Introduction
Fluoroscopy has played a central role for injection therapies of the spine and peripheral joints, for both interventional pain management and orthopedics. In this chapter, we focus on the principles and the use of fluoroscopy regarding both spinal and peripheral interventions as it applies to the emerging field of regenerative medicine. Fields such as interventional radiology, sports medicine, physiatry, and orthopedics rely on fluoroscopy to enhance the accuracy and effectiveness of procedures. As these fields have incorporated regenerative injectates, it is a natural progression to use fluoroscopic guidance for more precise delivery of the biologic treatment to the injured tissue. Fluoroscopy allows providers to precisely, efficiently, and safely direct the needle and literally “pinpoint” the target location while minimizing damage to unintended structures. By using bony landmarks and confirmation with contrast, there is a very high degree of certainty that injectates reach the target tissues and give the best possible chance of therapeutic success. In addition, fluoroscopy allows for precision injection of materials, be it cement or orthobiologics into bone. Simply placing a needle into bone does not ensure placement and retention of the material into bone, and one can frequently see venous vascular flow of contrast out of the bone and away from the intended targets. Small adjustments of the needle tip can make a substantial difference in the deposition of the treatment injectate.
A keen sense of anatomy, trajectory, and understanding of vital structures is a crucial and required skill set for any interventionalist performing these therapies. Although it may be inferred, these procedures should only be performed by well-qualified physicians who have completed adequate postdoctoral training. Many injections, including joint injections, require experience, spatial awareness, and anatomic understanding so as not to puncture nerves, vessels, or critical structures that may be in the needle path. In the setting of fluoroscopic guidance, the superficial anatomy, manual palpation, and bony landmarks on the images serve as guides for the needle’s pathway. The developing field of regenerative orthopedics relies on the knowledge and techniques of traditional diagnostic and therapeutic injections of the spine, joints, nerves, and soft tissues. In this chapter, we discuss the principles of fluoroscopy as it applies to diagnosis and treatment of musculoskeletal conditions.
Radiation Terminology and Measurement
There are numerous terms and units of measurement used in radiology, and one must be familiar with their value and relationship to each other. Radiation can be described in terms of radioactivity, exposure, absorbed dose, and dose equivalent ( Table 3.1 ). In general:
- •
1 R (exposure) = 1 rad (absorbed dose) = 1 rem or 1000 mrem (dose equivalent)
Definition | Unit Measurements | Relationships | |
---|---|---|---|
Radioactivity | Amount of radiation released by a material | Curie (Ci) Becquerel (Bq) | 1 Ci = 3.7 × 10 10 Bq |
Exposure | Amount of radiation exiting the x-ray tube, traveling through the air | Roentgen (R) Coulomb/kilogram (C/kg) | 1 R = 2.58 × 10 −4 C/kg |
Absorbed dose | Amount of radiation absorbed by an object/person | Rad (r) Gray (Gy) | I Gy = 100 r |
Dose equivalent | Amount of radiation absorbed as it relates to the radiation type and subsequent biologic impact | Roentgen-equivalent-man (rem) Sievert (Sv) | 1/1000th rem is known as millirem (mrem); commonly used for biologic dose equivalent 1 Sv = 100 rem |
Fluoroscopy History and Background
Fluoroscopy is an imaging technique that uses real-time x-rays to create images of a patient. The history of fluoroscopy dates back over a century. In 1895, physicist Wilhelm Conrad Roentgen (1845–1923) discovered and coined the term “x-ray.” His discovery was accidental; he noticed a glow when attempting to pass cathode rays through glass. Later, he discovered that x-rays penetrate soft tissue but not metal or bone, and he captured the first x-ray image of his wife’s hand. In 1897, x-rays were used to identify broken bones during the Balkan War. In 1901, Roentgen won the Nobel Prize in Physics for his discovery. Following Roentgen’s work on x-rays in 1895, Thomas Edison’s assistant, glassblower Clarence Dally, worked on development of the Edison x-ray focus tube, resulting in a fluoroscope that produced sharper images than the Roentgen fluoroscope.
Unfortunately, the potential harmful effects of x-rays were identified more slowly. In 1904, Dally died from skin cancer. He is considered the first documented radiation fatality, but it was not until the 1950s that x-rays were determined to carry significant risk. In the subsequent decades, engineering and computing advances made fluoroscopy capable of high-resolution imaging using minimal radiation. Currently, the technique of fluoroscopy is practiced in most hospitals, surgery centers, and outpatient clinics and is readily available.
In general, fluoroscopes are considered fixed (permanent) or mobile (portable). Fixed fluoroscopes are either a vertical fluoroscope or fixed C-arm fluoroscope. Mobile fluoroscopes use a C-arm system on wheels and are commonly implemented in outpatient interventional pain practices. The C-arm system moves the x-ray tube and image intensifier in any axis, thereby allowing image acquisition from nearly any angle.
