This chapter is designed to give the rehabilitation specialist an overview of commonly prescribed medications in the rehabilitative setting. It is not intended to be a complete compendium of drugs but reviews a select few commonly seen in practice. It begins with an overview of pharmacokinetics and pharmacodynamics so that the reader can apply these principles for a better understanding of how drugs affect physiology and function.
Optimum drug response depends on a complex array of pharmacokinetic and pharmacodynamic parameters, as well as external influences such as patient compliance, formulations, dose and dosing schedules, physical activity, and even ambient temperature. Pharmacokinetics (PK) describes what happens to a drug as it enters the body and encompasses the phases of absorption, distribution, metabolism, and excretion.1 Generally, this is the process by which an active agent is absorbed into the systemic circulation, travels around the body either as free drug or bound to plasma proteins, and activates a target organ or receptor, before being metabolized into an inactive compound and excreted. Pharmacodynamics refers to what the drug does to the body, mechanism of action, and drug–receptor interactions (Fig. 45–1).
Figure 45–1
The relationship between dose and effect can be separated into pharmacokinetic (dose concentration) and pharmacodynamic (concentration effect) components. Concentration provides the link between pharmacokinetics and pharmacodynamics and is the focus of the target concentration approach to rational dosing. The three primary processes of pharmacokinetics are input, distribution, and elimination. (Reproduced with permission from Holford NG. Pharmacokinetics & Pharmacodynamics: Rational Dosing & the Time Course of Drug Action. In: Katzung BG, eds. Basic & Clinical Pharmacology, 14e New York, NY: McGraw-Hill; 2018.)

The amount of drug necessary to reach a therapeutic level in the blood depends on a delicate balance between the amount entering the body (dose) and the amount excreted.2 Drug absorption refers to the process by which a drug reaches the systemic circulation from its site of administration. Drugs can enter the body in a variety of ways; orally, via enteral administration through the gastrointestinal (GI) tract, and parenterally, referring to topical, transdermal, subcutaneous, and intravenous mode.3 Other routes of administration include sublingual, rectal, inhalation, intramuscular, and via implantable reservoirs. The mode chosen depends on the physiochemical properties, for example, molecular weight, solubility, and degree of ionization.
The most convenient administration is oral, in which a tablet, capsule, or liquid is swallowed and then enters the GI tract. However, this mode of administration is very inefficient. Usually the drug is absorbed through the GI tract and then enters the hepatic circulation, where some drug is lost as it undergoes metabolism before moving on to the systemic circulation. This is known as the “first-pass effect”4 (Fig. 45–2).
Figure 45–2
The principles of organ extraction and first-pass effect are illustrated. Part of the administered oral dose (blue) is lost in the gut in the feces or to metabolism and lost to metabolism in the liver before it enters the systemic circulation: this is the first-pass effect. The extraction of drug from the circulation by the liver is equal to blood flow (Q) times the difference between entering and leaving drug concentration: Q × (Ci – Co). CL, clearance. (Reprinted from Katzung BG, ed. Basic & clinical pharmacology, 11th ed. New York, NY: McGraw-Hill; 2001.)

Additionally, if the molecule is large, much of it may pass through the GI tract and be excreted in feces. The amount of drug that is absorbed is also dependent on the amount that may be destroyed by gastric secretions, the presence of food, rate of gastric emptying, and the pH. Since most drugs are either weak acids or weak bases, they carry a charge depending on the local pH. Drugs that are acidic become nonionized in the stomach but ionized in the basic environment of the small intestines.5,6 Because nonionized drugs are more readily absorbed through the GI tract, acidic drugs will be absorbed through the stomach and basic drugs will be absorbed through the small intestines. The caveat to this, however, is that the intestine offers a tremendous surface area for absorption compared to the stomach so some of the acidic drug may be absorbed in this area. Therefore, aspirin which might irritate the mucosal lining of the stomach can be formulated with an enteric coating preventing absorption in that location, while still being absorbed through the intestine.
The amount of drug that enters the systemic circulation is referred to as “bioavailability”4 (Fig. 45–3). Bioavailability varies from 1% for the oral daily formulation of alendronate (Fosamax), a bone building compound, to 100% for drugs given intravenously (IV). Drugs given via the enteral route tend to have reduced bioavailability due to the “first-pass effect” and poor absorption through the GI mucosa.
Figure 45–3
The plasma concentration plotted against the time and the area under the curve (AUC) is an indication of bioavailability. In this graph, an orally administered drug is compared with the same drug administered intravenously. (Reproduced with permission from Absorption, Distribution, and Clearance. In: Stringer JL, eds. Basic Concepts in Pharmacology: What You Need to Know for Each Drug Class, 5e New York, NY: McGraw-Hill; 2017.)

Table 45–1 lists factors affecting absorption of drugs given orally. Drugs given through inhalation or sublingually have the potential for greater bioavailability than those given orally. Nitroglycerin is the prototypic drug that is given sublingually. The tablet is placed under the tongue, where the drug is absorbed through the mucous membranes and enters a venous plexus that drains into the superior vena cava.2 This method of administration bypasses “first-pass effect” and results in relief of angina in 1 to 2 minutes. However, there is a possibility that some of the drug is swallowed. Rectal administration is similar, but absorption from this area tends to be inconsistent. Beta-2 inhalers, such as albuterol, used to improve bronchodilation during an asthma attack, work rapidly by inhalation, entering the pulmonary system through the thin alveolar walls and delivering drug directly to the area of disease. Drugs administered subcutaneously and intramuscularly slowly diffuse away from the site of administration, relying on a good blood supply. The rate of absorption can be controlled by altering the molecular weight, producing a differential duration of action, as with the case of short-acting and intermediate-acting insulins.
Drug characteristics | Dosage form Local concentration of drug Polarity Water/lipid sloubility Degree of ionization pKa |
Intestinal factors | Surface area for absorption Local blood flow Mottility Transit time Presence of food Presence of other agents |
Gastric factors | Rate of dissolution Gastric pH Gastric enzyme activity |
Following absorption and initial pass through the liver, the drug enters the systemic circulation and is distributed throughout the body following the vasculature. Much of the drug enters organs that are well perfused first. The drug may be distributed as free drug or bound to plasma proteins such as albumin. Only free drug is available to work at the target organ. Drugs with high protein binding may produce adverse drug reactions (ADRs). The anticoagulant warfarin likes to bind to protein. If given along with another highly bound drug, warfarin may be displaced, causing higher-than-expected plasma concentrations leading to excessive bleeding (Fig. 45–4).
Figure 45–4
Effect of drug binding on volume of distribution. Drug A diffuses freely between the two compartments and does not bind to macromolecules (heavy wavy lines) in the vascular or the extravascular compartments of the hypothetical organism in the diagram. With 20 units of the drug in the body, the steady-state distribution leaves a blood concentration of 2 units. Drug B, on the other hand, binds avidly to proteins in the blood. At equilibrium, only 2 units of the total are present in the extravascular volume, leaving 18 units still in the blood. In each case, the total amount of drug in the body is the same (20 units), but the apparent volumes of distribution are very different. Drug C is avidly bound to molecules in peripheral tissues so that a larger total dose (200 units) is required to achieve measurable plasma concentrations. At equilibrium, 198 units are found in the peripheral tissues and only 2 units in the plasma, so that the calculated volume of distribution is greater than the physical volume of the system. (Reprinted from Katzung BG, ed. Basic & clinical pharmacology, 11th ed. New York, NY: McGraw-Hill; 2001.)

