Abstract
Objectives
Activities that provoke stress urinary incontinence (SUI) rapidly increase the intra-abdominal pressure and the impact loading on the pelvic floor muscles (PFMs). Coughing can cause urinary leakage and is often used to test SUI. However, PFM characteristics during coughing, including their reliability, have not been investigated. Here, we used electromyography (EMG) to describe PFM pre-activity and reflexivity during coughing and examined the reliability of the measurements.
Methods
This was an exploratory and reliability study including 11 young healthy women to characterize EMG reflex activity in PFMs during coughing. We describe 6 variables, averaged over 3 coughs per subject, and tested their reliability (intraclass correlation coefficient 3,1 [ICC(3,1)] and ICC(3, k ), related standard error of measurement (SEM) and minimal difference [MD]). The variables represented the mean EMG activity for PFMs during 30-ms time intervals of pre-activity (initial time point of coughing [ T 0] and minus 30 ms) and reflex activity ( T 0–30, 30–60, 60–90, 90–120 and 120–150 ms after T 0) of stretch-reflex latency responses.
Results
The mean %EMG (normalized to maximal voluntary PFM contraction) for EMG variables was 35.1 to 52.2 and was significantly higher during coughing than for PFM activity at rest (mean 24.9 ± 3.7%EMG; P < 0.05). ICC(3, k ) ranged from 0.67 to 0.91 (SEM 6.1–13.3%EMG and MD 16.7–36.8%EMG) and was higher than ICC(3,1) (range 0.40–0.77; SEM 9.0–18.0%EMG, MD 24.9–50.0%EMG).
Conclusions
PFM activity during reflex latency response time intervals during coughing was significantly higher than at rest, which suggests PFM pre-activity and reflex activity during coughing. Although we standardized coughing, EMG variables for PFM activity showed poor reliability [good to excellent ICC(3, k ) and fair to excellent ICC(3,1) but high SEM and MD]. Therefore, coughing is expected to be heterogeneous, with low reliability, in clinical test situations. Potential crosstalk from other muscles involved in coughing could limit the interpretation of our results.
1
Introduction
The signs of stress urinary incontinence (SUI) are involuntary leakage from the urethra with effort or physical exertion or on sneezing or coughing . Stress leakage is presumed to be due to increased abdominal pressure . Activities or efforts that typically provoke SUI rapidly increase the intra-abdominal pressure and the impact loading on the pelvic floor muscles (PFMs) . Such impact loads occur within milliseconds (ms); for example, peak-flow happens within 57 to 110 ms during coughing in women .
Fast and high impact loads on the PFMs require reflexive muscle contractions to guarantee continence . Amarenco et al. investigated external anal sphincter electromyography (EMG) during coughing and found that cough intensity was significantly related to EMG activity for the anal sphincter. The authors concluded that the cough anal reflex is not an unambiguous response (response or non-response) but rather a modulated reflex. Deffieux et al. also compared SUI affected with continent women and found a lack of modulated PFM response during successive coughing efforts. Both groups concluded that this gradual PFM adaptation might be one of the main factors contributing to urinary and fecal continence in women . Howard et al. investigated vesical neck descent by using ultrasonography during coughing in standing continent nulliparous women and found a mean downward movement of 8.2 ± 4.1 mm. Talasz et al. used real-time dynamic MRI and found a mean PFM downward movement of 7.4 ± 5.8 mm during coughing in healthy nulliparous women in the supine position.
The PFM downward displacement during coughing , which could mean an eccentric movement, together with the fast impact load on the PFMs during coughing suggested that PFM reflex activity during coughing might concern stretch reflexes. Stretch reflexes can be classified according to their latencies (i.e., reflex peaks), which occur within the first 150 ms after an impact and are characterized by slow, medium and long-latency responses and long-latency succeeding responses in relation to an impact (e.g., the impact and beginning loading phase and strain on the PFMs during coughing).
Because coughing rapidly increases the intra-abdominal pressure and typically provokes SUI, it is often used to provoke urine leakage and to test for SUI. Therefore, coughing is used in different pad tests recommended by the International Continence Society ; during the cough stress test, which is easy to conduct and thus particularly convenient in the clinical setting ; and for assessment of urethral sphincters and bladder neck mobility during the Q-tip test . Furthermore, coughing is used to test for the effects of PFM training on SUI symptoms .
However, little is known about the PFM behavior or contraction characteristics, muscle action or muscle activity during coughing. Furthermore, few studies have investigated the reliability of such PFM activity characteristics during coughing. Here, we aimed to characterize PFM pre-activity and reflexivity during coughing measured by EMG and to assess the reliability of the measurements.
