Additional videos related to the subject of this chapter are available from the Medizinische Hochschule Hannover collection. The following video is included with this chapter and may be viewed at https://expertconsult.inkling.com :
- 69-1.
Retrograde locked rodding for periprosthetic fractures of the distal femur after total knee replacement.
Introduction
Periprosthetic fractures of the lower extremity are commonly encountered in clinical practice and can be extremely challenging for the surgeon to manage. Because of an increasingly elderly and demanding population, greater numbers of total hip arthroplasty (THA) and total knee arthroplasty (TKA) procedures are being performed. In the last decade, the frequency of THA has increased by more than 25% whereas the rate of increase was even higher for TKA. This reflects not only the successful nature of lower limb arthroplasty but is also indicative of higher standards of living, higher patient expectations of surgery, and advances in implant technology. Consequently, complications specific to these operations are also on the rise. Periprosthetic fractures occurring around THA and TKA implants can arise either intraoperatively because of a variety of surgical factors or postoperatively usually following low-energy trauma.
Numerous epidemiologic studies have been performed regarding periprosthetic femoral fractures. Concerning intraoperative fractures, Berry reported an incidence of 0.3% in primary cemented and 5.4% in uncemented THAs from the Mayo Clinic joint registry. In the revision setting, higher rates of fracture were seen with a rate of 3.6% in cemented and 20.9% in uncemented THAs. In 2011, the U.K. National Joint Registry reported that intraoperative femoral shaft fractures were seen in 0.05% of 71,672 primary THAs and trochanteric fractures occurred in 0.2%. An analysis of 1049 postoperative fractures reported in the Swedish joint registry between 1997 and 2000 by Lindahl and colleagues revealed an annual incidence of 0.045% to 0.13% with a tendency to increase over time. Furthermore, they reported that the mean time to fracture was 7.4 years following primary THA and 3.9 years following revision THA. Periprosthetic fractures of the acetabulum are less common and are usually sustained intraoperatively. A review of 7121 primary THAs performed at the Mayo Clinic revealed an incidence of 0.29%, all of which were sustained during the insertion of uncemented acetabular components.
Periprosthetic fractures around TKA are less common and therefore, there are less epidemiologic data available. However, this is still a frequent indication for revision surgery following TKA. A review of 1000 cemented TKAs at 15-year follow-up by Vessely and colleagues revealed that 22% of all reoperations were caused by periprosthetic fracture. Intraoperative fractures are uncommon and according to the U.K. National Joint Registry data from 2011, they were seen in 0.15% of 79,516 primary TKAs. The overall incidence of postoperative fracture has been quoted as 0.3% to 5.5% for primary TKA and up to 30% for revision TKA. Supracondylar femoral fractures are most commonly encountered and have an estimated incidence of up to 2% after primary TKA. Patella fractures have been reported as having an incidence of 0.68% and tibial fractures are estimated to occur in 0.39% following primary TKA according to Mayo Clinic joint registry data.
The management of periprosthetic fractures is often difficult and surgical intervention involves the skills of fracture fixation as well as revision arthroplasty and is, therefore, best performed by an orthopaedic surgeon with the appropriate expertise. Nevertheless, it is important for all orthopaedic surgeons to have general understanding of the basic principles underlying these complex fractures. Hence, the purpose of this chapter is to discuss the evaluation and management of patients with periprosthetic fractures occurring around total hip and knee arthroplasty.
Risk Factors
Periprosthetic Fractures around Total Hip Arthroplasty
Intraoperative fractures sustained during THA can affect either the acetabulum or femur and can be a devastating complication resulting in increased morbidity to the patient. The risk factors can be considered in terms of the patient, surgical technique, and choice of implant. Female gender, increasing age, an index diagnosis of hip dysplasia, rheumatoid arthritis, Paget disease, osteoporosis, and chronic steroid use have all been shown to have a higher risk of fracture. Recently, much attention has been given to minimally invasive surgical approaches in order to improve postoperative pain relief and rehabilitation but this has been shown to have an increased rate of periprosthetic fracture when compared to conventional techniques. Furthermore, a higher rate of calcar fracture has been reported with toothed calcar mills highlighting the need for meticulous surgical technique during canal preparation.
The correlation between the use of uncemented implants and intraoperative fracture of both acetabulum and femur is well documented. During impaction of press-fit implants, hoop stresses are transmitted to host bone with the potential of cortical injury. In a cadaveric study, Kim and colleagues reported that excessive under-reaming of the acetabulum can predispose to fracture and suggested that the last reamer should be no more than 1 to 2 mm smaller than the final component, although manufacturers’ operative guides should always be consulted for the appropriate surgical technique. Uncemented femoral stems rely on either a wedge fit with a tapered prosthesis, a tight proximal fill with a metaphyseal filling component, or a scratch fit with an extensively coated stem, and heavy impaction with each design may result in fracture. An analysis of uncemented stem insertion performed by Sakai and colleagues showed through finite element analysis that a decrease in hammering sound frequency was associated with an increase in the estimated maximum stress. Based on these observations, they recommended that when a sudden drop in frequency is heard, the hammering force should be reduced in order to prevent fracture.
Postoperative THA fractures are usually the result of a low-energy fall but may also occur spontaneously as a result of severe osteolysis or in the presence of a stress riser from an adjacent implant. In a biomechanical study of cadaveric femora, Rupprecht and colleagues coupled certain fracture patterns with mechanism of injury. They confirmed that a femoral implant significantly reduced femoral fracture strength and that torsional forces caused intertrochanteric fractures, anterior loading caused supracondylar fractures, and lateral loading caused fractures at the tip of the stem. Patient-dependent risk factors include female gender, increasing age, high body mass index, osteoporosis, and an index diagnosis of rheumatoid arthritis or hip fracture. In addition, polished tapered cemented stems have been implicated in late fracture when compared to matte-finished, anatomically shaped cemented stems in Swedish registry data as a potential result of compressive hoop stresses being transmitted to the cement mantle and cortical bone. However, a subsequent study of a variety of cemented implants in 6458 patients over a 17-year period failed to confirm these findings. This study also reported that the incidence of fracture was 0.8% at 5 years and 3.5% at 10 years after primary THA and that patients older than 70 years had a 2.9 times greater risk of sustaining a periprosthetic fracture. In a comparison of 244 cemented and uncemented hemiarthroplasties performed for trauma over a 2-year period, Foster and colleagues found a 7% incidence of periprosthetic fracture in the uncemented group and none were seen in the cemented group. These findings suggest that in elderly patients, cementation may be preventive against fracture.