Drugs diffusing across membranes must be lipid soluble. This is especially true for drugs that need to enter the central nervous system. The blood–brain barrier consists of very tight junctions, and only highly lipid-soluble agents may traverse this barrier. Additionally, drugs that are lipid soluble may enter and be stored in adipose tissue. Drugs stored outside the plasma have a large volume of distribution. Vd relates the total amount of drug in the body to the dose given and mathematically is expressed as7:
Drugs with large Vd are found in the extravascular tissues, whereas drugs like warfarin, with a Vd close to the volume of blood, stay largely confined to the circulation. Drugs with a large Vd take longer to be cleared from the body and require larger loading doses to reach therapeutic levels.
The third phase of pharmacokinetics is metabolism, also known as biotransformation.8 This is the phase that takes an active drug and transforms it into an inactive water-soluble drug that can be filtered through the glomerulus and excreted from the body. Phase I reactions consist of oxidation, hydrolysis, or reduction reactions, performed by a group of enzymes referred to as P450 enzymes. Phase II metabolism includes conjugation reactions that take large polar molecules and attach them to the drugs (Fig. 45–5). Sometimes the compound administered is an inactive prodrug that becomes active with these reactions.9 Metabolism largely occurs in the liver, although some drugs undergo biotransformation in the lungs, small intestine, kidneys, and even skin. Changes in kidney and liver perfusion over time are responsible for greater toxicities in the elderly.
Figure 45–5
Phase I and phase II reactions, and direct elimination, in drug biodisposition. Phase II reactions may also precede phase I reactions. (Reproduced with permission from Correia M. Drug Biotransformation. In: Katzung BG, eds. Basic & Clinical Pharmacology, 14e New York, NY: McGraw-Hill; 2018.)

There are sex, ethnic, and racial differences in how drugs are metabolized resulting from genetic polymorphisms in the P450 enzyme genes.10 Some individuals may be ultra-rapid metabolizers, and others may be poor metabolizers. A consequence of being a slow metabolizer is higher plasma drug levels than expected, leading to toxicity. A related concept is enzyme induction and enzyme inhibition. Some drugs enhance the activity of the enzymes metabolizing other drugs.11 An example is the reaction between birth control pills and antimicrobials. The antimicrobials enhance the activity of the enzymes that metabolize contraceptives, making them less effective.
The primary organ for drug excretion is the kidney. Free drug is filtered through the glomerulus and enters the tubular fluid. If the drug is still lipid soluble, it will be reabsorbed back into the systemic circulation to be distributed again. If it is sufficiently water soluble and ionized, the compound will remain in the tubular fluid and be excreted in the urine.12 Drugs are also eliminated in the feces, saliva, sweat, and breast milk.
Determining clearance of a drug is critical to determining drug dosage. To be effective, the plasma level of the drug must remain at a therapeutic level, meaning that drug elimination must equal the rate of drug administration, which is known as “steady-state” concentration.13 Most drugs follow first-order elimination in which a certain percentage of drug is cleared from the body within a unit of time so that elimination is dependent on its concentration (Fig. 45–6).
Figure 45–6
Plasma concentration–time curves following intravenous administration of a drug (500 mg) to a 70-kg patient. (A) Drug concentrations are measured in plasma at 2-hour intervals following drug administration. The semi-logarithmic plot of plasma concentration (Cp) versus time appears to indicate that the drug is eliminated from a single compartment by a first-order process (Eq. 2-11) with a t1/2 of 4 hours (k = 0.693/t1/2 = 0.173 hr−1). The volume of distribution (V) may be determined from the value of Cp obtained by extrapolation to t = 0 (Cpo = 16 μg/mL). Volume of distribution (Eq. 2-10) for the one-compartment model is 31.3 L, or 0.45 L/kg (V = dose/Cpo). The clearance for this drug is 90 mL/min; for a one-compartment model, CL = kV. (B) Sampling before 2 hours indicates that in fact the drug follows multiexponential kinetics. The terminal disposition t1/2 is 4 hours, clearance is 84 mL/min (Eq. 2-6), Varea is 29 L (Eq. 2-11), and Vss is 26.8 L. The initial or “central” distribution volume for the drug (V1 = dose/Cpo) is 16.1 L. The example chosen indicates that multicompartment kinetics may be overlooked when sampling at early times is neglected. In this particular case, there is only a 10% error in the estimate of clearance when the multicompartment characteristics are ignored. For many drugs, multicompartment kinetics may be observed for significant periods, and failure to consider the distribution phase can lead to significant errors in estimates of clearance and in predictions of the appropriate dosage. Also, the difference between the “central” distribution volume and other terms reflecting wider distribution is important in deciding a loading dose strategy. (Reproduced with permission from Buxton IO. Pharmacokinetics: The Dynamics of Drug Absorption, Distribution, Metabolism, and Elimination. In: Brunton LL, Hilal-Dandan R, Knollmann BC, eds. Goodman & Gilman’s: The Pharmacological Basis of Therapeutics, 13e New York, NY: McGraw-Hill; 2018.)

Figure 45–7
The determination of the half-life (t1/2) of a drug with first-order kinetics is illustrated. The drug concentration is graphed against the time. The time it takes for the concentration to decrease by 50% is indicated at two places on the curve. The t1/2 is the same for both determinations. (Reproduced with permission from Pharmacokinetics. In: Stringer JL, eds. Basic Concepts in Pharmacology: What You Need to Know for Each Drug Class, 5e New York, NY: McGraw-Hill; 2017.)

If a drug’s half-life is 4 hours and the plasma concentration is equal to 100 mg, then in 4 hours, drug concentration has fallen to 50 mg. Four hours later the drug concentration has been reduced to 25 mg, and after 4 more hours the concentration has fallen to 12.5 mg. Generally, it takes four to five half-lives to eliminate the drug from the body.14 Some drugs follow zero-order elimination in which the same amount of drug (not percentage) is eliminated in a specific period. This occurs when the metabolizing enzymes have become saturated.
Receptors are proteins embedded in cell membranes that contain a binding site. Drugs bind to these sites and cause a conformational change, leading to a cascade of events on a molecular level.15 Drugs may also bind to transport molecules, ion channels, enzymes that catalyze chemical reactions, nucleic acids, and some other miscellaneous targets. The binding of drugs to a receptor is specific and selective. Catecholamines like norepinephrine bind to sympathetic adrenergic receptors versus the parasympathetic muscarinic receptor, but it binds to more than one subtype of adrenergic receptor. It has affinity for α 1, α 2, β 1, and β 2 receptors. Beta blockers such as propranolol (Inderal) are nonselective for β 1 and β 2, whereas atenolol is selective for just β 1 receptors. Beta 1 receptors exist in the heart and when blocked by atenolol reduces heart rate and contractility, thus lowering blood pressure. However, as the concentration of atenolol increases, the drug binds to β 1 receptors in other areas of the body and may cause some unwanted effects. When a β 2 agonist is given as a rescue inhaler for asthma, β 1 receptors in the heart may also be stimulated and produce tachycardia. To minimize side effects, drugs should be specific for one receptor subtype.
