Platelets are small subcellular fragments that circulate in blood, where they promote hemostasis.
Platelets express receptors and a multitude of mediators recognized as being active in inflammation.
Inhibition of platelet functions generally involves blockade of G protein–coupled receptor soluble agonists (e.g., thromboxane, thrombin, and adenosine diphosphate). However, platelet immunoreceptor tyrosine-based activation motif signaling at the site of inflammation is also implicated.
Platelets likely contribute to inflammation in rheumatic diseases, including rheumatoid arthritis and systemic lupus erythematosus.
Megakaryocytes can act as pro-inflammatory cells independently of platelets and have been implicated in arthritis through animal models.
Blockade of platelet pro-inflammatory functions could represent an approach to treatment of the rheumatic diseases and their associated cardiovascular risks.
Introduction
Platelets patrol the vasculature to promote hemostasis. In blood, platelets represent the second most abundant cellular lineage after the red blood cell, outnumbering leukocytes by several orders of magnitude. When damage to the vasculature occurs, platelets quickly respond to prevent blood loss. In addition to their recognized role in thrombosis, platelets are emerging as important participants in the separation of blood and lymphatic systems, in maintenance of vasculature integrity in inflammation, and in immune responses. Accumulating evidence suggests that platelets and their bioactive mediators are important contributors to the pathogenesis of rheumatic diseases. The source cell for platelets, the megakaryocyte, also has immune sensing and effector capacity and has itself been implicated experimentally in systemic inflammatory disease.
Platelet Structure
Platelets are small subcellular fragments that circulate in blood. In humans, normal platelet counts range between 150 × 10 6 /mL and 450 × 10 6 /mL, making them the second most abundant cell lineage present in blood after erythrocytes. Structurally, resting platelets resemble irregular discs that are 2 to 5 μm in diameter and 0.5 μm thick, with a volume of 6 to 10 femtoliters. In comparison, lymphocytes and neutrophils have a volume of 218 and 330 femtoliters, respectively. , Because of their small size and discoid shape, platelets are continuously pushed by the blood flow toward the edge of blood vessels, positioning them optimally to recognize endothelial injury. Thus because of their structure and abundance in the blood, platelets are positioned to fulfill their hemostatic function: the maintenance of vascular integrity.
The cytoskeleton maintains the platelet’s discoid shape. A spectrin-based cytoskeleton is associated with the cytoplasmic side of the plasma membrane, microtubule coils form along the circumference of the cell, and 2000 to 5000 linear polymers of actin, the most abundant protein expressed by platelets, fill the cytoplasm. These cytoskeletal components maintain the platelet’s structure in the setting of high fluid shear forces and also enable conformational changes with activation to form finger-like filopodia and pseudopods. Under these conditions, the platelet structure is remodeled dramatically, changing from a disk to spiny sphere.
The resting platelet plasma membrane is composed of phospholipids that appear smooth on electron microscopy, with a fine corrugated surface. , The surface membrane contains channels and sinuous invaginations called the open canicular system (OCS). The OCS increases the platelet surface area in direct contact with the extra-cellular milieu. Plasma components such as serotonin and fibrinogen are imported into platelets through this system, and the OCS serves as a conduit for the release of mediators stored within the platelet. , The channels that constitute the OCS are also a source of membrane necessary for the profound morphologic changes, such as the formation of filopodia and spreading that occur rapidly when platelets are activated, increasing their exposed surface by 420%. Adherent platelets trail long (250 μm) tendrils into the blood vessel lumen, further increasing their contact surface and helping them interact with circulating leukocytes.