Sequelae of primary THA that can predispose to periprosthetic fracture include aseptic loosening and osteolysis. From analysis of Swedish joint registry data, Lindahl and colleagues found that 70% of periprosthetic femoral fractures occurred in the presence of implant loosening. In addition, Lewallen and Berry reported a large series of patients with periprosthetic femoral fractures of which 94% had a radiographically loose component prior to fracture. In a recent in vitro biomechanical study, Harris and colleagues found that in both synthetic and cadaveric femora, loose implants were associated with fracture at lower torque strength than well-fixed implants. Furthermore, proximal spiral fracture patterns were seen with loose stems and more distal fracture patterns with well-fixed stems.
Periprosthetic Fractures Around Total Knee Arthroplasty
Intraoperative fractures that occur during TKA may occur at the femur, tibia, or patella. Alden and colleagues reported 67 fractures in a series of 17,389 primary TKAs of which 64 were identified at the time of surgery. Fractures occurred more commonly in females (80.6%) and in the femur (73.1%). Of the femoral fractures, the medial femoral condyle (40%) was most commonly involved and of the tibial fractures, the lateral tibial plateau (33%) and anterior cortex (22%) were most frequently involved. Twenty-six fractures occurred during exposure and preparation, 22 while trialing, 13 during cementation, and 3 while inserting the polyethylene spacer. Newer implants, such as uncemented press-fit stems, have been implicated as a risk factor and a recent review of 420 consecutive TKAs revealed an incidence of 3%. The risk of patella fracture is lower intraoperatively than postoperatively, but predisposing factors include revision surgery, over-reaming of the patella, aggressive resection leaving less than 10 mm, overstuffing the joint, use of central-peg designs, posterior-stabilized TKA, excessive clamping, and thermal injury from the polymerization of bone cement.
A recent Mayo Clinic joint registry analysis of 17,633 primary TKAs showed that only age younger than 60 years was a significant risk factor for postoperative periprosthetic fracture. This may be because of the fact that younger patients have increased levels of physical activity putting them at greater risk of injury. Also, younger patients who have TKA are more likely to be on chronic steroid medication because of preexisting rheumatologic disease. Furthermore, analysis of 4090 revision TKAs revealed that previous nonunion, infection, and previous revision arthroplasty surgery were significant risk factors for fracture. Although most postoperative fractures occur because of trauma, there are also associations with preexisting conditions, such as osteoporosis, chronic steroid use, rheumatoid arthritis, epilepsy, parkinsonism, myasthenia gravis, poliomyelitis, cerebral palsy, tibial deformity, and infection. Surgical risk factors include component malpositioning, stress shielding, inadequate reaming, box cuts for posterior-stabilized implants, excessive resection, malalignment, patella maltracking, tibial intramedullary referencing, and osteolysis. Notching of the anterior femoral cortex has been the subject of much debate as it has been shown in biomechanical studies that anterior femoral notches greater than 3 to 5 mm produce stress risers that may lead to periprosthetic fracture. However, large clinical series have failed to find any correlation between notching and supracondylar periprosthetic fracture. Postoperative patella fractures have been shown to have an association with previous knee surgery, greater preoperative malalignment, thinner postresection patellar thickness, and patella baja. Also, performing a lateral release in combination with a medial parapatellar approach may lead to avascular necrosis of the patella and may eventually manifest as a pathologic fracture.
Evaluation
Examination
All patients presenting with periprosthetic fractures of the lower extremity require a detailed medical evaluation, particularly as they may be elderly with complex medical comorbidities. A thorough history should be taken to include age, occupation, details of the mechanism of injury, social and functional status, past medical history, and medication history. Particular attention must be paid to the affected limb in terms of indication for primary arthroplasty and any associated postoperative complications, for example, pain, infection, and instability. As osteolysis can predispose to fracture, careful questioning about pain prior to the injury must be performed as this will help direct management. Previous medical notes should be retrieved and carefully reviewed in order to determine the exact timing and nature of the primary arthroplasty procedure. Details of the implant should be obtained, including manufacturer, model, and size as this will enable the desired extraction kit to be ordered if required for revision surgery. A thorough clinical assessment should be performed concentrating primarily on initial resuscitation maneuvers that may be required due to blood loss and hemodynamic instability. Any other life-threatening injuries should be dealt with as a priority in the case of multiply injured patients or those involved in high-energy trauma. A complete neurovascular examination should be performed and documented accurately.
Investigations
Once adequate analgesia has been administered, a full radiographic and laboratory workup is required in order to obtain a definitive diagnosis and prepare the patient for any potential surgical intervention. Initially, a full set of hematologic investigations should be performed to include a full blood count, urea and electrolytes, clotting screen, blood group screening and crossmatching if transfusion is required or for presurgical workup. Urinalysis should be performed to rule out a urinary tract infection, which may need treating prior to surgery. A radiograph of the affected limb to include the entire femur or tibia in two orthogonal planes must be performed in order to obtain an accurate diagnosis. Detection of implant loosening can be confirmed by comparing with previous radiographs where possible. In cases where this reveals osteolysis, an infection screen must also be performed to include inflammatory markers and blood cultures. However, it must be noted that inflammatory markers (i.e., erythrocyte sedimentation rate and C-reactive protein) may be slightly elevated as a consequence of trauma and may be a poor indicator for infection. A review of 206 consecutive patients with periprosthetic hip fractures revealed that increased inflammatory markers correlated poorly with positive aspirate samples for deep periprosthetic infection. For the patients with negative microbiologic results from aspiration, 43.5% had an elevated C-reactive protein level, 30.6% had an elevated erythrocyte sedimentation rate, and 15.1% had an elevated white blood cell count. Therefore, if infection is suspected on clinical grounds, then further investigations should be performed, for example, joint aspiration, bone scan, or computed tomography (CT) scan. Further imaging can also be extremely useful in outlining the location and extent of bone loss when planning surgical fixation or revision. Importantly, if calf pain is reported by the patient, a duplex ultrasound scan may also be warranted to investigate the presence of deep vein thrombosis (DVT), which will need medical treatment prior to surgery. Where DVT is present in a high-risk patient, consideration should be given to insertion of vena cava filter prior to surgery to minimize the risk of pulmonary embolism.