The drug–receptor interaction may be illustrated with a dose–response curve in which dose is plotted along the X-axis and response is plotted on the Y-axis.16 As dose increases, so does response. However, at some point all the receptors are filled, and further increasing the dose does not alter the response. The dose–response curve is further affected by the presence of agonists or inhibitors (Fig. 45–8). A plateau is reached called Emax. Emax is a measure of drug efficacy. Converting the dose–response curve into a log-dose–response curve helps determine the ED50, which is the effective dose that produces 50% of the response, or one-half of the expected maximum. If two drugs have the same mechanism of action (i.e., dose–response curves are parallel) then the ED50 becomes a measure of potency. The drug with the lowest ED50 is the more potent drug, meaning that less drug is needed to produce the same effect.
Figure 45–8
Potential mechanisms of drug interaction with a receptor. Possible effects resulting from these interactions are diagrammed in the dose–response curves at the right. The traditional agonist (drug A)–receptor binding process results in the dose–response curve denoted “A alone.” B is a pharmacologic antagonist drug that competes with the agonist for binding to the receptor site. The dose–response curve produced by increasing doses of A in the presence of a fixed concentration of B is indicated by the curve “A+B.” Drugs C and D act at different sites on the receptor molecule; they are allosteric activators or inhibitors. Note that allosteric inhibitors do not compete with the agonist drug for binding to the receptor, and they may bind reversibly or irreversibly. (Reproduced with permission from Katzung BG. Introduction: The Nature of Drugs & Drug Development & Regulation. In: Katzung BG, eds. Basic & Clinical Pharmacology, 14e New York, NY: McGraw-Hill; 2018.)

The effect of exercise on pharmacokinetics of a drug may be profound. Exercise produces a shunting of blood flow away from the GI tract toward the heart, muscle, and subcutaneous tissues.17,18 Therefore, exercise should reduce absorption of orally administered drugs due to the reduction in splanchnic blood flow. However, because there is also reduction of blood flow to the liver, the drug that is absorbed through the GI tract may not undergo rapid metabolism, and blood level of the drug may increase and produce some level of toxicity. Elimination of the drug will also be reduced because there is a reduction in blood circulation to the kidney. To avoid possible drug–exercise interactions, it is best to exercise before one takes their daily medications. Just as exercise may affect drug pharmacokinetics, drugs may affect exercise performance. Blocking beta receptors reduces glycogenolysis and gluconeogenesis, adding to fatigue. Beta blockers also reduce recovery from hypoglycemia in patients with diabetes. Exercise enhances absorption of drugs injected into the subcutaneous or intramuscular tissues if administered near the area of injection. Thus, patients with insulin-dependent diabetes must take precaution to measure glucose level pre- and post-exercise and be ready to administer glucose if they become hypoglycemic.
Heat, ice, and massage may all affect pharmacokinetics of injected drugs. Heat and massage increase blood flow to the subcutaneous tissues and enhance absorption.18 This can have profound negative effects if the injected drug is an opioid or insulin. Enhanced absorption of opioids can produce respiratory depression and hypoglycemia in the case of insulin. Ice will have the opposite effect.
The term opioid refers to any substance that relieves pain and acts on the mu receptor and whose action is reversed with naloxone.19 Additionally, the term refers to the opium that exudes from an incision made in the Papaver somniferum poppy and contains morphine.20 Opioids are divided according to their affinity for the different opioid receptors, mu, delta, and kappa, which are found in the periphery, dorsal root ganglion, spinal cord, and brain. Opioids that are pure agonists at the mu receptor produce the strongest analgesia, and include morphine, fentanyl, methadone, and oxycodone.21 They have less affinity for the delta and kappa receptors. Codeine produces less receptor binding than morphine and therefore is used for mild to moderate pain.
Opioids mimic the action of our endogenous opioids, endorphins, and enkephalins. When the drug binds to the receptor, it inhibits pain impulses as they travel along the ascending pathways but also modulates the descending signals.19 Opioids work by activating potassium channels and inhibiting calcium channels, with the net effect being a hyperpolarization of the post-synaptic membrane. When the cell is made more negative, there is a reduction in release of the excitatory neurotransmitters such as glutamate and substance P. Noteworthy to mention is that norepinephrine (NE) reduces the descending inhibition of pain signals, but serotonin can produce both inhibitory and excitatory effects on pain signaling. As acute pain transitions into chronic pain, the contribution of the monoaminergic system increases over the opioid effects, and patients may respond better to an NE or serotonergic reuptake inhibitor. Additionally, a paradoxical condition may occur with long-term use of opioids called opioid-induced hyperalgesia21 (Fig. 45–9).
Figure 45–9
Potential receptor mechanisms of analgesic drugs. The primary afferent neuron (cell body not shown) originates in the periphery and carries pain signals to the dorsal horn of the spinal cord, where it synapses via glutamate and neuropeptide transmitters with the secondary neuron. Pain stimuli can be attenuated in the periphery (under inflammatory conditions) by opioids acting at μ-opioid receptors (MOR) or blocked in the afferent axon by local anesthetics (not shown). Action potentials reaching the dorsal horn can be attenuated at the presynaptic ending by opioids and by calcium blockers (ziconotide), α2 agonists, and, possibly, by drugs that increase synaptic concentrations of norepinephrine by blocking reuptake (tapentadol). Opioids also inhibit the postsynaptic neuron, as do certain neuropeptide antagonists acting at tachykinin (NK1) and other neuropeptide receptors. (Reproduced with permission from Schumacher MA, Basbaum AI, Naidu RK. Opioid Agonists & Antagonists. In: Katzung BG, eds. Basic & Clinical Pharmacology, 14e New York, NY: McGraw-Hill; 2018.)

Most of the opioids may be delivered in a variety of ways: transdermal, oral, intramuscular, intravenous, rectal, epidural, intranasal, continuous infusion, and transmucosal administration. There are also immediate-release preparations for breakthrough pain and extended-release preparations to provide basal analgesia (Table 45–2).