The platelet cytoplasm includes numerous small organelles with three main types of secretory granules: α-granules, dense bodies (δ-granules), and lysosomes ( Table 17.1 ). The α-granules are the most abundant organelle in platelets (40 to 80 per platelet). , They are round or oval, have a diameter of 200 to 500 nm, and contain proteins in their lumens or membrane, including von Willebrand factor (vWF), P-selectin, coagulation factor V, thrombospondin, fibrinogen, platelet factor-4 (also known as CXCL4), and growth factors such as platelet-derived growth factor (PDGF) and transforming growth factor-β (TGF-β). Dense bodies are smaller than α-granules, are fewer in number (4 to 8 per platelet), and are rich in calcium, magnesium, adenosine diphosphate (ADP), adenosine triphosphate (ATP), serotonin, and histamine. , Calcium and serotonin are responsible for the opacity of dense bodies on electron microscopy. Platelets also usually bear approximately one lysosome (sometimes none and never more than three). These lysosomes may serve as an endosomal digestion compartment, but the importance of this function in hemostasis is unknown. Proteolysis can also take place in platelet proteasomes. The proteasome is necessary for platelet function, because its pharmacological blockade by the proteasome inhibitor bortezomib (given to patients with multiple myeloma) inhibits platelet thrombotic activities and platelet production. , Platelets express residual fragments of Golgi complexes and functional mitochondria (4 to 7 per platelet), which are implicated in the production of energy and platelet activation. ,
Platelet Component | Actions | |
---|---|---|
Surface molecules | P-selectin (CD62P), PECAM (CD31), GPIbα | Adhesive targets for leukocytes |
PAF, ROS | Neutrophil activation | |
CD154 (CD40 ligand) | Agonist for endothelial cells | |
Soluble factors | Serotonin, histamine | Regulators of vascular permeability |
β-Thromboglobulin, PF4 | Chemotaxis | |
Acid hydrolases, ROS | Tissue destruction | |
PDGF, TGF-β | Cellular mitogens, chemoattractant | |
End products of platelet procoagulant activity | Thrombin, fibrin | Promote leukocyte accumulation |
Platelet Production
The great majority of the platelets will never be involved in any hemostatic process and will be eliminated at senescence through the reticuloendothelial system in the liver and spleen. Because of their relatively short life span in circulation (10 days), approximately 100 billion platelets must be produced every day in the human body. Platelets are not cells per se; they are fragments derived from megakaryocytes and, consequently, they are without a nucleus. , The megakaryocyte arises from a master stem cell in the bone marrow under the control of thrombopoietin, a hormone produced in the liver and kidneys that promotes megakaryocyte maturation, number, and size and forestalls megakaryocyte apoptosis. , Thrombopoietin is a soluble, 80 to 90 kDa protein that acts through binding to its receptor, c-Mpl, which is expressed by megakaryocytes and platelets. The binding of thrombopoietin to platelets induces its catabolism. More thrombopoietin is thus available in people with lower platelet counts, thereby driving megakaryocyte activity. However, serum thrombopoietin levels remain normal in severely thrombocytopenic mice, and mice deficient in apoptosis-regulatory molecules (e.g., Bax and Bak) exhibit high levels of thrombopoietin despite the increased platelet counts, thus suggesting that thrombopoietin metabolism by platelets is not the only determinant of platelet biogenesis. This mechanism may involve glycoprotein Ibα (GPIbα), a receptor expressed by platelets, as its ablation in mice or its absence in humans is associated with reduced levels of hepatic thrombopoietin. Further, aging platelets directly induce thrombopoietin expression in hepatocytes because they are cleared through an interaction between desialyated platelet surface glycans and the hepatic asialyloglycoprotein receptor.
Cytokines such as IL-1, IL-3, IL-6, IL-11, stem cell factor (also called Kit ligand), and granulocyte-macrophage colony-stimulating factor (GM-CSF) amplify megakaryocyte production. Enhanced platelet production can be assessed by measurement of the mean platelet volume, a measure that is often part of a complete blood cell count. A larger average platelet size is seen when platelet production by megakaryocytes is increased, generally reflecting compensation for accelerated platelet destruction as seen in immune thrombocytopenic purpura (ITP), myeloproliferative diseases, and Bernard-Soulier syndrome.