Periprosthetic Fractures Around Total Hip Arthroplasty
Classification
Periprosthetic Fractures of the Acetabulum
Although uncommon, periprosthetic fractures of the acetabulum are usually iatrogenic injuries caused either by component insertion during primary THA or component removal at revision THA. Recognizing intraoperative fractures is extremely important, as any defect in the acetabulum may contribute to instability and failure of the component, particularly when using press-fit uncemented implants. Although this can be difficult, a high index of suspicion should be maintained and the use of intraoperative radiographs to include obturator and iliac oblique views may be helpful. Postoperative fractures usually occur secondary to osteolysis rather than trauma and may be regarded as pathologic. Adequate imaging is essential in determining the fracture type and presence of osteolysis. Miller reported a consecutive series of nine patients with a postoperative fracture of the acetabulum occurring between 14 and 48 months following THA of which only one was caused by direct trauma.
Numerous classification systems have been proposed based on implant stability, fracture pattern, and time to presentation. However, the most comprehensive classification system is that of Della Valle and colleagues, which is organized by clinical presentation and also guides treatment ( Table 69-1 ). Type I fractures are fractures recognized intraoperatively and therefore, meticulous evaluation of fracture displacement and implant stability is critical in order to determine whether component exchange or internal fixation is required. Type II fractures occur during component removal and an assessment of the remaining bone stock guides selection of the revision component. Type III fractures are caused by postoperative traumatic injury and are further classified according to component stability. Type IV fractures occur without a history of trauma and may be due to osteolysis with or without implant loosening. Infection must be considered as well as other osteolytic lesions. Paprosky and Sekundiak described specific radiographic markers for severe bone loss to include superior component migration of more than 2 cm (loss of superior structural support), ischial lysis (loss of posterior column support), destruction of the teardrop (loss of the inferior part of the anterior column), and a break in Kohler’s line (anterior column deficiency). Type V fractures constitute the complex problem of pelvic discontinuity with varying degrees of osteolysis or previous exposure to irradiation therapy and require careful consideration of reconstruction options.
Type | Description | Subtypes |
---|---|---|
I | Intraoperative fracture secondary to acetabular component insertion |
|
II | Intraoperative fracture secondary to acetabular component removal |
|
III | Traumatic fracture |
|
IV | Spontaneous fracture |
|
V | Pelvic discontinuity |
|
Periprosthetic Fractures of the Femur
Periprosthetic fractures of the femur are much more common and many classification systems have been described in the past. However, the most universally accepted system is the Vancouver classification devised by Duncan and Masri, which is based on fracture site, implant stability, and surrounding bone stock. This was initially devised for postoperative fractures and its high interobserver and intraobserver reliability has been well documented in numerous validation studies. The authors have modified this system to classify intraoperative periprosthetic fractures of the femur to include cortical perforations, longitudinal cracks, or displaced fractures with varying degrees of comminution. Iatrogenic fractures may occur during insertion of uncemented stems and the sound of a crack or sudden change in resistance during implantation warrants the use of intraoperative radiography to confirm the fracture. The Vancouver classification of intraoperative femoral fractures ( Fig. 69-1 and Table 69-2 ) describes type A fractures as affecting the proximal metaphysis, type B fractures concerning the diaphysis, and type C fractures as distal injuries extending beyond the tip of the stem. Each category is further subdivided into type 1, which is a simple cortical perforation; type 2, which is an undisplaced linear crack; and type 3, which represents a displaced or unstable fracture.

Type | Description | Subtypes |
---|---|---|
A | Fractures affecting proximal metaphysis |
|
B | Fractures affecting diaphysis |
|
C | Fractures occurring distal to the femoral stem |
|
The Vancouver classification of postoperative femoral fractures ( Fig. 69-2 and Table 69-3 ) describes type A fractures as involving the trochanteric area and this is further divided into type A G affecting the greater trochanter and type A L affecting the lesser trochanter. Type A G fractures are usually stable whereas large displaced type A L fractures may compromise implant stability through loss of support from the medial buttress. Type B fractures involve fractures around or just distal to the femoral stem and are further divided into type B1 in which the femoral component is well-fixed and stable, type B2 in which the femoral component is loose and unstable, and type B3 in which the femoral component is loose and in the presence of severe bone loss (e.g., due to osteolysis, osteopenia, or comminution). Type C fractures occur distal and well away from the tip of the stem and do not usually interfere with the femoral implant. Although simple and reproducible, there are some limitations of this classification system in clinical practice. It is often difficult to subclassify type B fractures based on the initial presentation. Plain radiographs may not be enough to help appreciate the level of bone loss so further imaging with CT scans may be warranted. Also, implant stability may only best be appreciated during the time of surgery unless the patient reports preexisting problems with their THA in which case a higher index of suspicion may be aroused. Furthermore, there has been a recent report of a unique fracture pattern associated with a polished tapered collarless cemented femoral stem design in which 14 patients with type B2 fractures were noted to have common radiologic and intraoperative findings. The authors described a spiral fracture pattern with extensive fragmentation of bone and cement, debonding of cement from the implant, and cement fracture in the presence of a well-fixed cement–bone interface. Consequently, they urged caution that these fractures could be misinterpreted as type B1 fractures and treated inappropriately.

Type | Description | Subtypes |
---|---|---|
A | Fractures affecting trochanteric area |
|
B | Fractures affecting diaphysis |
|
C | Fractures occurring distal to the femoral stem | N/A |
Management
Periprosthetic Fractures of the Acetabulum
Intraoperative Fractures
The aims of treatment include stabilizing the fracture, achieving bony union, maintaining component orientation and stability, and preserving hip function. Intraoperative fractures may be difficult to recognize but the use of fluoroscopy can help define the extent of the fracture and associated displacement. Type I fractures caused by component insertion may result in instability of the acetabular implant leading to early failure. Undisplaced acetabular wall fractures with a stable component (type IA) can be treated with supplementary acetabular component screws and a postoperative period of restricted weight-bearing for 6 to 12 weeks. Type IB fractures are often caused by column injuries, which render the acetabular component unstable. Therefore, removal of the implant is required to fully determine the extent of the fracture before anatomic reduction and rigid internal fixation is performed ( Fig. 69-3 ). Following this, a line-to-line fit acetabular component should be inserted with supplementary screws for added stability. Again, a period of restricted weight-bearing is advised postoperatively. Type IC fractures are more difficult to treat because they are recognized late and are associated with high risk of failure. Sharkey and colleagues reported a series of 13 patients in which intraoperative acetabular fracture was sustained during insertion of an uncemented cup. Nine of these were noticed intraoperatively and were treated by either additional screw stabilization, autologous bone grafting, restricted weight-bearing, or with no additional treatment if the fractures were deemed to be stable and undisplaced. Although all of these fractures united, two of the four fractures noticed on postoperative radiographs were associated with loosening and required revision arthroplasty. Type II fractures occurring during revision surgery may be associated with varying degrees of bone loss. If there is less than 50% loss of bone stock (type IIA), then a porous-coated hemispherical acetabular component can be used to good effect after the fracture has been reduced and stabilized with internal fixation. Contained defects can be filled with morselized bone graft, whereas larger defects may require structural grafts in order to provide stability for the acetabular component. However, more severe cases of bone loss (type IIB) will require additional mechanical support through the use of specific reconstruction devices, for example, antiprotrusio cages or biologic metal constructs.