Drug | Approximate Equianalgesic Oral Dose | Approximate Equianalgesic Parenteral Dose | Recommended Starting Dose (Adults >50 kg) | Recommended Starting Dose (Children and Adults <50 kg) | ||
Oral | Parenteral | Oral | Parenteral | |||
Opioid Agonists | ||||||
Morphine | 30 mg/3–4 h | 10 mg/3–4 h | 15 mg/3–4 h | 5 mg/3–4 h | 0.3 mg/kg/3–4 h | 0.1 mg/kg/3–4 h |
Codeine | 130 mg/3–4 h | 75 mg/3–4 h | 30 mg/3–4 h | 30 mg/2 h (IM/SC) | 0.5 mg/kg/3–4 h | Not recommended |
Hydromorphone | 6 mg/3–4 h | 1.5 mg/3–4 h | 2 mg/3–4 h | 0.5 mg/3–4 h | 0.03 mg/kg/3–4 h | 0.005 mg/kg/3–4 h |
Hydrocodone (typically with acetaminophen) | 30 mg/3–4 h | Not available | 5 mg/3–4 h | Not available | 0.1 mg/kg/3–4 h | Not available |
Levorphanol | 4 mg/6–8 h | 2 mg/6–8 h | 4 mg/6–8 h | 2 mg/6–8 h | 0.04 mg/kg/6–8 h | 0.02 mg/kg/6–8 h |
Meperidine | 300 mg/2–3 h | 100 mg/3 h | Not recommended | 50 mg/3 h | Not recommended | 0.75 mg/kg/2–3 h |
Methadone | 10 mg/6–8 h | 10 mg/6–8 h | 5 mg/12 h | Not recommended | 0.1 mg/kg/12 h | Not recommended |
Oxycodone | 20 mg/3–4 h | Not available | 5 mg/3–4 h | Not available | 0.1 mg/kg/3–4 h | Not available |
Oxymorphone | 10 mg/3–4 h | 1 mg/3–4 h | 5 mg/3–4 h | 1 mg/3–4 h | 0.1 mg/kg/3–4 h | Not recommended |
Tramadol | 100 mg | 100 mg | 50–100 mg/6 h | 50–100 mg/6 h | Not recommended | Not recommended |
Fentanyl Transdermal 72-h patch (25 μg/h) = morphine 50 mg/24 h | ||||||
Opioid Agonist-Antagonists or Partial Agonists | ||||||
Buprenorphine | Not available | 0.3–0.4 mg/6–8 h | Not available | 0.4 mg/6–8 h | Not available | 0.004 mg/kg/6–8 h |
Butorphanol | Not available | 2 mg/3–4 h | Not available | 2 mg/3–4 h | Not available | Not recommended |
Nalbuphine | Not available | 10 mg/3–4 h | Not available | 10 mg/3–4 h | Not available | 0.1 mg/kg/3–4 h |
Opioids are well absorbed when given via injection or taken through the oral route. Morphine, however, has a large first-pass effect necessitating a much larger dose given orally than via injection; in contrast, codeine and oxycodone have a low first-pass metabolism and therefore are effective orally. Once absorbed, opioids are distributed to highly perfused tissues like the brain but accumulate in fatty tissue and are metabolized by hepatic enzymes. Whereas the CYP3A4 P450 enzyme metabolizes fentanyl, producing nonactive metabolites, the CYP2D6 P450 enzyme metabolizes codeine, oxycodone, and hydrocodone, producing active metabolites with greater affinity for their receptors. However, individuals with a genetic polymorphism of CYP2D6 enzyme are poor metabolizers and may not receive pain relief.
Opioids produce both transient and persistent adverse effects. The transient effects include euphoria, nausea and vomiting, pruritus, cognitive dysfunction, diminished psychomotor performance, sedation, and urinary retention. The nausea and vomiting occur because of the drug’s effect on the chemoreceptor trigger zone and effects on the vestibular system. These symptoms will likely lessen with time as the patient develops tolerance. Persistent adverse effects include meiosis and constipation. Patients also develop tolerance to respiratory depression; however, this is lethal if the dose is increased beyond the tolerable level. Respiratory depression is more likely to occur when dosed along with a benzodiazepine and/or when the patient suffers from certain medical conditions, such as cancer, sleep apnea, and chronic obstructive pulmonary disease. Treatment for an overdose includes IV or intramuscular (IM) administration of the opioid antagonist naloxone. Naloxone can reverse respiratory depression and hypotension within 1 to 2 minutes with IV administration, and in 2 to 5 minutes with IM or subcutaneous (SC) administration.22 Because access to peripheral veins may be difficult to find in IV drug abusers, in 2016, the Food and Drug Administration (FDA) approved an intranasal formulation of naloxone called Narcan Nasal Spray. Narcan is effective in 8 to 13 minutes after intranasal administration. Repeated doses may be necessary because the half-life of naloxone is one of the shortest. Naloxone precipitates acute opioid withdrawal with the following symptoms: piloerection, hypertension, yawning, sneezing, chills, insomnia, diarrhea, nausea, vomiting, and abdominal and muscle aches lasting from 1 to 14 days.23,24
Opioids are extremely effective agents for pain reduction. However, they are one of the most abused drugs available. The Centers for Disease Control and Prevention (CDC) reported 33,000 deaths in the United States from opioid overdoses in 2015, and 4.31% of Americans age 12 and older misuse prescription pain relievers.25 A Blue Cross Blue Shield study showed that opioid abuse has increased 493% from 2010 to 2016. Opioids activate the dopaminergic neurons, thus providing strongly pleasant sensations. However, tolerance develops rapidly so that in as little as 2 weeks, patients will need higher doses to achieve the same effect. This leads to both physical dependence and addiction. Having intense cravings for the drug along with obsessive thoughts about when and how to obtain the next fix are cardinal features of addiction, whereas physical dependence is present when symptoms appear with abrupt drug withdrawal.
A comprehensive approach to opioid addiction includes counseling along with certain opioid medications, methadone and buprenorphine, with or without naltrexone. Methadone is a synthetic opioid that blocks heroin from binding to the receptor and prevents withdrawal symptoms. It does not produce euphoria and has a much longer half-life, 1 to 2 days compared to 4 to 6 hours for heroin. Thus, patients can be switched to methadone and then undergo a slow reduction in dose over time. Methadone has some risks, in that it can cause prolonged QT interval and arrhythmias, and when combined with a serotonin reuptake inhibitor, may cause serotonin syndrome.22 Because it has a long half-life, initial titration takes some time. Withdrawal from a short-acting opioid is much more severe than withdrawal from an agent with a long half-life. Buprenorphine is a partial agonist at the mu receptor but has antagonist properties at the other receptors. When given to patients addicted to morphine or heroin, it may precipitate withdrawal, and therefore the first dose must not be given until moderate withdrawal symptoms are already present. Agents that can be used in the detox phase include other opioid agonists, alpha-2 agonists (clonidine), nonsteroidal anti-inflammatories, anticholinergics, and antacids. Additionally, antianxiety medication may be needed Naloxone combined with buprenorphine (Suboxone, 1:4) in a sublingual tablet or film is used for maintenance and to prevent relapse.20 If patients are using an opioid concurrently with a benzodiazepine, it is safer to undergo detox from the opioid first and then slowly reduce the tranquilizer.26 Abrupt discontinuation of a benzodiazepine can trigger rebound anxiety, seizures, and hallucinations.
Given the number of individuals addicted to pain killers, plus the increasingly high rates of overdoses, the FDA, drug companies, regulators, and medical practitioners are working toward developing strategies to reduce abuse. These risk mitigation strategies include education for the prescribers, abuse deterrent formulations, and drug monitoring programs. Drug deterrent formulations include using excipients such as polyethylene oxide, hyperabsorbent materials, lipids, or ceramic nanoparticles.27,28 Some of these turn into a gel when exposed to water or prevent pulverization when crushed—all are designed to prevent the addict from crushing the tablet to enhance absorption or to make it unsuitable for injection.
There are several items to consider when prescribing opioids for pain control. Clinicians should discuss the risks and benefits of opioid therapy. It is critical for patients to understand the adverse effects as well as the addictive nature of the drugs. Clinicians should prescribe the lowest dose possible, for the shortest amount of time, without any dose escalation for acute pain.26 Ideally, clinicians will prescribe short- and long-acting opioids that belong to the same family (i.e., avoidance of prescribing oxycodone, morphine, and hydrocodone concurrently), as this will allow for an opioid rotation in the future if necessary. Emphasis should be on improving function even if pain remains because there is little evidence that continued use will result in a reduction of chronic pain.29 The concepts of tolerance should also be reviewed. Tolerance wears off quickly, so patients taking a drug holiday should not resume with the same amount of drug consumed previously, but should reduce the dose to avoid respiratory depression.