Two categories of fluoroscopic units are currently available for mainstream medical use, traditional image-intensifier, and flat-panel detector systems. The more traditional image-intensifier television camera-based systems have been in use since the 1950s. The traditional image-intensifier based C-arm fluoroscopy system ( Fig. 3.1 ) consists of the following components (from bottom to top):
- •
X-ray generator and tube: generates x-rays
- •
Collimator: restricts the x-ray field and focuses the beam
- •
Image intensifier: allows visualization of the image in a brightly lit room and reduces the radiation dose when compared with standard screen-film cassette
- •
Input phosphor—cesium iodide: converts x-ray photons to light photons
- •
Photocathode—antimony and alkali metals: converts light photons to electrons
- •
Electrostatic focusing lens (electrode focusing plates): guides electrons to the anode
- •
Accelerating anode: accelerates electrons and gives them electronic gain
- •
Output phosphor—zinc cadmium sulfide: converts electrons back to light photons, creating magnification and a thousand times increase in brightness or intensity
- •
- •
Lens/aperture: focuses the light
- •
Video camera (new technology) or television camera (older technology): displays the image

More recently, flat-panel detector systems have been available and have many advantages, most importantly reduction in radiation dose. Flat-panel digital fluoroscopy, derived from thin film transistor technology, emerged in the 1990s and is replacing the image intensifier and video camera. Thin film transistor technology, best known for its use in modern television, avoids motion or image distortion and improves dynamic range, allowing for improved image quality. , , However, some concerns remain about its potentially poor image quality at low exposure levels, technology replacement costs, and equipment durability. ,
There are significant benefits with flat-panel display (FPD) systems when compared with the image intensifier–based units. Solid-state FPD image receptors generally have better stability, lower radiation dose rates, and improved dynamic range, and they eliminate glare and geometric distortions such as vignetting and defocusing effects. Costs for units appropriate for interventional pain procedures have dramatically reduced, which had been a major limiting factor for prior generations of the technology. Based upon the reduced exposure to radiation for both the patient and physician, this technology should be seriously considered by anyone purchasing new units for implementation. Disadvantages include a different appearance than the traditional image intensified units, and lower spatial resolution with very large or very small field of view (FOV).
Three-dimensional (3D) fluoroscopy, the newest innovation to modern fluoroscopy systems, is rotational 3D imaging in which the gantry of a fluoroscopy system rapidly rotates through 180 degrees while continuously acquiring images. The resultant cine display resembles a volume-rendered computed tomographic image and may be used by physicians to better understand the geometric location of various structures, and precision delivery of biologics into bone, disc, and tissue.
Precautions: As Low As Reasonably Achievable
Fluoroscopic guidance involves channeling beams of high-energy, ionized particles (x-rays) through the human body. The x-rays that do not follow this path are scattered into the surrounding environment and create occupational hazard concerns. , The resultant patient and staff exposure to radiation necessitates safety equipment and guidelines to minimize the risk of skin injury or cancer. ALARA (as low as reasonably achievable) is a radiation reduction concept that should be used and enforced in all fluoroscopic settings. It aims to minimize radiation exposure to patients and staff by implementing guidelines around exposure time, shielding, and distance ( Table 3.2 ).
Important actions for reducing exposure time and risk to patient and provider when using fluoroscopy | |
1. | Obtain adequate and appropriate training |
2. | Wear a dosimeter and know your own dose |
3. | Use personal protective equipment including aprons, thyroid collar, eyewear, and shielding when appropriate |
4. | Use good imaging-chain geometry; do not overuse geometric or electronic magnification |
5. | Use collimation, and always collimate to the area of interest |
6. | Position yourself in a low-scatter area |
7. | Plan the intervention in advance to minimize number of acquired images |
8. | Obese patients will require and receive higher doses, and you will too |
9. | Keep beam-on time to a minimum |
10. | Keep the image intensifier as close to the patient as possible and keep the patient as far from the x-ray tube as possible |
11. | Use last-imaged-stored viewing |
12. | Incorporate variable frame rate pulsed practices rather than continuous or “live” fluoroscopy when possible |
13. | If image quality is not compromised, remove the grid during procedures on small patients or when the image intensifier cannot be placed close to the patient |
14. | A single step away from the patient will decrease provider exposure by a factor of four |
The annual individual radiation dose limits were set in 1990 and reconfirmed in 2007 by the International Commission on Radiological Protection and are currently as follows: 20 mSv (5000 mrem/year) for whole body, 150 mSv for thyroid, and 500 mSv for hands. However, protective equipment is imperfect. It is estimated that approximately 0.6% to 6.8% of x-rays are transmitted through protective aprons. Finally, the x-ray generator should be as far and the image intensifier as close to the patient as possible. The scatter of x-rays is greatest between the patient and the x-ray generator, and positioning the x-ray generator below the patient will concentrate scatter to the operator’s feet rather than head. Placing the x-ray generator twice the distance from the patient reduces the scatter of x-rays by one-fourth. As the demonstrated efficacy and ease of access of fluoroscopy increases, and thus the frequency of fluoroscopic use increases, it is imperative that practitioners prioritize radiation safety. Pain management interventionalists and regenerative specialists must appreciate the unwelcome fallout of improperly managed radiography, develop safety protocols to limit both patient and provider exposure, and follow the principle of ALARA.