Postoperative Fractures
Postoperative periprosthetic fractures of the acetabulum are less commonly encountered in clinical practice but may be due to trauma, that is, type III fractures. The management of these injuries is largely dictated by implant stability. Patients with stable components (type IIIA) can be managed nonoperatively with a period of restricted weight-bearing until union is achieved. However, those with unstable components (type IIIB) require revision surgery combined with fracture fixation. Type IV fractures arise as a result of severe osteolysis and the principles for management are analogous to revision acetabular surgery in the presence of severe bone loss. In addition to reconstituting bone stock with suitable graft material and selecting an adequate revision implant (e.g., cup-cage construct) the fracture must also be held in rigid internal fixation for optimal results. Pelvic discontinuity occurs when there is loss of bone in both the anterior and posterior columns of the acetabulum that disrupts the continuity of the superior and inferior parts of the pelvis. This may occur because of trauma and is associated with varying degrees of bone loss (type V). These fractures present a particularly difficult challenge and require complex forms of reconstruction surgery, which are often associated with high complication rates. However, newer techniques are being reported with promising early results. Sporer and colleagues reported on a series of patients with chronic pelvic discontinuity treated with a tantalum metal elliptical acetabular component using an acetabular distraction technique. Of the 20 patients available for review after a minimum of 2 years’ follow-up, only one required revision for aseptic loosening.
Fortunately most postoperative periprosthetic acetabular fractures are minimally displaced and respond well to nonoperative treatment. Peterson and Lewallen reported on 11 patients who sustained fractures at a mean of 6.2 years after primary THA. Eight of these were deemed to be stable and were managed nonoperatively, one patient died from an associated intrapelvic vascular injury, and two patients with component instability were managed with revision surgery. Despite the majority of the nonoperatively treated fractures uniting, 80% of patients eventually needed revision surgery highlighting the importance for long-term surveillance despite successful initial treatment.
Periprosthetic Fractures of the Femur
Intraoperative Fractures
Intraoperative fractures of the femur can be prevented by paying close attention to surgical technique. Sufficient exposure must be provided by an adequate skin incision especially in patients with risk factors for fracture. An in situ femoral neck cut can prevent fracture in those with acetabular protrusio and excessive torsional movements of the femur must be avoided during canal preparation and reduction maneuvers. It is recommended that broaching should be performed gently and forceful impaction be avoided. Any sudden change in resistance or sudden cracks during broach or stem insertion should warrant urgent fluoroscopy particularly in bone compromised by osteolysis. Generally, the management of intraoperative fractures of the femur depends on their location and degree of displacement.
Undisplaced type A1 fractures are cortical perforations affecting the trochanteric region and are usually stable. They can, therefore, be treated with packed bone graft obtained from acetabular reamings and a period of restricted weight-bearing. Type A2 fractures are undisplaced but require reinforcement with cerclage fixation to prevent propagation of the fracture, implant failure, decreased abductor muscle function, and dislocation. Type A3 fractures are displaced and therefore, need reduction and internal fixation to achieve union and implant stability. This can be achieved through the use of cerclage wires, cables, or trochanteric claw plates prior to final stem insertion. Cerclage cables have been shown to provide stronger fixation than stainless steel wires and result in lower rates of nonunion and trochanteric migration although potential complications include debris generation and third-body polyethylene wear. If the initial implant is a proximal metaphyseal press-fit design, then it should be changed to diaphyseal fitting stem in order to bypass the fracture site and allow adequate fixation.
Cortical perforations of the diaphysis (type B1) often occur during revision surgery at the time of cement removal and may act as a stress riser. Therefore, the revision stem should be long enough to bypass the perforation by at least two cortical diameters. Prophylactic cerclage fixation above and below the defect can prevent fracture propagation and is advised in those with poor bone quality. Furthermore, a cortical strut allograft can be used to supplement fixation particularly in cases where the longest available stem will not adequately bypass the defect. Type B2 fractures are undisplaced linear cracks usually caused by hoop stresses during broach or stem insertion. Often detected at postoperative review, the treatment depends on implant stability. If the implant is stable then a period of protected weight-bearing can be commenced, but if the implant shows signs of migration then operative intervention with cerclage fixation is indicated with revision to a long-stemmed implant. If the revision stem is insufficiently long, then a cortical strut with plate fixation provides adequate stability and high rates of union. Type B3 fractures are displaced and may occur during dislocation of the hip, cement removal, canal preparation, or during broach or stem insertion. The management of these fractures involves open reduction and internal fixation along with revision to a long-stemmed implant. Oblique or spiral fractures can be treated with cerclage fixation whereas transverse fractures are more unstable and require additional support through the use of cortical strut grafts.
Cortical perforations distal to the tip of the stem (type C1) are uncommon but may arise following removal of cement or canal preparation. Morselized bone graft should be used to pack the defect along with a cortical strut graft to prevent a stress riser. Type C2 fractures are undisplaced but require stabilization to prevent fracture propagation. If recognized at the time of surgery, then internal fixation with either cerclage cables or a locking plate should be performed. Again, this should be supplemented with a cortical strut graft in the presence of poor bone quality. Type C3 fractures are displaced and require reduction and fixation using the same principles.