If an opioid is prescribed for chronic pain, the clinician should re-evaluate the patient within 1 to 4 weeks, especially if there has been a history of abuse. A urine drug screen may be part of that assessment if the patient is suspected of adding other opioids or benzodiazepines. The urine screen can identify drugs or metabolites consumed within 1 to 5 days.30 A blood test provides information on more recent use. Clinicians may also administer the Screener and Opioid Assessment for Patients with Pain-Revised (SOAPP-R). This is a tool to assist the prescriber in determining risk for deviant behavior by patients who will be receiving opioids for chronic pain. It consists of 24 questions that ask about mood swings, cravings for medications, alcohol use, and other items that might suggest the patient is at high risk for addiction.31 A Google search with the title of the test will reveal a variety of websites that publish the survey. The Current Opioid Misuse Measure (COMM) is another tool that can be used to identify aberrant behavior.32 The reader is also referred to the CDC’s Opioid Overdose webpage (https://www.cdc.gov/drugoverdose/prescribing/resources.html) containing videos, training, clinical tools, and posters for clinicians to help them disseminate information about the dangers of opioids.
Other therapeutic concerns include recognizing that patients may experience drowsiness and dulled cognitive function on these drugs and therefore should not be operating any dangerous equipment. Patients wearing a fentanyl or lidocaine patch or any transdermal delivery system must avoid heat, pressure, or exercise in the area near the patch to avoid an increase in absorption.33 Additionally, great care should be taken to not increase the dosage in a short period, as it takes 72 hours to reach steady-state levels in the plasma. Premature dose escalation with a fentanyl patch may lead to respiratory depression or even death. Patients wearing patches must also avoid sleeping with infants or small children, as there have been cases in which the patch has disengaged from the patient and ended up on the baby during sleep, resulting in severe respiratory depression.34 Opioids are excellent agents to reduce pain, but their risk may quickly outweigh their benefits.
This section will focus primarily on pharmacology for schizophrenia. Rehabilitation professionals should be aware that physical activity and exercise can reduce symptoms of schizophrenia and have recently been considered an important adjunct to pharmacotherapy.35
Prevailing theories of schizophrenia pathophysiology show dysfunction in the dopamine, serotonin, glutamate, and gamma-aminobutyric acid (GABA) neurotransmitters.36 The dopamine and serotonin hypotheses of schizophrenia are the most widely accepted. In schizophrenia, dopamine levels are increased in the mesolimbic and mesocortical pathways.37 Antipsychotic medications were originally developed to decrease the levels of dopamine by blocking dopamine in limbic and cortical areas.
Antipsychotics can be classified as first generation and second generation.38 First-generation antipsychotics (FGAs) (Table 45–3) are dopamine receptor-2 (D2) antagonists. Typical antipsychotic drugs are known for their extrapyramidal side effects. Extrapyramidal symptoms include various abnormal movements such as dystonia (sustained abnormal postures and muscle spasm), akathisia (restlessness and pacing), parkinsonism (tremors and rigidity), and tardive dyskinesia (repetitive involuntary facial movements).39 In rare cases, the potentially fatal neuroleptic malignant syndrome may be seen with typical antipsychotics, which is characterized by rigidity, fever, and increase plasma creatine kinase.40 Although the incidence of extrapyramidal symptoms varies among specific FGAs, these side effects have been reported as high as in 50% of patients treated with high-potency FGAs (haloperidol). The therapeutic effect of FGAs occurs when 60% to 80% of D2 receptors are blocked; extrapyramidal symptoms have been shown to occur at 75% to 80% of D2 receptor occupancy,41 leaving a very small window for a therapeutic effect without serious side effects.
Individually, different FGAs have actions on alpha-adrenergic,42 histaminic,43 and muscarinic receptors.44 These actions are associated with a high side effect profiles that result in nonselective blocking of these receptors throughout the body. Generally, high-potency FGAs have low histaminergic, muscarinic, and sedative side effects but a high risk of extrapyramidal signs, whereas low-potency FGAs have high muscarinic and histaminergic side effects, with a lower risk of extrapyramidal signs.45
FGAs have limited bioavailability, due to extensive first-pass metabolism by the liver.46 The drug’s reliance on hepatic metabolism increases its drug–drug interactions and potential for liver impairment.47 Antipsychotics and many antidepressants share the metabolizing enzyme cytochrome P450. Sharing the same metabolizing enzyme increases the risk for drug–drug interactions; with that said, interactions have been shown to be mild and readily manageable.48
Most antipsychotics are highly lipid soluble, easily passing the blood–brain barrier.
FGAs also have large volumes of distribution and a long clinical duration due to binding to the D2 receptors for a prolonged period.49 Urine excretion of FGAs may occur weeks after the last dose, and with some injectable antipsychotics, this may be 3 to 6 months later. Time to symptom recurrence is highly variable among antipsychotics.50
The action of second-generation antipsychotics (SGAs) (Table 45–4), or atypical antipsychotics, generally has a higher affinity for blocking serotonin receptors than dopamine. This significantly reduces their ability to cause extrapyramidal symptoms.40,51 Although SGAs still bind to the dopamine receptors, they do so to a much lesser extent, for a shorter period,52 and selectively in areas not responsible for movement.
Clozapine* (Clozaril) Olanzapine (Zyprexa) Quetiapine (Seroquel) | Risperidone (Risperdal) Paliperidone (Invega) Ziprasidone (Geodon) Lurasidone (Latuda) | Aripiprazole (Abilify) Brexpipazole (Rexulti) Cariprazine (Vraylar) |
SGAs and FGAs do not differ in their effectiveness in treating schizophrenia; the most important difference between SGAs and FGAs is their ability to generate extrapyramidal side effects.53 SGAs are still capable of producing these movement disorders to some degree, to a much lesser extent than FGAs, but the risk depends on the specific drug.39 All patients on any antipsychotics should be questioned about any tremors, slowed movement, or restlessness.
Absorption and bioavailability of SGAs are variable among specific drugs.54 SGAs are available as immediate-release and long-acting injectables and as orally disintegrating tablets.55 Oral dosing is the general method of administration for SGAs, but long-lasting antipsychotics may be injected every 2 to 4 weeks for patients unable to adhere to an oral drug.56 Specific SGAs vary significantly in regard to their dosing, absorption rates, half-life, drug–drug interactions, and hepatic and renal response. This should be kept in mind when treating patients on these drugs, but the specifics are beyond the scope of this text.
Although the aforementioned extrapyramidal symptoms are the largest concern with antipsychotic medications, both typical and atypical antipsychotics have metabolic, anticholinergic, cardiovascular, and sexual side effects.57 All antipsychotics unintentionally block or partially block muscarinic, histaminergic, and alpha-adrenergic receptors. Anticholinergic side effects from blocking muscarinic (cholinergic) receptors result in dry mouth and constipation; these side effects are not serious and are often manageable.
Cardiac and metabolic abnormalities are extremely important to consider with both FGAs and SGAs, with some sources showing a higher risk of cardiac abnormalities in SGAs.58 Patients with schizophrenia die, on average, 25 years earlier than the general population, with cardiovascular-related deaths being the main cause.59 This increases the importance of prescribing exercise programs and promoting healthy habits in patients with schizophrenia.
Although alpha-adrenergic receptor blocking is thought to decrease positive symptoms of schizophrenia, these receptors are also present in the heart.60 Alpha-adrenergic receptor blockers, which are used to treat high blood pressure,61 can result in excessively low blood pressure and other cardiac side effects. Prolonged QT interval has been demonstrated in patients with cardiac risk factors on antipsychotics, making an electrocardiogram an important recommendation in patients starting such drugs.62 Orthostatic hypotension is also a common cardiac antipsychotic side effect.63 Orthostatic hypotension often results in compensatory tachycardia, increasing resting and exercising heart rates. Rehabilitation professionals should be constantly monitoring cardiac parameters in all patients on antipsychotics, as these side effects are particularly common.