The mature megakaryocyte is a giant cell (50 to 100 μm in diameter) resulting from repeated cycles of endomitosis, which means that it undergoes repeated cycles of DNA replication without cell division, leading to polyploidy, typically up to 16N (128N can be observed). This amplification of DNA results in gene amplification and increases protein production, which is necessary for the generation of up to 5000 platelets per megakaryocyte. During platelet formation, long (millimeters in length) cytoplasmic extensions emanate from the megakaryocyte and protrude out of the bone marrow in the interior of vascular sinusoids. These extensions, called proplatelet processes , elongate through the action of microtubules, which also serve as a track for the transportation of membranes, organelles, and granules from the mother megakaryocyte distally into nascent proplatelets. Actin filaments participate in proplatelet branching. Cytoplasmic continuity between the mother megakaryocyte and nascent proplatelets also enables platelets to bear functional microRNA and messenger RNA, as well as the molecular machinery required to translate the latter efficiently into protein. ,
Fragments from proplatelet processes are next released into the blood as either globular pre-platelets or as barbell-shaped proplatelets ( Fig. 17.1 ). These interconvertible forms generate platelets upon fission. Furthermore, individual human platelets can divide. Thus platelet maturation likely continues in the circulation. Interestingly, megakaryocytes can migrate into the bloodstream, and evidence suggests that an appreciable proportion of platelets is generated by the megakaryocytes that have taken up residence in the lungs. , ,

Platelets and Hemostasis
The sum of the processes by which the loss of blood is prevented is called hemostasis . Intravital microscopic analyses, performed as early as 1882 by the Italian scientist Bizzozero, demonstrated that platelets could recognize a damaged blood vessel and form a plug. Since then, the molecular participants in the promotion of hemostasis have been described. The intact endothelium produces molecules, such as prostacyclin (PGI 2 ), nitric oxide, and thrombomodulin, which, conjointly with cell-associated ecto-adenophosphatase (ADPase; CD39), inhibit platelet activation. Endothelial injury triggers vasoconstriction, which reduces blood flow and exposes the subendothelial matrix. At this stage, the main platelet glycoprotein (GP) receptors implicated are the members of the GP Ib-IX-V complex (25,000 copies/platelet), and the integrin αIIbβ3 (GPIIb-IIIa complex; 80,000 copies/platelet). ,
Under high shear, the GPIb-IX-V complex attaches to vWF, which is itself bound to the wound rich in collagen fibers, mediating transient adhesion (also referred to as tethering). Glycoprotein VI (GPVI), which is expressed as a complex with the homodimeric Fc receptor γ chain, , and the integrin α2β1 stabilize the association by binding collagen directly. Although GPIIb-IIIa is a receptor for several ligands (fibronectin, fibrinogen, vWF, thrombospondin, and vitronectin), only its activated form binds fibrinogen. Platelet activation through GPIb-IX and GPVI thus promotes inside-out signaling, leading to GPIIb-IIIa activation and the binding of the latter to fibrinogen, thereby bridging platelets together and recruiting additional platelets onto adhered spread platelets. , , Deficiency of components of the GPIb-IX-V complex and the GPIIb-IIIa complex leads to the congenital bleeding disorders Bernard-Soulier disease and Glanzmann’s thrombasthenia, respectively. , vWF catabolism is mediated by the metalloprotease ADAMTS-13, which is expressed in plasma and acts as a natural anti-thrombotic agent. Mutations of ADAMTS-13 or depletion by autoantibodies cause familial and acquired thrombocytopenic purpura, respectively.
Platelet activation engages intra-cellular signaling. The actin cytoskeleton pushes the granules toward the platelet membrane. Granule fusion with the plasma membrane is mediated by soluble N-ethylmaleimide-sensitive factor attachment protein (SNAP) receptor (SNARE) complexes, leading to release of granule content through the OCS route. The release of ADP further activates platelets through P2Y12 receptors. Because phospholipids serve as a reservoir of fatty acid, such as arachidonic acid (AA), the cleavage of phospholipids by phospholipase A2 generates lysophospholipids, which can be metabolized into platelet activating factor (PAF) and AA. AA is the substrate of cyclo-oxygenase 1 (COX-1) present in platelets, leading to generation of thromboxane A 2 . Thromboxane A 2 is highly potent at inducing platelet aggregation and vasoconstriction, acting via the thromboxane A 2 receptor. As a result, growth of the platelet plug occurs and the blood coagulation cascade is initiated, leading to thrombin generation and fibrin clot formation. In line with the significance of these activation pathways, the inhibition of ADP activity using antagonists of P2Y12 receptors (such as clopidogrel) and of the biosynthesis of thromboxane A 2 using COX-1 inhibitors (such as acetylsalicylic acid) is frequently applied in people at risk of myocardial infarction and thrombotic stroke.