Postoperative Fractures
Postoperative femoral fractures usually tend to occur in elderly patients after low-energy falls and emergent management should be directed toward analgesia, fluid resuscitation, splinting the affected limb in traction, and optimization of coexisting medical comorbidities. Skin traction is usually sufficient but if skeletal traction is deemed necessary then the traction pin should be placed in the tibia so as not to interfere with any potential surgical fixation. The majority of patients will require surgery and therefore, a full preoperative assessment must be performed. Only those with undisplaced and stable fracture patterns or those whose medical history deems them unfit for surgery can be treated nonoperatively. This will entail either a period of restricted weight-bearing or bed rest in traction although the former option is preferred as there are serious risks associated with prolonged bed rest, for example, death, chest infection, and pressure sores. Involvement of a multidisciplinary team, including physicians, physical therapists, and occupational therapists is mandatory in order to allow medical optimization, mobilization, and safe discharge. Follow-up radiographs should be performed on a regular basis to enable an assessment of fracture union and implant stability.
Initially, periprosthetic femoral fractures should be classified according to the Vancouver classification in order to guide surgical management. Type A fractures affecting either the greater or lesser trochanters are usually minimally displaced avulsion fractures and are regarded as stable ( Fig. 69-4 ). Pritchett reported on a series of 30 patients who suffered a postoperative fracture of the greater trochanter and noted that displacement of 2 cm or more was associated with persistent pain or limp at 1 year. Therefore, fractures with less than 2 cm displacement can be treated nonoperatively with protected weight-bearing for 6 to 12 weeks followed by a progressive return to normal weight-bearing. However, occasionally these fractures are more displaced or extend into the calcar region with the potential to affect implant stability and must therefore be treated with internal fixation ( Fig. 69-5 ). Displaced type A G fractures may also result in poor abductor function and may lead to abnormal gait patterns. Type A L fractures are less common but if displaced result in a loss of medial support to the proximal femur further compromising implant stability and again, require operative stabilization ( Fig. 69-6 ). In order to prevent implant failure, it is generally recommended to reduce and stabilize displaced trochanter fragments with either cerclage fixation or a trochanteric claw plate. The use of morselized allograft can help restore bone stock as the presence of osteolysis secondary to stress shielding is a common finding. Hsieh and colleagues reported a series of 887 uncemented THAs in which 23 patients (2.6%) sustained a postoperative type A G fracture by 14 years. All were noted to have occurred through an area of osteolysis. Conservative treatment was employed in 17 patients of which only two failed to unite (11.7%) by 8 weeks. Four patients were treated surgically and all fractures united successfully. However, by latest follow-up, 16 hips (69.6%) had required revision THA because of aseptic loosening. The authors concluded that these fractures should be considered pathologic as they occur in the presence of osteolysis secondary to aseptic loosening and ultimately lead to implant failure.



Type B1 fractures occur around or just distal to the tip of a well-fixed femoral implant and can be successfully treated with open reduction and internal fixation ( Fig. 69-7 ). The aims of surgery include adequate fracture reduction, achieving bony union, maintaining implant stability, and allowing early mobilization. Particular attention must be given to the fracture pattern as this will dictate the method of fixation. Long oblique or spiral fracture patterns can be treated with plate and cerclage fixation, but short oblique or transverse fractures are more unstable and therefore, require biplanar fixation with a combination of plates and/or cortical strut grafts. Furthermore, there is some evidence that treating transverse fractures with stem revision can improve union rates when compared to internal fixation. Cortical strut grafts can be shaped with a burr to allow close cortical contact with the femoral shaft and provide further stabilization as well as increasing femoral bone stock. These are especially indicated in the presence of osteolysis or poor bone quality. The importance of accurately excluding the presence of an unstable stem in these fractures cannot be overemphasized. If any doubt exists, then further imaging is essential, otherwise last-minute adjustments to the surgical plan will have to be made intraoperatively if a stem is unexpectedly found to be loose. Open reduction and internal fixation has previously been shown to have a 34% failure rate because of the misdiagnosis of a type B1 fracture in the presence of an unstable femoral component. Corten and colleagues reported a technique of hip dislocation prior to performing a stem stability test and found that 9 of 45 (20%) type B1 fractures actually occurred around loose stems.

Modern periprosthetic femoral plating systems have rapidly evolved and are now anatomically contoured to the femoral shaft and provide the option for both locking screws and cerclage fixation. Screw configurations vary in the literature and options include the use of unicortical, bicortical, fixed-angle, and variable angle screws depending on the plating system. It is generally accepted that engaging eight cortices either side of the fracture site is adequate for stability with a near-far configuration to be used where possible. Numerous biomechanical studies have been performed on simulated B1 fractures in order to determine the best method of internal fixation. Plate and allograft strut constructs have been shown to be stiffer in bending and have a higher load to failure than plates used without a strut graft. Furthermore, a lateral locking cable-plate system used in combination with a second anterior locking plate is significantly stiffer than a single lateral locking plate with a strut allograft. However, the use of locking screws appears to have a limited biomechanical advantage. In a comparison of locking and nonlocking plates, the combination of a nonlocking plate with an allograft strut resulted in the highest stiffness. The use of cerclage fixation has also been subject to biomechanical testing and according to recent comparative studies, optimal fixation can be achieved through the use of double looped cables placed as far from each other as possible along the plating system. Furthermore, cerclage cables provide more fixation strength compared to stainless steel cerclage wires. Although there is concern that cerclage fixation may compromise periosteal vascularity, this has not been demonstrated in contact pressure studies of cadaveric human femora. Caution must be taken when applying these results to clinical practice as although stiff constructs can prevent failure of fixation, excessive stiffness is also associated with poor rates of fracture union.
Clinical results for internal fixation of type B1 fractures are extremely encouraging. Haddad and colleagues reported a 98% union rate in multicenter series of 40 patients treated with a cortical strut graft used either alone or in combination with a plating system. Ricci and coworkers reported on 41 consecutive patients treated with indirect reduction and a single lateral plate without the use of cortical strut or morselized allograft. All fractures united by 12 weeks without evidence of implant loosening or malalignment. Old and colleagues confirmed the successful treatment of B1 fractures through plate fixation without the additional use of cortical strut grafts in a series of 20 patients and reported a union rate of 95% with a mean time to weight-bearing of 10 weeks. They argued that the use of cortical strut grafts is unnecessary as they are expensive, carry the risk of disease transmission, and weaken as they integrate with host femur. Sandhu and colleagues reported the outcome of 20 patients treated with a modern cable-plate system (Dall-Miles plates; Stryker, UK) and achieved union in all subjects at a mean time of 3.9 months. More recently, minimally invasive fracture fixation techniques have been applied to the management of periprosthetic femoral fractures. Apivatthakakul and colleagues published their results of 10 patients with type B1 fractures using a locking compression plate and percutaneous cerclage wires. All fractures healed with a mean time to union of 18 weeks and there were no cases of implant failure, stem loosening, wound complications, or infections at a mean follow-up of 13.2 months.