The blocking of histamine receptors in antipsychotics is thought to result in the metabolic side effects of weight gain, diabetes, and dyslipidemia. These side effects may vary depending on the specific drug, and should be an important consideration, considering the high incidence of cardiac-related deaths in this population.43,64 These metabolic side effects are associated with FGAs and SGAs to a similar extent.
Histaminergic pathway blocking also results in sedation. In 2017, the Food and Drug Administration released a warning regarding the risk of falls and hip fractures in elderly individuals taking antipsychotics. A combination of sedation, orthostatic hypotension, and potential motor impairments has been shown to increase falls in the elder populations.65 In addition, all FGAs and SGAs have sexual dysfunction as a common side effect due to dopamine blocking in the pituitary gland, resulting in excessive prolactin release. This hyperprolactinemia is associated with menstrual cycle and sexual dysfunction, including impaired desire, arousal, and orgasm.66 The specific occurrence of sexual dysfunction depends on the specific drug.
According to the Centers for Disease Control and Prevention, antidepressants are among the top three most commonly prescribed drugs. In addition to depression being common in individuals with pain, antidepressants themselves are first-line treatments for pain syndromes and neuropathic pain.67–69 The monoamine hypothesis of depression claims deficiencies in serotonin, norepinephrine, and/or dopamine result in depressive symptoms,70,71 with just about all antidepressants targeting restoring monoamine neurotransmitter levels.
Monoamine oxidase inhibitors (MAOIs) and tricyclic antidepressants (TCAs) are the first-generation antidepressants. Monoamine oxidase is an enzyme responsible for metabolizing serotonin, norepinephrine, and dopamine. MAOIs cause long-lasting, irreversible inhibition of the monoamine oxidase enzyme, leaving the neurotransmitters for use in the synaptic cleft. MAOIs were found to also inhibit the reuptake of norepinephrine in the autonomic nervous system. This can lead to agitation, hyperthermia, restlessness, dry mouth, urinary retention, and hypertension. Dangerous interactions with MOAIs were found with sympathomimetics (over-the-counter cold and cough medications) and food containing tyramine (meats, pork, chocolate, bananas, eggplant, cheese, beer, red wine, raisins, and avocados), resulting in hypertensive crisis.
TCAs block serotonin and norepinephrine reuptake transporters, increasing the concentration of these neurotransmitters in the synapse. Signs of anticholinergic side effects and atrioventricular (AV) heart block should be monitored; patients on MAOIs or TCAs should have their vitals taken often to assure their blood pressure and heart rate responses are normal. Patients should be asked if they feel dizzy or if they feel their heart flutter. Exercise releases norepinephrine, making it important to monitor patients on these drugs when performing activities.
Second-generation antidepressants (Table 45–4) are far more commonly used and have fewer side effects and less toxicity. Second-generation antidepressants include selective serotonin reuptake inhibitors (SSRIs) and serotonin norepinephrine reuptake inhibitors (SNRIs). SSRIs are the most prescribed and utilized antidepressant.72,73 Similar to TCAs, SSRIs and SNRIs act on the transport molecules that return neurotransmitters to the neuron to be metabolized. SNRIs can be thought of as an updated version of TCAs, as they do not unintentionally block alpha-1-adrenergic, cholinergic, dopaminergic, or histaminergic receptors, as seen with TCAs.74 Clinicians should recognize that most patients will not achieve remission after initial treatment with second-generation antidepressants.71 It takes 2 to 3 weeks before a clinical response is seen after initiating antidepressants.75
SSRIs are highly lipid soluble and have a large volume of distribution. They are easily absorbed in the GI tract and have peak plasma levels between 1 and 8 hours.76 Hepatic metabolism and elimination occurs with all SSRIs (except fluvoxamine). Drug interactions from inhibiting the metabolizing enzyme cytochrome P450 in the liver may occur, but this depends on the specific drug. SNRIs do not have effects on the P450 enzymes and have subsequently fewer drug–drug interactions than SSRIs.
The most common atypical antidepressant that rehabilitation professionals should be aware of is bupropion (trade names Wellbutrin or Zyban). Bupropion has been found to minimize sexual dysfunction and have stimulating effects, which is one reason this may be prescribed over an SSRI. The stimulating effect of bupropion may be preferable in patients suffering from fatigue and poor concentration.77 However, some evidence shows these drugs are associated with seizures and cardiac abnormalities in certain individuals (Table 45–5).
Side Effects | ||||||||||||
Class Agent | Doseamg/d | Biogenic Amine | Agitation | Seizures | Sedation | Hypo-Tension | Anti-Ach Effects | Gi Effects | Weight Gain | Sexual Effects | Cardiac Effects | |
NE reuptake inhibitors: 3° amine tricyclics | ||||||||||||
Amitriptyline | 100–200 | NE, 5HT | 0 | 2+ | 3+ | 3+ | 3+ | 0/+ | 2+ | 2+ | 3+ | ![]() |
Clomipramine | 100–200 | NE, 5HT | 0 | 3+ | 2+ | 2+ | 3+ | + | 2+ | 3+ | 3+ | |
Doxepin | 100–200 | NE, 5HT | 0 | 2+ | 3+ | 2+ | 2+ | 0/+ | 2+ | 2+ | 3+ | |
Imipramine | 100–200 | NE, 5HT | 0/+ | 2+ | 2+ | 2+ | 2+ | 0/+ | 2+ | 2+ | 3+ | |
(+)-Trimipramine | 75–200 | NE, 5HT | 0 | 2+ | 3+ | 2+ | 3+ | 0/+ | 2+ | 2+ | 3+ | |
NE reuptake inhibitors: 2° amine tricyclics | ||||||||||||
Amoxapine | 200–300 | NE, DA | 0 | 2+ | + | 2+ | + | 0/+ | + | 2+ | 2+ | ![]() |
Desipramine | 100–200 | NE | + | + | 0/+ | + | + | 0/+ | + | 2+ | 2+ | |
Maprotiline | 100–150 | NE | 0/+ | 3+ | 2+ | 2+ | 2+ | 0/+ | + | 2+ | 2+ | |
Nortriptyline | 75–150 | NE | 0 | + | + | + | + | 0/+ | + | 2+ | 2+ | |
Protriptyline | 15–40 | NE | 2+ | 2+ | 0/+ | + | 2+ | 0/+ | + | 2+ | 3+ | |
SSRIs | ||||||||||||
(±)-Citalopram | 20–40 | 5HT | 0/+ | 0 | 0/+ | 0 | 0 | 3+ | 0 | 3+ | 0 | ![]() |
(+)-Escitalopram | 10–20 | 5HT | 0/+ | 0 | 0/+ | 0 | 0 | 3+ | 0 | 3+ | 0 | |
(±)-Fluoxetine | 20–80 | 5HT | + | 0/+ | 0/+ | 0 | 0 | 3+ | 0/+ | 3+ | 0/+ | |
Fluvoxamine | 100–200 | 5HT | 0 | 0 | 0/+ | 0 | 0 | 3+ | 0 | 3+ | 0 | |
(−)-Paroxetine | 20–40 | 5HT | + | 0 | 0/+ | 0 | 0/+ | 3+ | 0 | 3+ | 0 | |