2
Materials and methods
2.1
Study design
This trial was designed as an exploratory and intra-session retest reliability study to characterize PFM activity during coughing. It focused on the reliability of 6 previously defined EMG variables of pre-activity and reflexivity . The study was conducted in accordance with the Declaration of Helsinki, and all participants gave written informed consent. According to the Swiss Human Research Act, approval from the Ethics Committee of the Canton of Bern was not required because the investigation concerned a physiotherapy-relevant reliability low-risk study.
2.2
Participants
We included 11 female participants from the Bern University of Applied Sciences who were 20 to 35 years old, nulliparous and healthy on the basis of the anamnesis and had a BMI between 18 and 25 kg/m 2 . Exclusion criteria were menstruation on the measurement day, surgery in the urogenital region, acute vaginal infection, incontinence, pelvic floor complaints, acute back or joint pain, nickel or latex allergy, or chronic cough or acute respiratory disorder. All participants were familiar with maximal voluntary contraction (MVC) of their PFMs because they had learned about isolated PFM (maximal) contraction as part of the practical PFM rehabilitation program in their professional physiotherapy or midwifery education.
2.3
Instrumentation
PFM activity was measured with a vaginal surface EMG probe (Periform ® , Neen, UK-Oldham Lancashire: Bipolar stainless steel electrode, rectangular size of 4.9 cm 2 per electrode, mediolateral electrode location with a good vaginal fixation because of the cavity of the electrode, depth of electrode insertion until the retaining ring contacts the internal labia). A single reference adhesive surface electrode (Nicolet; Viasys Healthcare, Warwick, UK) was fixed on the right iliac crest according to the SENIAM recommendations . EMG involved a 16-channel telemetry system (TeleMyo 2400 G2, Noraxon USA Inc., Scottsdale, AZ; digital high pass filter: 10 Hz, digital low-pass filter: 500 Hz; gain: 500). A 3-D accelerometer (22 × 16 mm; Noraxon European Service Center, Cologne, Germany) was fixed on the processus xiphoideus sterni to identify the onset of acceleration ( T 0) (i.e., the very beginning of a cough and therefore the impact and beginning loading phase and strain on the PFMs). A Voldyne ® (HUDSON RCI ® , Voldyne ® 5000 mL) was used to determine the maximal inspiratory volume in milliliters and a peak-flow-meter (AsmaPLAN, Vitalograph GmbH, Hamburg, Germany) to measure the cough following maximal inspiration (peak-flow value of at least 250 L/min ).
2.4
Procedures
To familiarize participants with the testing procedure, they were asked to perform 3 test coughs through the peak-flow meter, followed by maximal inspiration by using the Voldyne ® . Thereafter the participants were equipped with the accelerometer and the EMG reference electrode and were instructed in how to vaginally insert the EMG probe by using ultrasonography lubrication and then performed the insertion themselves after emptying the bladder. Maximal inspiratory volume measured with the Voldyne ® involved the subject standing (instructions: stand on a marked spot on the floor with feet hip-width apart, hips and knees minimally bent; one hand holding the Voldyne ® tube close to the mouth and the other holding the handle vertically in front of the body and keep the mouth tightly sealed around the mouthpiece of the tube). Two retests were taken, each following a 15-s break.
EMG of the PFMs was performed twice for 30 s at rest (i.e., without any voluntary contraction) and twice for 5 s during maximum voluntary contraction (contraction as fast and maximal as possible) with the participant standing, always taking a 30-s break between measurements. We chose to perform the MVC test with the participant standing instead of the usual supine position because it seemed more functional for coughing in that the PFMs has to contract against gravity .
EMG and accelerometer signals were measured continuously during 3 coughs. For coughing, the participants were instructed to stand as relaxed as possible (feet hip-width apart, hips and knees minimally bent) and not perform any voluntary contractions of the PFM, hold the peak-flow-meter with both hands, keep their mouth tightly sealed around the mouthpiece and then perform 3 coughs of maximal expulsion effort through the peak-flow-meter after maximal inspiration without using the Voldyne ® but in the same manner (i.e., with an inspiratory volume as equal as possible) as during Voldyne ® measurements. A 15-s break was taken between coughs.
2.5
Data reduction
EMG and accelerometry signals were sampled at a rate of 2 kHz (sampling interval [dt] = 0.5 ms) by using a 12-bit analog-to-digital converter (ME-2600i, SisNova Engineering, Zug, Switzerland) and the software Analoge und digitale Signalverarbeitung (ADS) 1.12 (uk-labs, Kempen, Germany).