Type B2 fractures involve a loose femoral stem that may be detected either on radiographs or intraoperatively. They are the most common type of periprosthetic femoral fracture and successful treatment requires fracture stabilization with revision to a long-stemmed implant that should bypass the most distal aspect of the fracture site by at least two cortical diameters. Soft tissue balancing is critical to avoid postoperative dislocation and can be achieved through preoperative digital templating. In the presence of loosening, the acetabular component must also be evaluated and revised if necessary. Loose femoral implants are usually relatively uncomplicated to remove but if further exposure is needed to remove cement or cement restrictors, an extended trochanteric osteotomy can be helpful. The revision stem can be implanted using either a cemented or a cementless technique and there is no firm consensus as to which method is superior. Most commonly, uncemented, extensively coated diaphyseal fitting stems ( Fig. 69-8 ) are used as they have an excellent track record and previous epidemiologic studies from the Swedish joint registry indicate that they have a lower reoperation rate. Following provisional reduction of the fracture, a line-to-line fit reaming technique should be used to avoid further displacement of the fracture. The revision stem provides intramedullary fixation but is then augmented with plates and/or cerclage cables prior to final reduction. Although this is suitable for femoral canals with parallel endosteal cortices, a fluted grit-blasted tapered stem may be more appropriate for narrow femoral canals or those with divergent cortices. Cemented revision implants are becoming more popular in the management of these fractures and advantages include a lower risk of iatrogenic fracture and immediate secure fixation that may be of particular importance in osteoporotic femora with a wide intramedullary canal. Cement-in-cement revision also allows for easier surgery as complete extraction of retained cement is unnecessary. However, some concerns exist regarding the extrusion of cement through the fracture site, which some believe may impair healing. This technique involves provisional fracture reduction while leaving the existing cement mantle in place followed by rigid fixation and cement-in-cement femoral revision. Bone grafting in the form of either impacted morselized allograft or cortical strut allograft can be used in order to promote healing and provide extramedullary stabilization.

MacDonald and colleagues reported a series of 14 proximal femoral fractures managed with extensively porous-coated long-stemmed femoral implants at a mean follow-up of 8.2 years. All fractures had united with a mean time to union of 4 months and no patients required revision surgery. Rayan and colleagues prospectively evaluated a series of 26 patients who were managed with uncemented femoral stems along with cortical struts, cable plating systems, bone allograft, and demineralized bone matrix as indicated. All fractures achieved both clinical and radiographic union with a satisfactory outcome reported by all patients. Neumann and colleagues reported their experience using a distally fixed uncemented modular stem in 53 patients at mean follow-up of 67 months. Although all fractures united, subsidence of the stem was seen in two patients (4%) within 6 months postoperatively and required further revision surgery. Ko and colleagues reviewed the results of 12 elderly patients with type B2 fractures with mean follow-up of 58.5 months. All patients were treated with long-stemmed uncemented Wagner implants and achieved fracture union with no implant failures. Most recently, Marx and colleagues reported on 15 type B2 fractures treated with the same implant and at mean follow-up of 74 months; all fractures had united but there was one case of aseptic loosening requiring revision.
Recently published studies provide clinical evidence regarding cement-in-cement revision that may be particularly useful in elderly patients and those unfit for lengthy surgical procedures. Richards and colleagues advised certain criteria to help select suitable patients for this type of surgery, that is, a good quality cement mantle that is well fixed to both the femur and fracture fragments in a low-demand patient. Briant-Evans and colleagues analyzed a series of 19 fractures treated with cemented stem revision and a lateral plate or cortical strut allograft and followed up for a mean of 3 years. Eighteen fractures achieved union by a mean of 4 months and there was no evidence of loosening on follow-up radiographs. One case of nonunion was reported, which was attributed to extruded cement from the fracture site at the time of surgery. In addition, the authors noted that the stem did not bypass the most distal fracture line leading to mechanical failure. Corten and colleagues reported on 28 elderly patients treated with cement-in-cement revision and additional allograft or plate fixation. No revisions were required by a mean follow-up of 46 months. However, seven major complications were seen within the first 3 months, including three deaths in patients with severe preexisting medical comorbidity, two deep infections, and one type C fracture following a fall. Although the mortality rate is high in this series, it cannot solely be attributed to a cemented technique as similar rates have been reported in a comparable group of patients undergoing uncemented revision.
Type B3 fractures involve varying degrees of bone loss caused by osteolysis and/or fracture comminution and are extremely challenging. For a successful outcome, both stem revision and restoration of bone stock are required and multiple options exist, for example, distally fixed uncemented femoral implants, cemented femoral implants, allograft prosthetic composites (APCs), proximal femoral arthroplasty (PFA), and most recently, modular fluted tapered femoral stems ( Fig. 69-9 ). Decision making is often difficult but can be guided by both the age and activity level of the patient. In the presence of severe bone loss, younger and more active patients are more likely to benefit from an APC as this enables reliable restoration of bone stock, which may be useful for any future arthroplasty procedure. Elderly patients may be best suited with a cemented PFA as this avoids the potential complications associated with more complex procedures. The use of additional bone grafting procedures to restore bone loss can help provide stability to the revision implant and aid fracture union. Richards and colleagues classified treatment options into (1) reconstruction of the deficient proximal femur using either impaction grafting or cortical strut allograft with a distally fixed uncemented femoral stem, (2) resection of the proximal femur with insertion of either an APC or PFA, and (3) a distally fixed, extensively coated femoral stem, which provides a scaffold for remaining proximal host bone to reconstitute.

Canbora and colleagues reported the results of nine type B3 fractures treated with extensively porous-coated femoral stems with a selective use of cortical strut allografts. At mean follow-up of 41.7 months, all fractures had united, and there were no reported cases of radiologic or clinical loosening. Garcia-Rey and coworkers reported a series involving 15 type B3 fractures treated with uncemented long revision stems without the additional use of cortical strut allografts. All fractures united and all stems were clinically and radiographically stable by mean follow-up of 3 years. Tsiridis and colleagues retrospectively reviewed a large series of 106 patients with both type B2 and type B3 fractures in which 89 patients were treated with cemented femoral stem revision and impaction allografting. According to an odds ratio analysis, patients treated with long stem revision and impaction grafting were significantly more likely to have united fractures than in those treated with a long stem implant without impaction grafting. Also, the use of long-stemmed implants was more than five times more likely to result in fracture union than short-stemmed implants. Maury and colleagues reviewed the results of 25 type B3 fractures in 24 patients treated with an APC over a mean follow-up of 5.1 years. Twenty-one patients reported mild or no pain and 23 patients were able to walk. Bony union of the allograft to the host femur occurred in 20 hips, but there was mild or moderate graft resorption in 6 hips. However, 4 of the 25 hips failed because of loosening of the APC, progressive varus angulation of the construct, or nonunion and required revision surgery.