(+)-Sertraline | 100–150 | 5HT | + | 0 | 0/+ | 0 | 0 | 3+ | 0 | 3+ | 0 | |
(±)-Venlafaxine | 75–225 | 5HT, NE | 0/+ | 0 | 0 | 0 | 0 | 3+ | 0 | 3+ | 0/+ | |
Atypical antidepressants | ||||||||||||
(−)-Atomoxetine | 40–80b | NE | 0 | 0 | 0 | 0 | 0 | 0/+ | 0 | 0 | 0 | ![]() |
Bupropion | 200–300 | DA, ?NE | 3+ | 4+ | 0 | 0 | 0 | 2+ | 0 | 0 | 0 | |
(+)-Duloxetine | 80–100 | NE, 5HT | + | 0 | 0/+ | 0/+ | 0 | 0/+ | 0/+ | 0/+ | 0/+ | |
(±)-Mirtazapine | 15–45 | 5HT, NE | 0 | 0 | 4+ | 0/+ | 0 | 0/+ | 0/+ | 0 | 0 | |
Nefazodone | 200–400 | 5HT | 0 | 0 | 3+ | 0 | 0 | 2+ | 0/+ | 0/+ | 0/+ | |
Trazodone | 150–200 | 5HT | 0 | 0 | 3+ | 0 | 0 | 2+ | + | + | 0/+ | |
MAO inhibitors | ||||||||||||
Phenelzine | 30–60 | NE, 5HT, DA | 0/+ | 0 | + | + | 0 | 0/+ | + | 3+ | 0 | ![]() |
Tranylcypromine | 20–30 | NE, 5HT, DA | 2+ | 0 | 0 | + | 0 | 0/+ | + | 2+ | 0 | |
(−)-Selegiline | 10 | DA, ?NE, ?5HT | 0 | 0 | 0 | 0 | 0 | 0 | 0 | + | 0 |
The SNRI duloxetine (Cymbalta) along with the anticonvulsants (gabapentin or pregabalin) are first-line drugs for the treatment of neuropathic pain67,78 according to the 2017 National Institute for Health and Care Excellence (NICE) Guidelines for pharmacologic management of neuropathic pain.69,79 The mechanisms by which antidepressants produce analgesia have been theorized to relate to serotonin acting in descending pain pathways. Serotonin has been found to be released in the synapses of this pathway, with higher levels resulting in pain inhibition.
Duloxetine (Cymbalta) is specifically indicated as a first-line neuropathic drug.80 SNRIs have been efficacious in the treatment of diabetic peripheral neuropathy,81 fibromyalgia,82 and even chronic musculoskeletal pain,83 including low back pain.84
SNRI side effects most commonly include nausea, diarrhea, dry mouth, and constipation.85 TCAs have a stronger affinity for histaminergic and cholinergic receptors, resulting in patients experiencing dry mouth, constipation, blurred vision, urinary retention, and postural hypotension.74 In addition, one of the most poorly tolerated adverse effects of TCAs includes drowsiness. Cardiac arrhythmias or palpitation may also occur with TCAs,86 and patients with arrhythmias may be at risk for cardiac toxicity. TCAs are generally less tolerable in the elderly population due to potential cardiac and strong anticholinergic side effects (Table 45–6).
Gen. | Class | Mechanism of Action | Common Drugs |
1st | Monoamine oxidase inhibitor (MAOI) | Inhibition of monoamine oxidase enzyme | Phenelzine (Nardil) |
Selegiline (Emsam) | |||
Tranylcypromine (Parnate) | |||
Tricyclic (TCA) | Inhibit serotonin and norepinephrine from combining to their reuptake transporters | Amitriptyline* (Elavil) | |
Clomipramine* (Anafranil) | |||
Imipramine* (Tofranil) | |||
2nd | Selective Serotonin Reuptake Inhibitor (SSRI) | Selectively inhibit serotonin from binding to its reuptake transporters | Escitalopram (Lexapro) |
Fluoxetine (Prozac) | |||
Fluvoxamine (Faverin) | |||
Paroxetine (Paxil) | |||
Sertraline (Zoloft) | |||
Citalopram (Celexa) | |||
Serotonin Norepinephrine Reuptake Inhibitor (SNRI) | Block norepinephrine and serotonin transporters, lack the alpha, anticholinergic and antihistaminic blocking actions TCAs. | Duloxetine* (Cymbalta) | |
Venlafaxine* (Effexor) | |||
Tetracyclic/ Unicyclic | Varying structures which do not belong to a specific class. Many mechanisms of action are unknown. | Amoxapine* (Asendin) | |
Bupropion (Wellbutrin) | |||
Mirtazapine (Remeron) | |||
Serotonin Receptor Modulators | Block a subgroup of serotonin receptors. | Nefazodone (Serzone) | |
Trazodone (Oleptro) |
Although SSRIs are known for their safety and tolerability compared with other antidepressants and most adverse effects are minor, they frequently lead to discontinuation of treatment. Insomnia, sedation, appetite changes, nausea, dry mouth, headache, and sexual dysfunction are considered common adverse reactions.87 Agitation, impulsivity, and irritability are slightly less common. Sexual dysfunction in particular has been seen as high as 73% of patients on SSRIs.88 Weight gain may be a concern with SSRIs, but the literature is unclear, with some studies showing 12% of patients on the drugs gaining weight but others showing no association with weight changes. Patients should also be educated on “discontinuation symptoms,” which is a phenomenon that occurs when discontinuing an SSRI that results in symptoms such as dizziness, lightheadedness, insomnia, fatigue, anxiety/agitation, nausea, headache, and sensory disturbance that may last up to 3 weeks.89
SNRIs and SSRIs share the same side effects. SNRI side effects most commonly included nausea, diarrhea, dry mouth, and constipation.85 In addition, an SNRI’s ability to increase norepinephrine release adds side effects related to an increase in sympathetic nervous system output. This may include elevated blood pressure, nausea, dizziness, and diaphoresis. MAOIs should not be taken with an SNRI or SSRI or within 4 weeks of each other.90 SSRIs lead to serotonin syndrome, a life-threatening condition characterized by excess serotonin, particularly when there is overlap with another serotonergic drug. Serotonin syndrome (Table 45–7) is diagnosed clinically and includes muscular, autonomic, and mental changes.
System | Symptoms |
Neuromuscular | Hyperreflexia, clonus, tremors, rigidity, bilateral Babinski’s sign, akathisia |
Autonomic | Diaphoresis, tachycardia, hyperthermia, hypertension, vomiting, and diarrhea, dilated pupils, dry mucous membranes |
Mental Status | Anxiety, agitation, delirium, restlessness, disorientation, easily startled |
Although anticonvulsant medications are known for their role in seizure control and epilepsy, certain anticonvulsants are first-line medications for neuropathic pain.67,78 Gabapentin (Neurontin) and pregabalin (Lyrica) have especially been found to be efficacious in diabetic neuropathy, postherpetic neuralgia,91,92 and reflex sympathetic dystrophy.93
Gabapentin and pregabalin are structural analogues (Table 45–7) of the inhibitory neurotransmitter GABA. The original intent for these medications was to mimic the neurotransmitter GABA, acting GABAergically. However, the mechanism of action was different than originally intended, and the drugs ended up being more involved with modifying glutamate release.94 Acting presynaptically, they decrease calcium entrance into the neuron, thus reducing glutamate release (Table 45–8).