The EMG signals were 1st-order high-pass – filtered with a cut-off frequency of 10 Hz (± 10%) and low-pass – filtered with a cut-off frequency of 1.5 kHz by EMG preamplifier leads. EMG variables were calculated according to existing study protocols as RMS values within 30-ms intervals, representing the reflex latency responses (See Table 1 ) with use of Matlab R2013a (The Mathworks, Inc.). To identify amplitude peaks during MVC, EMG was calculated as RMS (200-ms moving window) by using the ADS software. EMG 100% equals the average of the 2 peak amplitude values during the two 5-s sessions of MVC. The EMG variables were averaged over 3 coughs and normalized to peak MVC (%EMG). The EMG activity variables are described in Table 1 . EMG data during standing at rest and MVC were averaged over the test and retest, and PFM EMG data for coughing were averaged over 3 coughs per participant.
Variable | Unit | Description | To identify |
---|---|---|---|
T –30–0 | %EMG | Mean EMG activity between –30 ms and T 0 | Representing the pre-activity as a regulatory component of anticipation |
T 0–30 | %EMG | Mean EMG activity from T 0 to 30 ms | Representing the interval preceding latency responses |
T 30–60 | %EMG | Mean EMG activity from 30 to 60 ms after T 0 | Detecting short latency response characterizing reflex activity during a stretch-shortening cycle |
T 60–90 | %EMG | Mean EMG activity from 60 to 90 ms after T 0 | Detecting medium latency response characterizing reflex activity during a stretch-shortening cycle |
T 90–120 | %EMG | Mean EMG activity from 90 to 120 ms after T 0 | Detecting long-latency response characterizing reflex activity during a stretch-shortening cycle |
T 120–150 | %EMG | Mean EMG activity from 120 to 150 ms after T 0 | Detecting long-latency succeeding response characterizing reflex activity during a stretch-shortening cycle |
The variables of pre-activity and variables of slow, medium and long latency and long-latency succeeding responses to characterize reflex activity were chosen from protocols of former studies . Because basically no clear and reproducible reflex peaks after the initial time point of coughing (i.e., the impact and beginning loading phase and strain of the PFMs) could be determined visually on the rectified EMG for consecutive coughs, we calculated the mean amplitudes for fixed 30-ms intervals covering the phase in which reflex activity is expected to occur. Therefore, reflex phase amplitudes were calculated from 30 to 60 ms (short latency response), 60 to 90 ms (medium latency response), 90 to 120 ms (long-latency response) and 120 to 150 ms (long-latency succeeding response). Additionally, pre-activity was computed for the interval between –30 ms and T 0 .
2.6
Statistical analysis
We used G*Power to estimate a sample size of ≥ 9 and associated power of 0.83 with a bivariate normal model based on the following assumptions: one-tailed test, correlation coefficient of alternative hypothesis: 0.75; alpha error probability: 0.05; power (1 – beta error probability): 0.80; correlation coefficient of null hypothesis: 0.00. Data are described with mean ± SD. The reliability test followed the 3-step suggestions of Weir : to identify possible systematic errors between the repeated measures, the Friedman test and Wilcoxon rank order test were applied for participants at rest and the MVC tests, and the Friedman test for n -dependent samples was used to compare EMG over the 3 coughs as well as peak-flow and inspiratory volume. For the PFM EMG variables, reliability was calculated for single and average measures (consistency) with the two-way fixed intraclass correlation coefficients [ICC(3,1) and ICC(3, k )] (i.e., relative reliability), which do not consider systematic error. We computed the absolute standard error of measurement ( <SPAN role=presentation tabIndex=0 id=MathJax-Element-1-Frame class=MathJax style="POSITION: relative" data-mathml='SEM=SD×1−ICC2′>SEM=SD×1−ICC−−−−−−−√2SEM=SD×1−ICC2
S E M = S D × 1 − I C C 2
; i.e., absolute reliability), relative SEM related to the mean (SEM%), and the absolute minimal difference ( <SPAN role=presentation tabIndex=0 id=MathJax-Element-2-Frame class=MathJax style="POSITION: relative" data-mathml='MD=SEM×1.96×22′>MD=SEM×1.96×2–√2MD=SEM×1.96×22
M D = S E M × 1.96 × 2 2
) needed to be considered real, and relative MD related to the mean (MD%). SEM and MD were calculated twice, once for the ICC(3,1) and once for the ICC(3, k ). Regarding participants at rest and MVC as well as peak-flow and maximal inspiratory volume, only ICC(3, k ) and related SEM and MD values were calculated. To evaluate relative reliability, ICC benchmarks from Shrout and Fleiss were used: > 0.75 excellent, 0.40–0.75 fair to good and < 0.40 poor. P < 0.05 was considered statistically significant. Statistical analysis involved use of IBM SPSS 22 for Windows (SPSS, Inc., Chicago, IL).