Platelets also respond to activation and shear stress through the release of small extra-cellular vesicles called microparticles or microvesicles. Microparticles, originally termed “platelet dust,” are produced by cytoplasmic blebbing and fission. They measure approximately 100 to 1000 nm in diameter and are distinct from the exosomes that are smaller (50 to 100 nm in diameter) and originate from multivesicular bodies and α-granules through exocytosis. Megakaryocytes can also elaborate microparticles directly, independent of platelet synthesis. Platelets and megakaryocytes are the most abundant source of microparticles in circulating blood, as identified by expression of GPIIb (CD41). During microparticle release, loss of membrane asymmetry generally occurs, leading to exposure of phosphatidylserine (PS) on the microparticle surface. Exposed PS can support blood coagulation. Platelet microparticles are implicated in the pathogenesis of several inflammatory pathologies, including rheumatic diseases (see below).
Beyond their role in the defense of injured blood vessels, platelets appear to play an incompletely understood role in the maintenance of endothelial integrity. Patients with severe thrombocytopenia have been noted to develop abnormalities of the endothelium such as thinning, fenestrations, and enhanced vascular permeability. , Accordingly, animals with profound experimental thrombocytopenia experience bleeding at the site of inflammation in skin, lung, or brain.
Signaling Pathways in Platelet Activation
ADP, thromboxane A 2 , and thrombin, the latter through its binding to protease-activating receptors (PARs), share signaling via G protein–coupled receptors (GPCRs). , However, GPCR signaling is dispensable for the prohemostatic role of platelets in inflammation, pointing to the existence of other significant activation signaling pathways in platelets.
Immunoreceptor tyrosine-based activation motif (ITAM) signaling can play such a role. Platelets express three receptors that belong to the family of ITAM receptors: Fc receptor γIIA (a low affinity receptor for IgG immune complexes), Fc receptor γ chain, which is noncovalently associated with GPVI and is necessary for GPVI function, , and the C-type lectin 2 (CLEC2), a receptor for podoplanin.
Fc receptor γIIA (FcγRIIA) is expressed on human platelets but is absent in mice, , and its activation is critical in the pathogenesis of immune-mediated thrombocytopenia, bacterial sepsis–associated thrombocytopenia, disseminated intravascular coagulation, and various thrombotic manifestations of antiphospholipid antibody syndrome. , Importantly, FcγRIIA also amplifies platelet aggregation and thrombus formation by contributing to integrin outside-in signaling. ,
To determine how platelets prevent hemorrhage during inflammation, transgenic mice expressing a chimeric human IL-4 receptor α/GPIbα (hIL-4Rα/GPIbα) protein instead of GPIbα on the platelet surface were used to induce thrombocytopenia. By infusion of antibodies against hIL-4Rα, profound thrombocytopenia was generated and hemorrhages were detected in the skin after inducing an Arthus reaction. Because wild-type (WT) platelets are insensitive to platelet depletion by anti-IL4Rα (they don’t express the chimeric α/GPIbα [hIL-4Rα/GPIbα] protein), WT platelets were transfused in thrombocytopenic mice and could rescue mice from bleeding at the site of inflammation. Importantly, transfused platelets lacking the expression of GPVI and CLEC2 failed to prevent bleeding in skin during an Arthus reaction, demonstrating the key role of GPVI and CLEC2 signaling in the maintenance of endothelial integrity by platelets.
Another prohemostatic role of platelet ITAMs is the separation of blood and lymphatic vessels. Mouse embryos deficient for CLEC2 display blood-filled lymph sacs and die shortly after birth, reminiscent of lethality seen in mice lacking spleen tyrosine kinase (Syk) and SLP-76, two kinases downstream of CLEC2 signaling. Blood-filled lymphatic vessels also develop in lethally irradiated mature mice transplanted with bone marrow lacking CLEC2, Syk, or SLP-76, and they die of lymphatic dysfunction, demonstrating that CLEC2 and its signaling are implicated in the separation of blood and lymph in the adult. , It is not known whether drugs intended to target Syk in the treatment of chronic inflammatory conditions might have an impact on blood-lymphatic vessel separation.
Similarly to most hematopoietic cells, platelets also express immunoreceptor tyrosine-based inhibition motif (ITIM)-containing receptors. Although ITIM receptors, such as PECAM-1 and G6b-B, are best known for their role in the attenuation of ITAM-mediated platelet activation, studies show that they may also contribute to platelet reactivity or production.