Klein and colleagues reported the results of PFA in a retrospective series of 21 patients with type B3 fractures managed with a modular, proximally coated implant. At mean of 3.2 years’ follow-up, all but one were able to walk with minimal or no pain. The outcome was considered to be excellent or good in 11 hips (52.4%), fair for 9, and poor for 1. Local complications occurred in 28.6% of patients, including infection, dislocation, refracture, and acetabular failure following complex reconstruction. More recently, Zaki and colleagues reported the outcome of 37 type B3 fractures treated with cemented PFA and followed up for a mean of 14 years. The outcome was recorded as excellent or good in 24 patients (64.9%), fair for 10, and poor for 2. There were no reported cases of loosening or cement fracture. Local complications were seen in 19% of patients, including wound hematoma, superficial infection, and dislocation. The use of modular fluted tapered titanium stems has recently expanded in the management of femoral stem revision associated with diaphyseal bone loss. The tapered design of the stem includes fluted splines, which allow distal fixation where there is less than 2 cm of diaphyseal bone stock remaining. Mulay and colleagues reported their use in a series of 24 patients with both type B2 and B3 fractures and found that 91% of fractures united uneventfully. However, complications included five dislocations (20.8%) and one infection (4.2%). Abdel and colleagues retrospectively reviewed a series of 44 type B2 and B3 fractures treated with these implants for a mean of 2 years’ follow-up. They reported that 98% of patients had united fractures with stable implants on radiographic analysis. However, there were seven additional reoperations, including five for recurrent instability (11.4%) and two for deep infection (4.5%). Munro and colleagues have also recently presented their results of 47 patients with type B2 and B3 fractures with mean follow-up of 54 months. Radiographically, union was achieved in 98% with only one observed nonunion. Two femoral stems required revision (4.3%) due to loosening and deep infection. Although subsidence occurred in 24%, most migrated less than 3 mm and did not correlate to poor pain or functional scores.
Type C fractures are effectively fractures of the distal femur and do not usually result in implant instability. Therefore, they can be managed independently of the femoral stem. Although it is usual to manage these fractures with internal fixation, undisplaced or stable fractures can be managed nonoperatively with traction, braces, or a long leg cast in patients who are medically unfit for surgery. However, to prevent the problems associated with malunion or nonunion, operative intervention is recommended for displaced or unstable fracture patterns. Surgical options most commonly involve open reduction and internal fixation with plates but also include retrograde intramedullary nailing. Plating techniques have evolved with technological advances and there are now precontoured plates available with the options of both locking and nonlocking screws. Often, these are combined with cerclage fixation for further stabilization. Certain principles apply when planning plate fixation. First, the plate should be long enough to overlap the femoral prosthesis to avoid a potential stress riser, which may ultimately lead to an interprosthetic fracture. This can be achieved with the use of unicortical screws and cerclage fixation. Second, although locking plates have emerged as the primary fixation method for fractures affecting osteoporotic bone, there are recent concerns about the potential for failure in the treatment of type C fractures, particularly as they are more expensive than conventional plates. A parametric computer modelling study based on the management of a patient with a type C fracture compared rigid locking-plate fixation with a more flexible nonlocking plate. This revealed that rigid fixation with a locking plate and a short bridging length ultimately failed while a flexible nonlocking plate with a larger bridging length promoted healing. Furthermore, a comparative study of 40 patients with type B1 and type C fracture treated with either locking or nonlocking plates failed to find any evidence in favor of locking plates with regard to union rates, hip scores, walking ability, operation time, or blood loss. Finally, the use of supplementary cortical strut allografts in order to improve bone stock has also been studied. Font-Vizcarra and colleagues reported a series of 14 patients treated with plate fixation and a cortical strut graft, and by mean follow-up of 3.2 years, all but one had successfully united and there were no cases of fixation failure or plate breakage. Khashan and colleagues retrospectively compared the results of a series of 21 patients who were treated with plate fixation with or without an additional cortical strut graft. At mean follow-up of 23 months, there was no difference in time to union but there was a significantly higher rate of failure in the group treated by a plate alone. Therefore, in the presence of a type C fracture with compromised bone quality serious consideration should be given to the use of a cortical strut allograft.
The clinical outcomes of the treatment of type C fractures with plate fixation vary in the literature. Zuurmond and colleagues reported a 52% reoperation rate in a series of 21 patients with a type C fracture of whom 20 underwent open reduction and internal fixation with a dynamic compression plate, a standard nonlocking plate with cerclage wires, or a dynamic condylar screw plate. Chakravarthy and colleagues analyzed a series of 12 patients involving six with a type C fracture treated with a distal femur Less Invasive Stabilization System (LISS) or a locking compression plate. There were two implant failures that were attributed to technical errors, including inadequate plate length leading to an interprosthetic fracture above a TKA and improper fracture reduction. Froberg and colleagues reported their series of 60 consecutive patients with both type B1 and type C fractures treated with locking plates. At a median follow-up time of 23 months, eight patients (13%, four type B1, four type C) had required reoperation. Of the type C fractures in the reoperation group, all had initially been treated with a LISS plate with failure of fixation occurring in three patients and stem loosening occurring in one patient due to misinterpretation of the initial radiograph.
The treatment of type C fractures with retrograde intramedullary nailing is an attractive option as it is less invasive than open reduction techniques although it is limited by both the presence of the femoral stem and the potential for leaving a stress riser. Wroblewski and colleagues reported an early series of eight cases of femoral shaft periprosthetic fracture that were treated with both a Küntscher nail and a modified Charnley cemented stem. In this small series, all fractures united at a mean time of eight months. Ponzer and coworkers also published good results in another small series of seven patients with fractures distal to an uncemented hemiarthroplasty treated with retrograde nailing. At follow-up of 7 to 15 months, all fractures united and there were no major complications. In order to provide a solution to the potential problem of leaving a stress riser, Zuurmond and colleagues innovatively designed a bridging nail made to slide over the tip of the in situ femoral stem. In a series of 18 patients treated with this retrograde nail, all fractures had evidence of radiographic union by 1 year but revisions were required in two patients because of implant failure and protrusion of the nail into the knee joint.
