVS by many hundreds of patients.179 The Philips-Respironics Company created a mouthpiece NVS ventilatory support mode for their Trilogy ventilator in 2013.
In 1964, the Bennett Lipseal became available. The Lipseal fixed a mouthpiece into the user’s mouth and sealed the lips for effective NVS during sleep. In 1987, nasal NVS was first described as an alternative to Lipseal NVS for sleep for patients requiring CNVS.180 Patients who leak too much of the NVS out of their noses or mouths during sleep can be switched to using oronasal interfaces that can provide a closed system of NVS in like manner to tracheostomy mechanical ventilation (TMV) with an inflated tracheostomy tube cuff.
In a recent study of 55 patients with typical to severe SMA type 1 who began using NVS for sleep at 0.4 ± 0.5 years of age, 27 became CNVS dependent with little or no ventilator-free breathing ability for 12.2 ± 3.5 years to a current age of 14.1 ± 3.4 years of age (Fig. 50–10). We now have eight SMA type 1 patients over twenty years of age dependent on CNVS since infancy without tracheostomy tubes. Seven became CNVS dependent without being hospitalized, and fifteen are still CNVS dependent; seven died; four underwent tracheotomy, although three of the four were in other states and not referred back to us, at 4.8 ± 3.7 years of age. Others have also reported CNVS dependence for patients with SMA type 1181 (Fig. 50–10).
Of 116 of our patients with DMD who began sleep nasal NVS at 20.3 ± 2.8 years of age and used it for less than CNVS for 2 ± 2.1 years, 114 became CNVS dependent at 22.5 ± 5.9 years of age for a mean 11.0 ± 5.9 years to a mean 33.6 ± 6.1 years of age thus far; 38 became CNVS dependent without developing ARF or being hospitalized; 95 continue to use CNVS, 1 underwent tracheotomy, and 21 died from cardiac/sudden and apparently nonrespiratory causes. Eight of our DMD patients have now lived over forty years, up to 54, using CNVS for 22 to 29 years. Twenty-one other centers have also reported prolongation of life for DMD by CNVS.181 In one of them, 21 consecutive DMD patients whose ventilatory insufficiency was managed by tracheostomy died at a mean 28.1 years of age as opposed to 88 consecutive CNVS users for whom 50% survival was to age 39.6 without tracheostomy tubes. None of over 250 CNVS-dependent patients with DMD in a multicenter study has undergone tracheotomy.181
Likewise, of 246 amyotrophic lateral sclerosis (ALS) patients, 115 went on to become CNVS dependent for 1.2 ± 3.4 (0.1–10.2) years before their O2 saturation baseline decreased and persistently remained below 95% due to upper motor neuron upper airway collapse and they required tracheotomy for further survival. Another center reported that 25% of ALS patients survived for almost 1 year using NVS before needing a tracheotomy or dying.181 Thus, it is not BIM impairment that necessitates tracheotomy but upper motor neuron/central nervous system BIM impairment that causes hypertonicity and collapse of the upper airways (stridor).
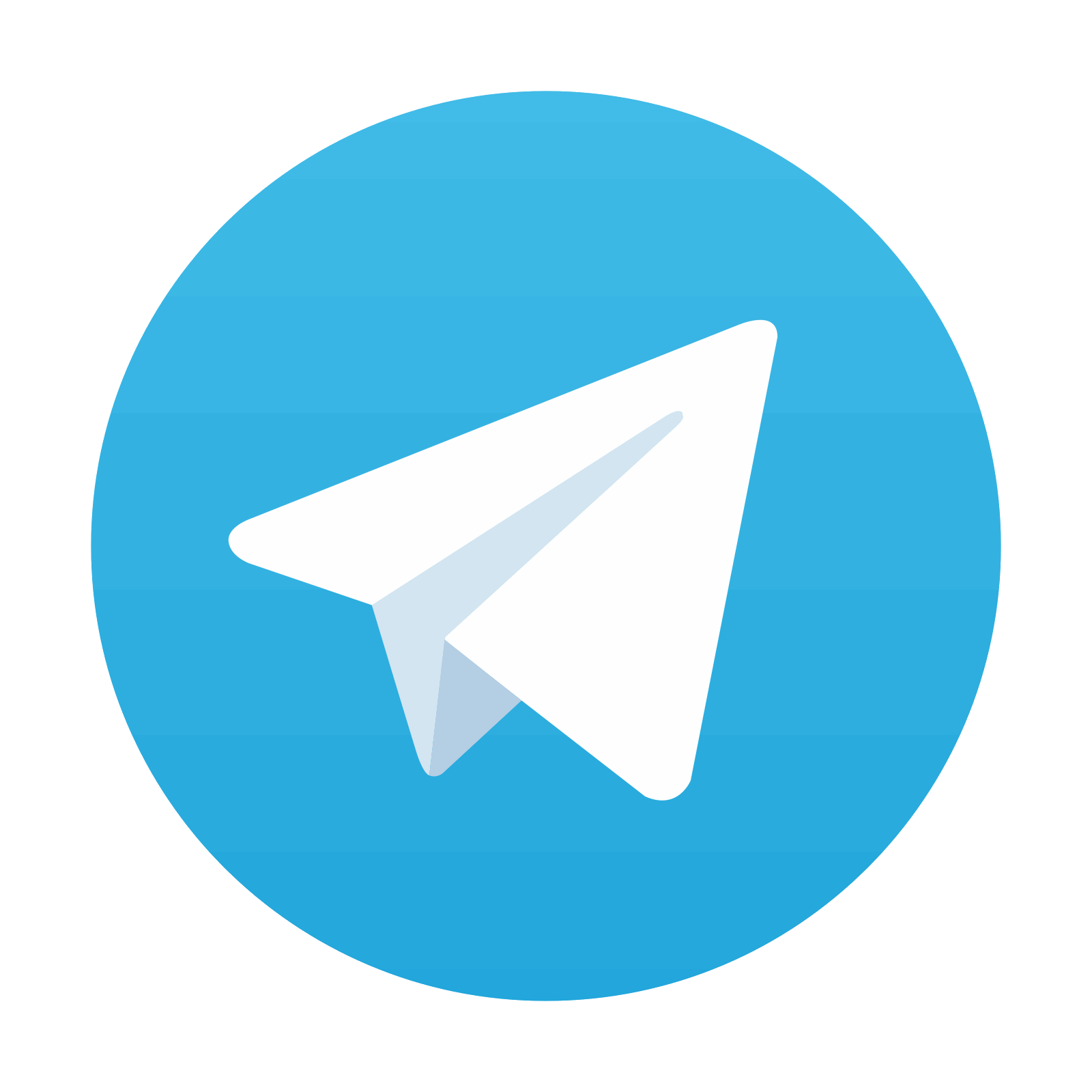
Stay updated, free articles. Join our Telegram channel

Full access? Get Clinical Tree
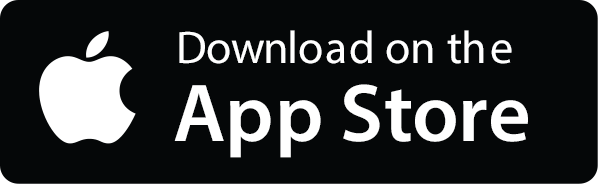
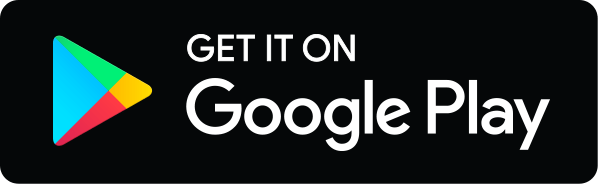