Anticonvulsants | ||
First-line treatment of neuropathic pain67,69,78,79 | Some evidence for treatment of neuropathic pain | No evidence for treatment of neuropathic pain |
Gabapentin (Neurontin) Pregabalin (Lyrica) | Carbamazepine* (Tegretol) Clonazepam (Klonopin) Oxcarbazepine (Trileptal) Topiramate (Topamax) Valproate (Valproic Acid) Lamotrigine (Lamictal) Phenytoin (Dilantin) | Tiagabine (Gabitril) Zonisamide (Zonegran) Phenobarbital (Luminal) |
Unlike most anticonvulsant drugs, which are metabolized, gabapentin and pregabalin are not metabolized and are eliminated unchanged, solely by the renal system.96 This lack of hepatic metabolism decreases drug–drug interactions that may be present when using antidepressants. The bioavailability of gabapentin and pregabalin is not affected by food intake and remains unchanged following multiple dose administration. Neither drug is bound to plasma proteins, increasing its ability to diffuse through cell membranes and tissue. Both drugs have half-lives roughly from 4 to 8 hours, requiring dosing more than once a day.
Side effects of gabapentin and pregabalin mostly relate to central nervous system depression. The two most common adverse effects were sedation and dizziness. Studies show about 23.9% of patients experienced dizziness and 27.4% experience sedation with gabapentin. Ataxia was seen in 7.1% and peripheral edema in 9.7%.97 Headache, tremor, and confusion were also noted in patients on these drugs. Sexual side effects including libido, anejaculation, anorgasmia, and impotence were also seen.98 In general, gabapentin and pregabalin are considered safe and tolerable drugs.99
Most studies show that the antiepileptic drugs (AEDs) were more efficacious in reducing neuropathic pain than antidepressants. Individuals on membrane stabilizers generally experienced fewer side effects than on antidepressants, with 38% of patients reporting side effects with the serotonin-norepinephrine reuptake inhibitor duloxetine (Cymbalta) and 30% with gabapentin (Neurontin).100 A 2017 systematic review showed that tricyclic antidepressants and membrane stabilizers had the same efficacy in treating diabetic neuropathy, but antidepressants had higher dropout rates due to adverse reactions.92
Local anesthesia refers to the elimination of sensation at a specific area of the body. Although analgesia is the goal of most local anesthetics, they often diminish all sensation from the specified area, explaining their classification as an anesthetic. Local anesthetics can be used in a variety of ways. Neuraxial analgesia refers to local anesthetics being placed in the central nervous system, as with epidural injections. Peripheral nerve blocks are also accomplished using local anesthetics. Infiltration is another method of local anesthesia, often used by dentists to numb areas of the mouth before a procedure.
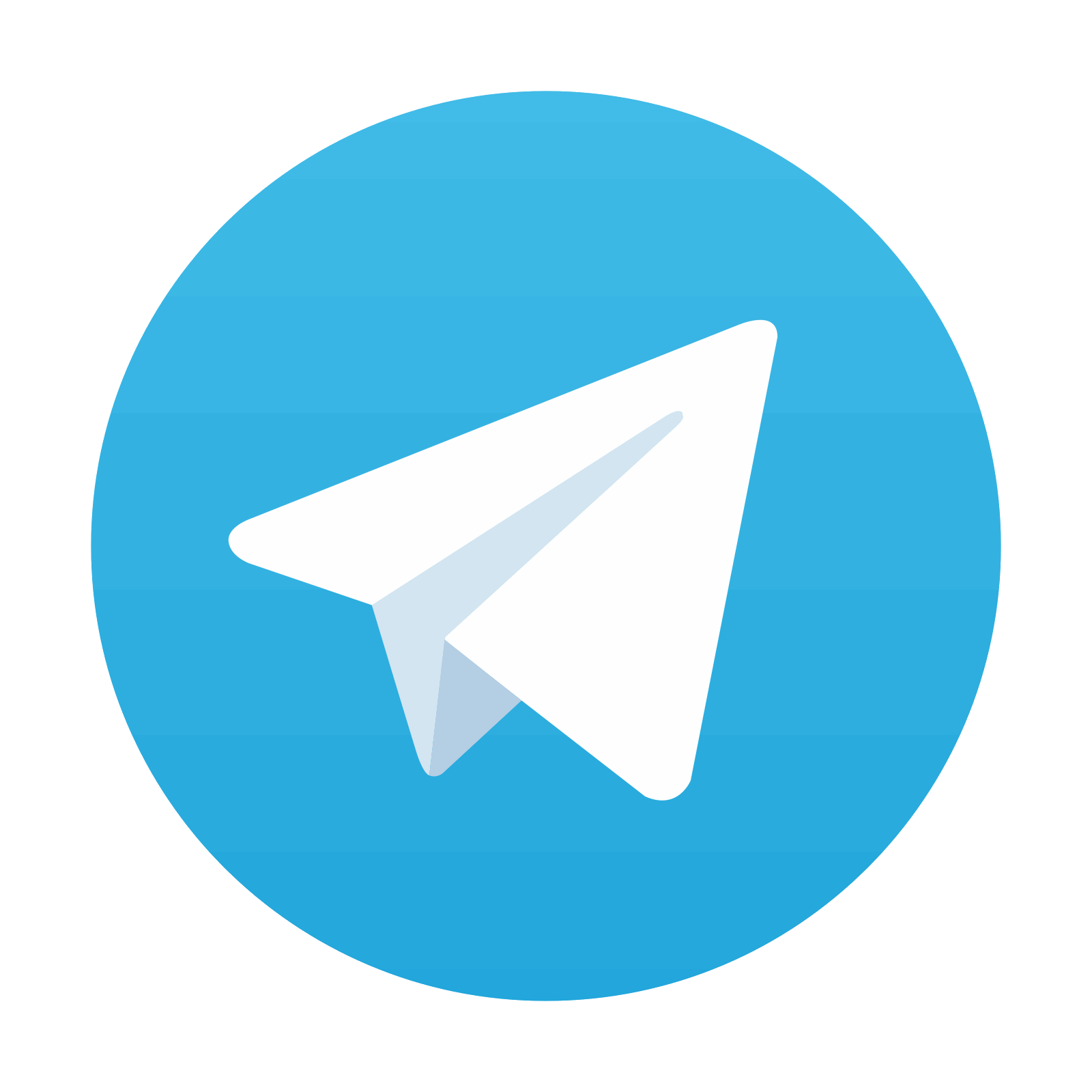
Stay updated, free articles. Join our Telegram channel

Full access? Get Clinical Tree
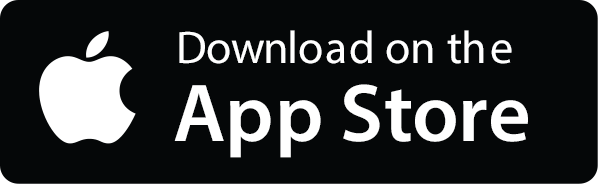
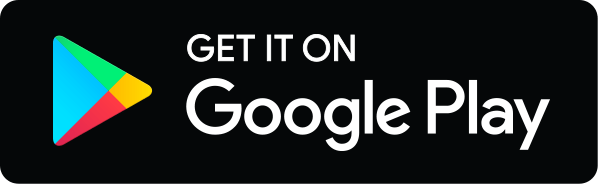