Radiation Dose
Most interventional pain medicine procedures performed under fluoroscopic guidance result in total radiation exposure time in seconds, not minutes. Regenerative medicine procedures should follow the benchmark guidelines of standard procedures and the average radiation dose delivered ( Table 3.3 ). Studies show that doses less than 2 Gy should not result in any observable effects over the span of 400 days. Assuming a 0.02 to 0.05 Gy/min skin absorption rate, this would take nearly an hour of constant fluoroscopic guidance to reach. There is contemporary discourse revolving around the wisdom of using peak skin dose or concentration in place of fluoroscopy time as a safety measure. This should be considered, particularly when a patient undergoes multiple consecutive injections, such as is commonly done with prolotherapy or platelet-rich plasma. Furthermore, due to the necessary increase in dose and exposure, obese patients—and those present for their procedure—are at significantly greater risk of skin injury and possibly cancer due to fluoroscopy.
Radiation Dose (mGy) | ||||||||
---|---|---|---|---|---|---|---|---|
Procedure | Number of Studies | Mean Dose | Standard Deviation | 10th Percentile With 95% CI | 25th Percentile With 95% CI | 50th Percentile With 95% CI | 75th Percentile With 95% CI | 95th Percentile With 95% CI |
Transforaminal lumbar | 3590 | 10.4 | 11.9 | 2.0 (1.9–2.2) | 3.5 (3.3–3.6) | 6.7 (6.5–7.0) | 12.5 (11.9–13.1) | 32.4 (30.6–34.2) |
