Risk Factors for Development of Musculoskeletal Disorders
- ▪
Workplace Factors
- ▪
Repetition
- ▪
Force
- ▪
Awkward, extreme, or fixed postures
- ▪
Cold temperatures
- ▪
Vibration
- ▪
Job-related psychosocial factors
- ▪
- ▪
Nonworkplace Factors
- ▪
History of injury or disease affecting musculoskeletal tissue(s) (comorbidities)
- ▪
Nonwork activities and lifestyle factors
- ▪
Non-job-related psychosocial factors
- ▪
Advanced age
- ▪
Female gender (industry-specific)
- ▪
Obesity
- ▪
Pathophysiology of Musculoskeletal Disorders
- ▪
Forceful or repetitive tasks can lead to tissue injury, tissue reorganization, or central nervous system (CNS) reorganization. Any of these pathways can lead to chronic disability if causal factors are perpetuated or if appropriate interventions are not implemented.
- ▪
Repeated microtrauma to previously injured tissues prevents normal healing and reconstitution of structure and physiology.
- ▪
There is an exposure-response relationship between ergonomic factors (e.g., force and repetition) and the severity of musculoskeletal disorders (MSDs), with higher levels of exposure leading to greater pathology.
- ▪
Tissue injury or reorganization can occur in multiple tissues, including muscle, tendon, loose connective tissue, peripheral nerve, and bone.
- ▪
Tissue injury elicits an inflammatory response, which may be acute or chronic (or both) or systemic. Systemic inflammation can cause widespread symptoms.
- ▪
Tissue reorganization is typically pathophysiologic (e.g., degenerative changes, fibrosis) in animal models and human studies of MSD. Compromised tissues have reduced tolerance for exposure to repetitive or forceful tasks and are more vulnerable to reinjury, thus perpetuating a cycle of injury, pain, and disability.
- ▪
Awareness of the pathophysiologic processes involved with MSDs can facilitate development of effective intervention and prevention strategies.
Musculoskeletal Disorders
This chapter reviews current knowledge about the pathophysiologic mechanisms of work-related MSDs. It is important for the clinician to appreciate the pathophysiology of MSDs in order to develop effective interventions and prevention tactics. The U.S. Department of Labor defines MSDs as injuries or disorders of muscles, nerves, tendons, joints, cartilage, or spinal disks associated with exposure to risk factors in the workplace. Examples of MSDs include sprains, strains, tears, back pain, soreness, carpal tunnel syndrome, and musculoskeletal or connective tissue diseases. Upper extremity diagnoses commonly associated with MSDs include tendinopathies, nerve compression or entrapment syndromes, vascular disorders, muscular disorders, joint disorders, and regional complaints of pain that cannot be attributed to a specific clinical diagnosis. MSDs account for a significant proportion of work injuries and workers’ compensation claims in Western industrialized nations (see Chapter 140 ). The economic toll of MSDs is staggering. Direct and indirect costs for work-related injuries were estimated to be $54 billion annually for reported cases.
Documentation of the phenomenon of repetitive motion injury dates back to 1713, when “writer’s cramp” symptoms among scribes and notaries were reported by Ramazzini in his book titled De Morbis Artificum . Ramazzini wrote, “The maladies that affect the clerks arise from three causes: first, constant sitting; secondly, incessant movement of the hand and always in the same direction; and thirdly, the strain on the mind … the incessant driving of the pen over paper causes intense fatigue of the hand and the whole arm because of the continuous … strain on the muscles and tendons.”
Contemporary reviews of epidemiologic research found evidence that MSDs of the hand and wrist have been associated with performance of repetitive and forceful tasks; performance of tasks in awkward, extreme, or fixed postures; in cold temperatures; and with vibration. The National Research Council and Institute of Medicine review of studies related to MSDs supported the association between both physical and psychosocial workplace exposures and upper extremity MSDs.
Work in certain industries increases the risk of developing a MSD. In 2006, occupations with the highest number of MSDs were nursing aides, orderlies, attendants; laborers; freight, stock, material movers; and tractor-trailer drivers. A sizable percentage (18%) of MSDs occur in the manufacturing sector. These epidemiologic trends clearly suggest that the task demands associated with certain jobs are related to the incidence of MSDs. In addition, specific ergonomic and job sector risk factors and nonworkplace factors such as individual predisposition and comorbidities (see Critical Points for a list all risk factors) can play a role in the development of MSDs. For example, a sewing machine operator with a history of fibromyalgia, degenerative joint disease, and heavy cigarette smoking is at greater risk for developing an MSD than is an otherwise healthy nonsmoker who performs that same job. The relative contributions of these nonworkplace risk factors are elusive and may vary greatly among individuals. Further study of the role of nonworkplace factors is needed.