Together, these observations illustrate that not all functions of platelets can be blocked simply through “classic” anti-platelet drugs such as antagonists of ADP, acetylsalicylic acid, and thrombin ( Fig. 17.2 ).

The Platelet as an Inflammatory Cell
Nucleated thrombocytes, called hemocytes , participate both in hemostasis and in immune defense in lower vertebrates (amphibians, birds, fish, and reptiles). It is generally accepted that these more primitive multifunctional cells slowly evolved to the more specialized role of the mammalian platelets. The platelet nevertheless maintains a broad repertoire of inflammatory mediators, and growing evidence points to an active role of the platelet in innate and adaptive immunity.
Inflammation occurs during innate and adaptive immune responses and in tissue repair and can sometime contribute critically to human disease. A central event in inflammation is the recruitment of leukocytes. When a blood vessel is damaged, not only do platelets rapidly seal the vascular leak, but they also recruit leukocytes that serve to combat potential infection at the injured site. Because of their abundance in blood and their adhesive receptors, platelets are perfectly positioned to have an impact on immune cell extravasation. Platelet P-selectin interacts with leukocyte P-selectin glycoprotein ligand-1 (PSGL-1), allowing leukocytes to roll on adherent activated platelets. Firm adhesion of leukocytes follows through the interaction between leukocyte integrin αMβ2 (CD11b/CD18, Mac-1) and platelet GPIb. ,
CD40L (CD154) is a transmembrane protein and is part of the TNF receptor family that is expressed by both T cells and platelets. Its counterligand CD40 is expressed by macrophages, dendritic cells, B cells, platelets themselves, and other lineages, including endothelial cells. Platelet-derived CD40L can support B cell differentiation and immunoglobulin class switching and induces the expression of intercellular adhesion molecule-1 (ICAM-1), vascular cell adhesion molecule-1 (VCAM-1), and release of chemokine (CC motif) ligand 2 (CCL2) by endothelial cells, promoting leukocyte adhesion and extravasation. Because platelets are the largest reservoir of soluble CD40L in the human body, plasma levels of CD40L correlate with platelet activation.
Platelets also express Toll-like receptors (TLRs), which are members of a family of pattern recognition receptors that recognize well-conserved molecular motifs common among pathogens. Platelets express TLRs 1 through 9, and platelet TLR stimulation provokes thrombocytopenia and engages TNF production in vivo. When platelet TLR4 is activated by bacterial lipopolysaccharide (LPS), it promotes the interaction of platelets with neutrophils, helping trigger neutrophil degranulation and release of neutrophil extra-cellular traps (NETs) that stretch downstream of the platelet-neutrophil aggregate in blood. NETs are readily observed in RA, systemic lupus erythematosus (SLE), and gout. Whether platelet-induced NETosis is beneficial or detrimental is unclear, but recent observations in gout suggest that NETs may form aggregates that trap cytokines and thereby limit inflammation.
Apart from their surface receptors, platelet-derived soluble molecules also contribute to inflammation via mediators including growth factors, cytokines, chemokines, and lipid mediators (see Table 17.1 ). Activated platelets release PDGF and TGF-β, which are responsible for the fibroproliferative response in chronic inflammation. PDGF is chemotactic for smooth muscle cells, fibroblasts, and macrophages and is central in wound healing. Platelets represent a major pool of TGF-β in humans (milligrams of TGF-β/kilogram) and regulate its blood levels. TGF-β is also chemoattractant for leukocytes, and its main function appears to be the inhibition of inflammation, because its systemic administration can reduce the development of inflammatory arthritis in rats, and TGF-β is also necessary for the development of CD4 + CD25 + FoxP3 + regulatory T (Treg) cells. However, fine control of its expression is important because TGF-β overexpression is potentially profibrotic.