Transforaminal cervical | 157 | 5.6 | 6.5 | 1.0 (0.8–1.1) | 1.8 (1.4–2.2) | 3.3 (3.0–3.7) | 5.7 (3.3–6.5) | 21.8 (18.0–27.6) |
Caudal epidural | 658 | 10.0 | 12.4 | 1.6 (1.3–1.7) | 3.1 (2.8–3.6) | 6.0 (5.5–6.4) | 11.0 (9.6–12.4) | 32.8 (27.3–36.3) |
Facet joint cervical | 62 | 2.6 | 2.8 | 0.5 (0.4–0.8) | 0.7 (0.3–0.9) | 1.5 (0.9–1.7) | 3 (1.3–4) | 8.4 (6.8–12.1) |
Facet joint lumbar | 410 | 6.45 | 6.41 | 1.1 (0.7–1.2) | 2.2 (2.1–2.5) | 4.1 (3.3–4.5) | 8.7 (7.9–10) | 19 (15.2–22.1) |
Interlaminar (translaminar) | 446 | 11.2 | 14.7 | 1.4 (1.2–1.6) | 2.7 (2.2–3.0) | 6.4 (5.8–7.4) | 12.4 (9.3–13.6) | 42.2 (26.7–52.5) |
Radiofrequency denervation (lumbar) | 318 | 4.7 | 6.6 | 0.5 (0.4–0.6) | 1.3 (1.1–1.5) | 2.7 (2.3–3.1) | 6.3 (5.7–7.5) | 15.8 (13.0–20.1) |
Lumbar sympathetic block | 296 | 15.4 | 16.2 | 2.5 (2.0–2.8) | 4.5 (3.1–5.3) | 9.9 (7.9–10.9) | 19.2 (15.3–21.9) | 49.9 (40.1–62.8) |
Medial branch block cervical | 41 | 2.2 | 2.1 | 0.4 (0.2–0.5) | 0.7 (0.3–1) | 1.7 (0.8–2.4) | 2.8 (1.4–3.4) | 6.4 (2.7–8.9) |
Medial branch block lumbar | 169 | 3.4 | 4.4 | 3.9 (3–4.1) | 5.2 (4.6–5.5) | 7.9 (6.8–8.5) | 12.1 (10.6–13.8) | 21.8 (14.8–25.7) |
Sacroiliac joint | 87 | 9.9 | 8.2 | 2.0 (1.4–2.5) | 3.7 (1.9–4.9) | 7.0 (5.9–7.7) | 15.0 (11.8–21.3) | 27.4 (22.6–33.9) |
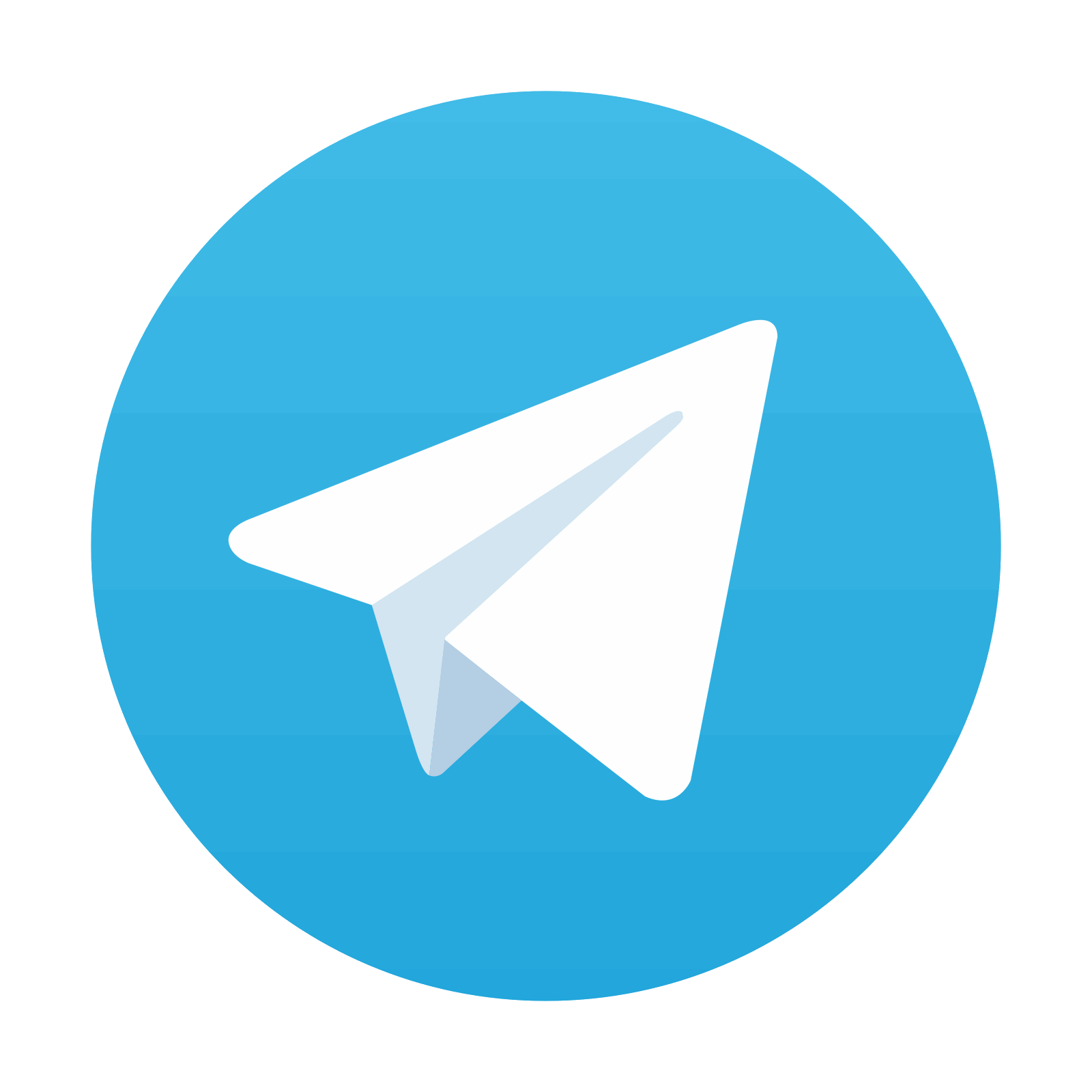
Stay updated, free articles. Join our Telegram channel

Full access? Get Clinical Tree
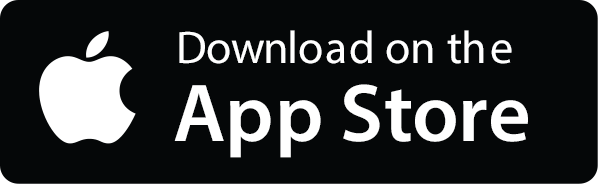
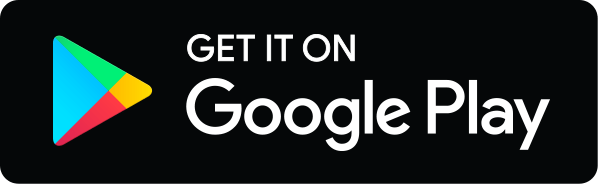