Bone Grafting
Bone graft is widely used in the treatment of periprosthetic fractures and can take a variety of forms. It is a useful adjunct in reconstituting bone loss in the presence of severe osteolysis or fracture comminution, although it can be used in nearly all fracture patterns. In addition to improving bone stock, it can also enhance stability and fracture healing. Autogenous bone graft is an excellent method of providing osteoconductive, osteoinductive, and osteogenic bone cells to the fixation construct. Cancellous bone graft is highly osteogenic but provides limited structural support. In contrast, cortical bone graft is initially mechanically superior but is at risk of osteoporosis and subsequent resorption by osteoclasts. Furthermore, autogenous bone graft is limited by supply and donor site morbidity. Therefore, the gold standard in the management of bone loss in revision hip arthroplasty is allograft, which is more readily available. However, it lacks the osteogenic capabilities of autograft and carries a small risk of infection and disease transmission.
Usually allograft takes the form of either morselized bone graft, which can be impacted into the bony defect or a strut graft, which is used in cortical onlay fixation. Impaction grafting is an intramedullary grafting technique in which morselized cancellous or corticocancellous allograft bone chips are impacted into the femoral canal to provide a “neo-endosteum” for a prosthesis to be inserted. Cortical strut grafts function as biologically active extramedullary devices, which enhance bone stock and are associated with less stress shielding than metal plates. In cases of massive structural bone loss, proximal femoral allograft can be used in conjunction with a prosthetic implant such as an APC.
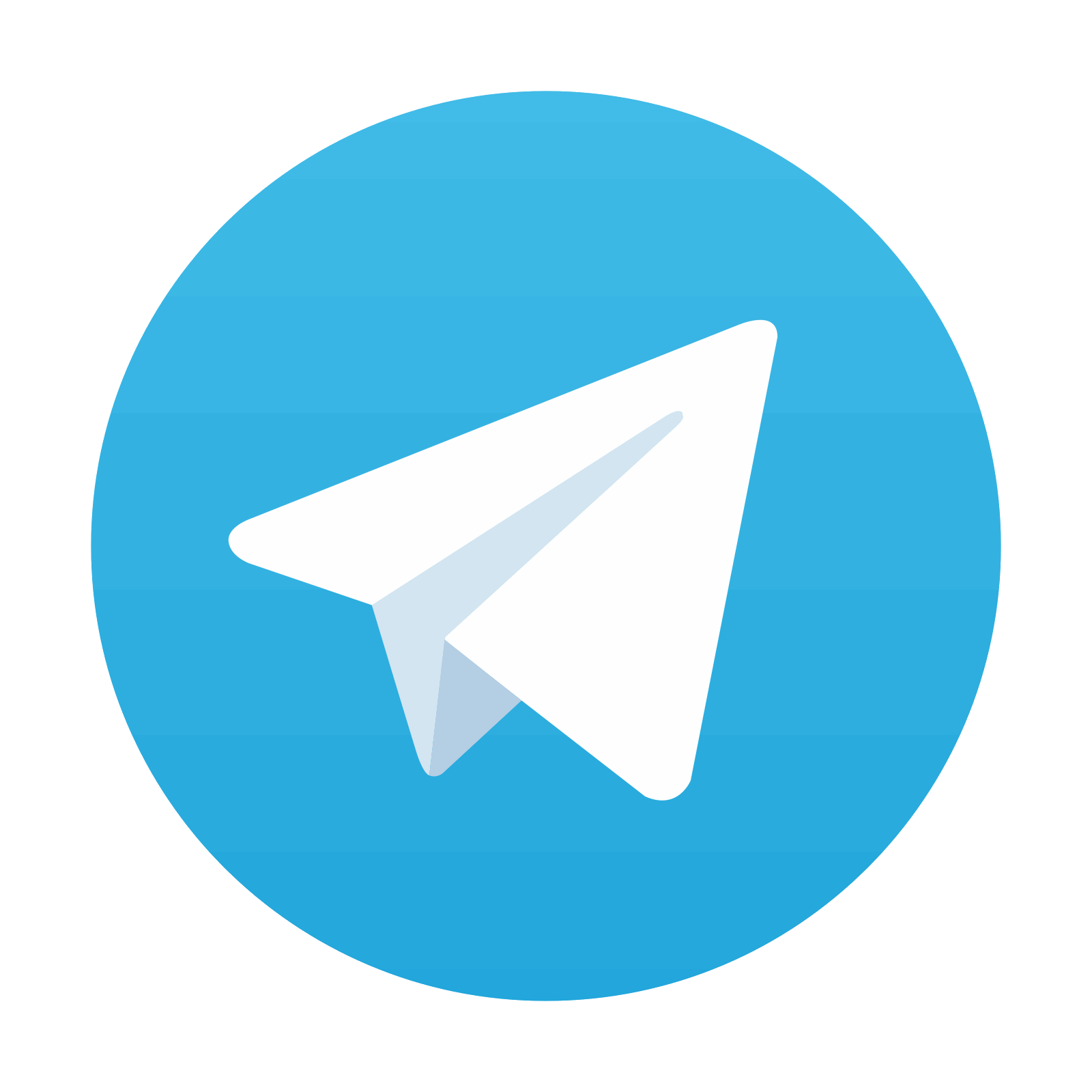
Stay updated, free articles. Join our Telegram channel

Full access? Get Clinical Tree
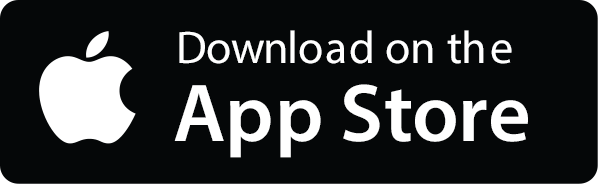
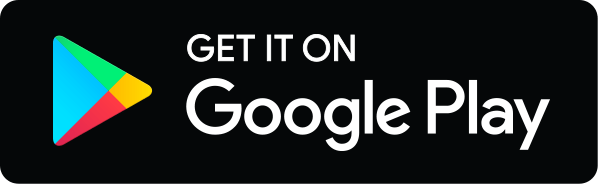