Other members of the platelet secretome implicated in the intertwined prohemostatic and pro-inflammatory reactions are the CXC chemokine PF4 and the CC chemokine RANTES (regulated upon activation, normal T cell expressed and secreted), stored in α-granules. Because patients who have gray platelet syndrome (and thus are deficient in platelet α-granules) do not experience recurrent infections, platelet pro-inflammatory activity may reside in other storage compartments or may reflect newly synthesized mediators. Indeed, activated platelets can rapidly synthesize and release IL-1β. , In sum, platelets are versatile players in inflammation. The fact that platelets express inflammatory mediators, which appear to be mostly dispensable for their hemostatic functions, supports the idea that they might contribute to inflammatory conditions, such as rheumatic diseases.
The Megakaryocyte as an Inflammatory Cell
Compared with platelets, evidence for a role for megakaryocytes in systemic inflammation is more limited. Like platelets, megakaryocytes can express several members of the TLR family, including TLRs 1, 2, 3, 4, and 6. TLR5 mRNA transcripts are expressed in murine lung megakaryocytes. The functional consequences of activation via these receptors are poorly understood but might include acceleration of megakaryocyte maturation and platelet production. , , Human megakaryocytes, like human platelets, can express the low-affinity IgG receptor FcγRIIA. , Murine studies suggest that stimulation via Fc receptors might enhance release of megakaryocyte microparticles, an observation that reflects stimulation of the high-affinity receptor FcγRI by noncanonical ligands such as C-reactive protein. The IgE receptor FcεRI is also expressed but serves primarily to pass this receptor to platelets, because human platelets but not megakaryocytes express this receptor at the cell surface. The role of these receptors in megakaryocyte function in vivo has not been explored.
Megakaryocytes might also contribute to adaptive immunity. Like platelets, megakaryocytes express CD40L. In the marrow environment, megakaryocytes promote plasma cell survival, potentially via IL-6 and a proliferation-inducing ligand (APRIL). Megakaryocytes can take up exogenous antigen and cross-present this in the context of class I major histocompatibility complex (MHC), enabling immune thrombocytopenia in a murine experimental model.
Megakaryocytes can elaborate a range of distinct cytokines, chemokines, and other mediators. The bone marrow niche is characterized by high concentrations of PF4 and TGF-β of megakaryocyte origin that participate in the regulation of hematopoietic stem cells. Expression of the neutrophil chemoattractants CXCL1 and CXCL2 (corresponding to human IL-8) enable megakaryocytes to regulate neutrophil egress from bone marrow. In fact, granulocyte colony-stimulating factor (G-CSF)–mediated release of murine marrow neutrophils is mediated by megakaryocytes that release CXCL1/2 in response to thrombopoietin generated by G-CSF–sensing cells in the marrow stroma; correspondingly, administration of thrombopoietin can cause an increase in circulating neutrophil count.
Megakaryocytes are also able to produce IL-1α and IL-1β as free cytokine or packaged within microparticles. , , Correspondingly, engraftment of WT but not IL-1–deficient megakaryocytes restores the susceptibility of certain disease-resistant mice to arthritis, even independent of their platelets, establishing a potential role for megakaryocytes in IL-1–driven systemic inflammatory disease.
A final, intriguing interaction of megakaryocytes in immunity is termed emperipolesis . This term, derived from the Greek em “inside,” peri “around,” and polesis “wandering,” refers to the observation that leukocytes can be seen moving about within megakaryocytes in fresh marrow specimens. Marrow histologic sections show active emperipolesis in 2% to 5% of megakaryocytes in bone marrow sections, both human and murine, with neutrophils represented disproportionately. Emperipolesis increases markedly under pathologic conditions, including hematologic malignancy and myelofibrosis. Recent development of an in vitro model of emperipolesis, together with ultrastructural imaging and in vivo murine experiments, have established that emperipolesis represents a novel pathway for the exchange of membrane and other material between megakaryocytes and neutrophils, which emerge viable from the interaction. The effect of this exchange on the function of megakaryocytes, platelets, and neutrophils remains to be established ( Fig. 17.3 ).