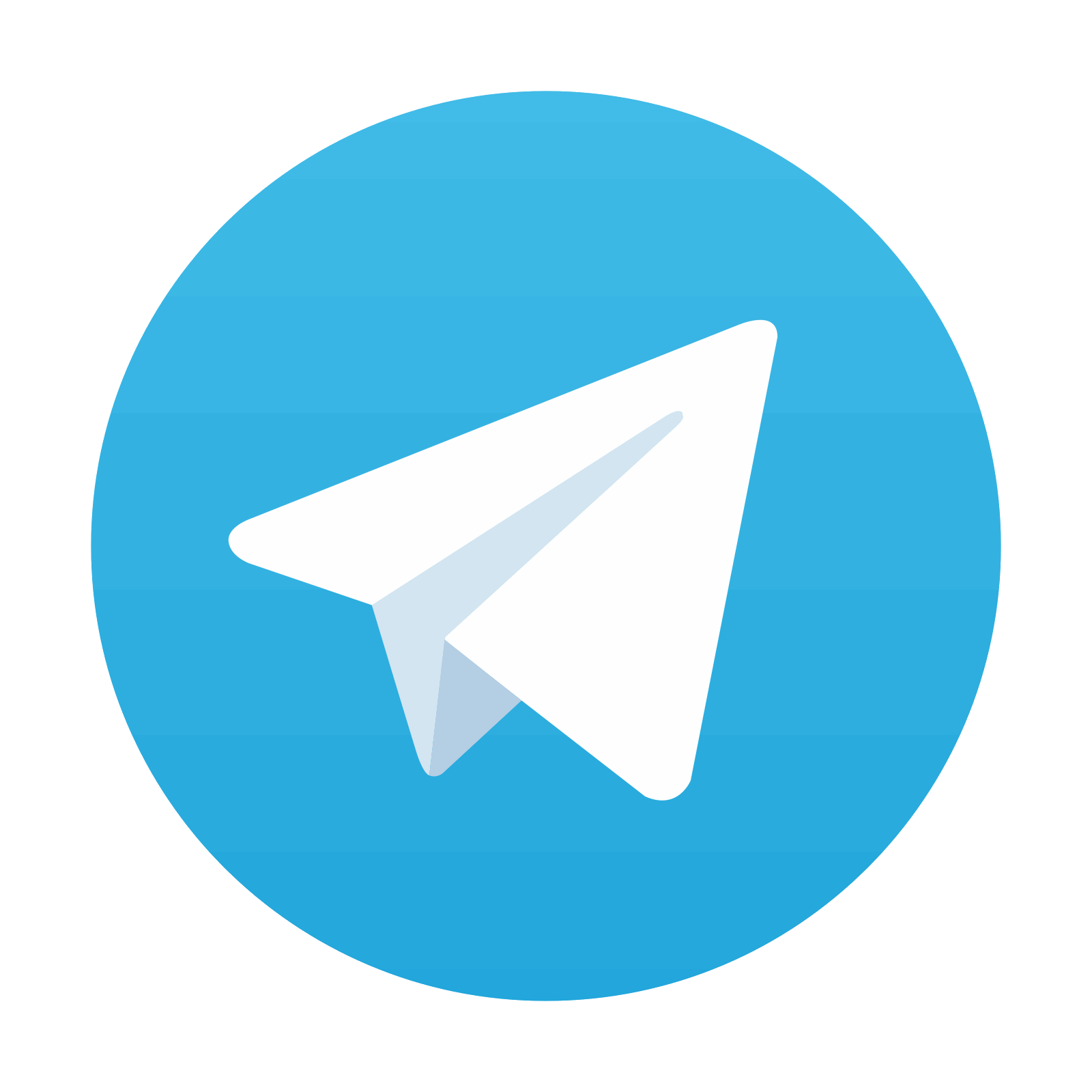
Stay updated, free articles. Join our Telegram channel

Full access? Get Clinical Tree
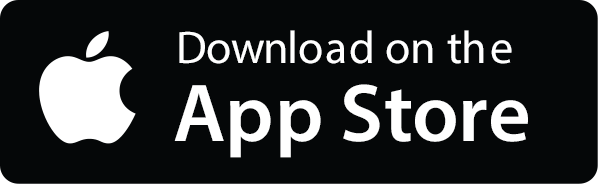
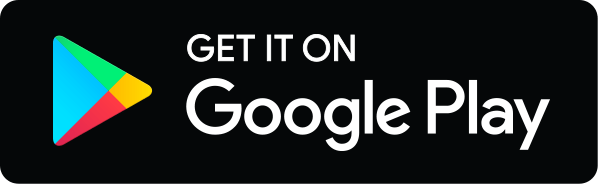