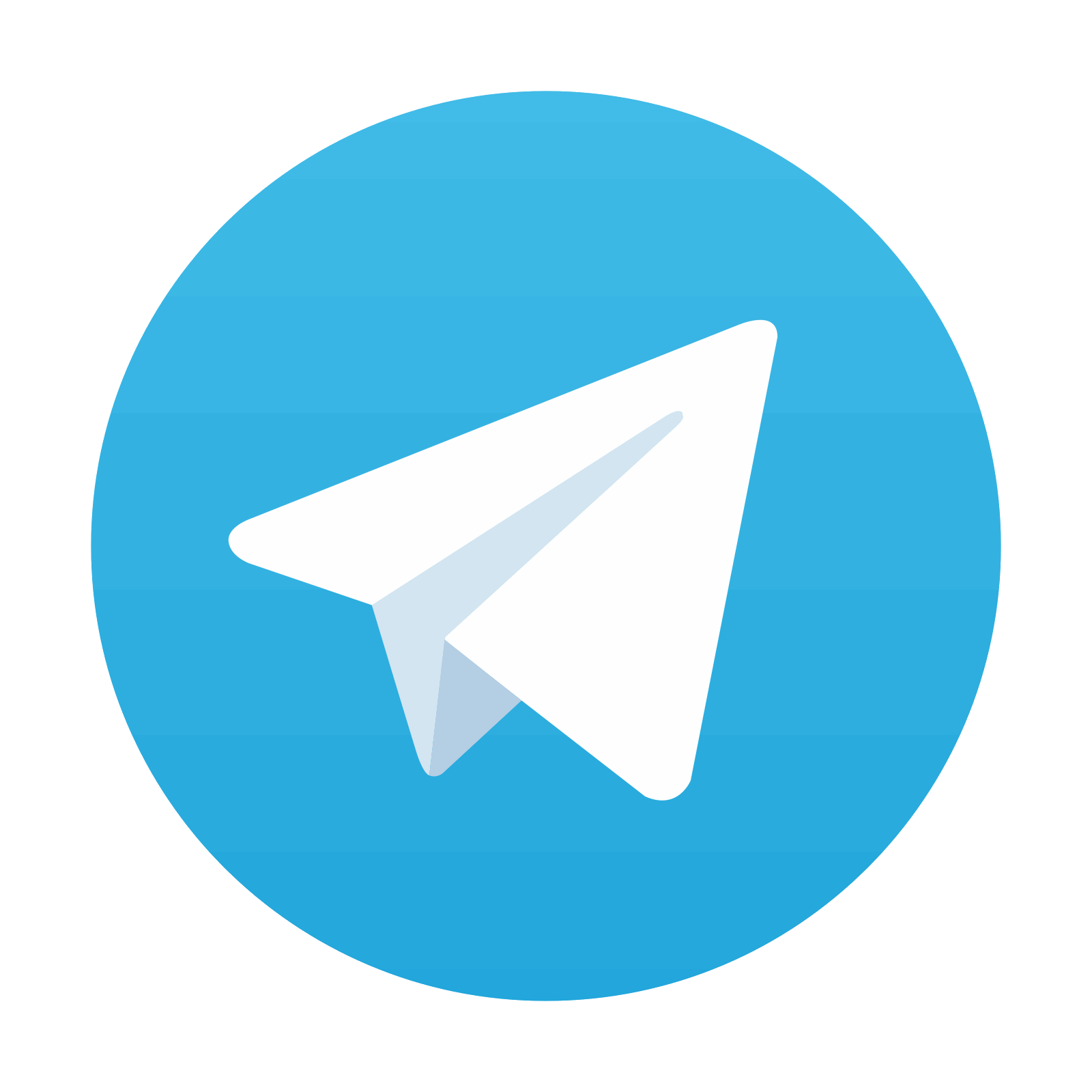
Stay updated, free articles. Join our Telegram channel

Full access? Get Clinical Tree
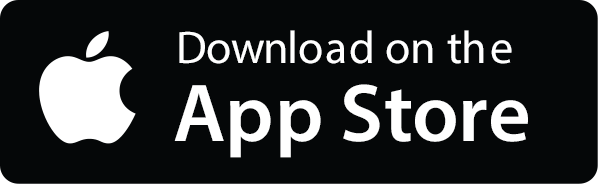
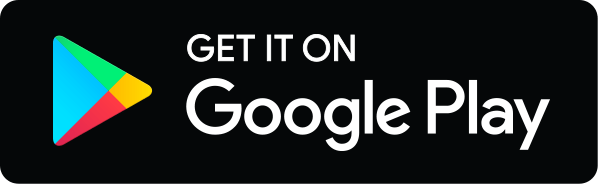