The exposure-response relationships between repetitive tasks and MSDs in the upper extremities have not been well defined. Scientific investigation in this area is ongoing. Multiple studies suggest a strong relationship between the development of MSDs and the interactive effects of repetition and force. Silverstein and colleagues reported that prevalence ratios for MSDs were 3.6 for high-repetition/low-force tasks, 4.9 for low-repetition/high-force tasks, and 30.3 for high-repetition/high-force tasks. This suggests that force and repetition have a more than additive effect in increasing the risk for development of MSDs.
A large number of studies using animal models (summarized in Tables 137-1 to 137-4 ) have illustrated the exposure-response relationship among repetitive tasks, tissue injury, and behavioral changes. For example, Barbe and coworkers reported exposure-dependent differences in proinflammatory cytokines/chemokines, macrophages, and grip strength based on exposure to low- or high-repetition reaching in rats. Using the same rat model, other studies from the same lab found declines in motor performance were greater with high-repetition/negligible-force reaching than with low-repetition/negligible-force reaching. Clark and associates found that declines in median nerve conduction velocity and motor performance were greater after exposure to high-force repetitive reaching versus negligible-force repetitive reaching. Compared with Clark and associates’ findings in rats that performed high-repetition/negligible-force reaching, Elliott and colleagues found 17% fewer inflammatory cells in the median nerve and delayed macrophage infiltration into the nerve in rats that performed a low-repetition/negligible-force task. Elliott and colleagues also found fewer TNF-α-positive cells than did Al-Shatti and coworkers, who exposed rats to high-repetition/negligible-force reaching. Nakama and associates found that adverse microstructural changes were greater with a higher rate of repetition in rabbit flexor digitorum profundus tendon. Findings such as these illustrate that the magnitude of exposure is related to the severity of pathophysiologic change.
Reference | Model/Tissue | Exposure | Key Findings |
---|---|---|---|
|
| 11 weeks: Increased mRNA expression of type III collagen and matrix metalloproteinase | |
Rat supraspinatus tendon |
| 2 and 4 weeks: Up-regulation of multiple cartilage-specific genes; down-regulation of genes normally associated with tendon phenotype | |
|
|
| |
|
|
| |
|
|
| |
|
|
| |
|
|
| |
| Forced lengthening 5 × 10 repetitions |
| |
|
|
| |
| Cast immobilization ×3 weeks followed by treadmill running 3–11 weeks | Recovery of tenascin-C after 8 weeks of running; expression of tenascin-C was exposure-dependent | |
|
|
| |
|
| 14 weeks: Increased microstructural changes (tear area, tear density, and mean tear size); increased VEGF, VEGFR-1, and CTGF cell densities | |
|
| 14 weeks: Increased microstructural changes (tear area, tear density, and mean tear size) in outer regions of tendon; less than with higher repetition rate in earlier studies | |
|
|
| |
|
| 4, 8, and 16 weeks: Increased cellularity, collagen disorganizaton, increased cross-sectional area, degradation of material properties | |
|
| 4, 8, and 16 weeks: Increased cellularity, collagen disorganization, increased cross-sectional area, degradation of material properties | |
|
|
| |
|
| Hypervascularity: Decreased muscle mass and myofiber area; increased noncontractile tissue and collagen content | |
|
| 4 weeks: Increased mRNA expression of inducible and endothelial nitric oxide synthase | |
|
| One of three animals with increased tenocytes density, disorganized collagen, and large areas of pink-stained glycosaminoglycans |
Reference | Model/Tissue | Exposure | Key Findings |
---|---|---|---|
|
|
| |
|
|
| |
|
|
| |
|
|
| |
|
| Demyelination and degeneration/regeneration; hypervascularity; intraneural fibrosis, decreased nerve conduction velocity | |
| 4–12 months | Demyelination; decreased neural tissue in fascicles; intraneural fibrosis | |
|
| Declines in nerve conduction velocity in three of four working hands; enlargement of nerves; recovery of NCV after termination of task exposure |
Reference(s) | Model | Exposure | Key Findings |
---|---|---|---|
Rat |
|
| |
|
| Decreased grip strength at weeks 6 and 8 in the high-repetition group, and at week 6 in the low-repetition group | |
Monkey |
|
| |
Monkey |
|
| |
Rat |
| Increased movement reversals at week 8; trend for reduced task duration at week 12 | |
Rat |
| Decline in reach rate at weeks 6 and 9; increased number of reach reversals and total reach time at week 9 in both high- and low-repetition groups | |
Rat |
|
| |
Rat |
| Adverse gait changes in 2 rats at 3 and 4 weeks; limping in 1 rat at 3 weeks | |
Primate |
| Declines in attempts and successes in 2 of 4 animals; decline in successful trials per day in 2 of 4 animals around the time of diagnosis of carpal tunnel syndrome | |
Monkey |
| Difficulty gripping and reduced ability to flex digits in 1 animal at 5 weeks and the other animal at 24 weeks |
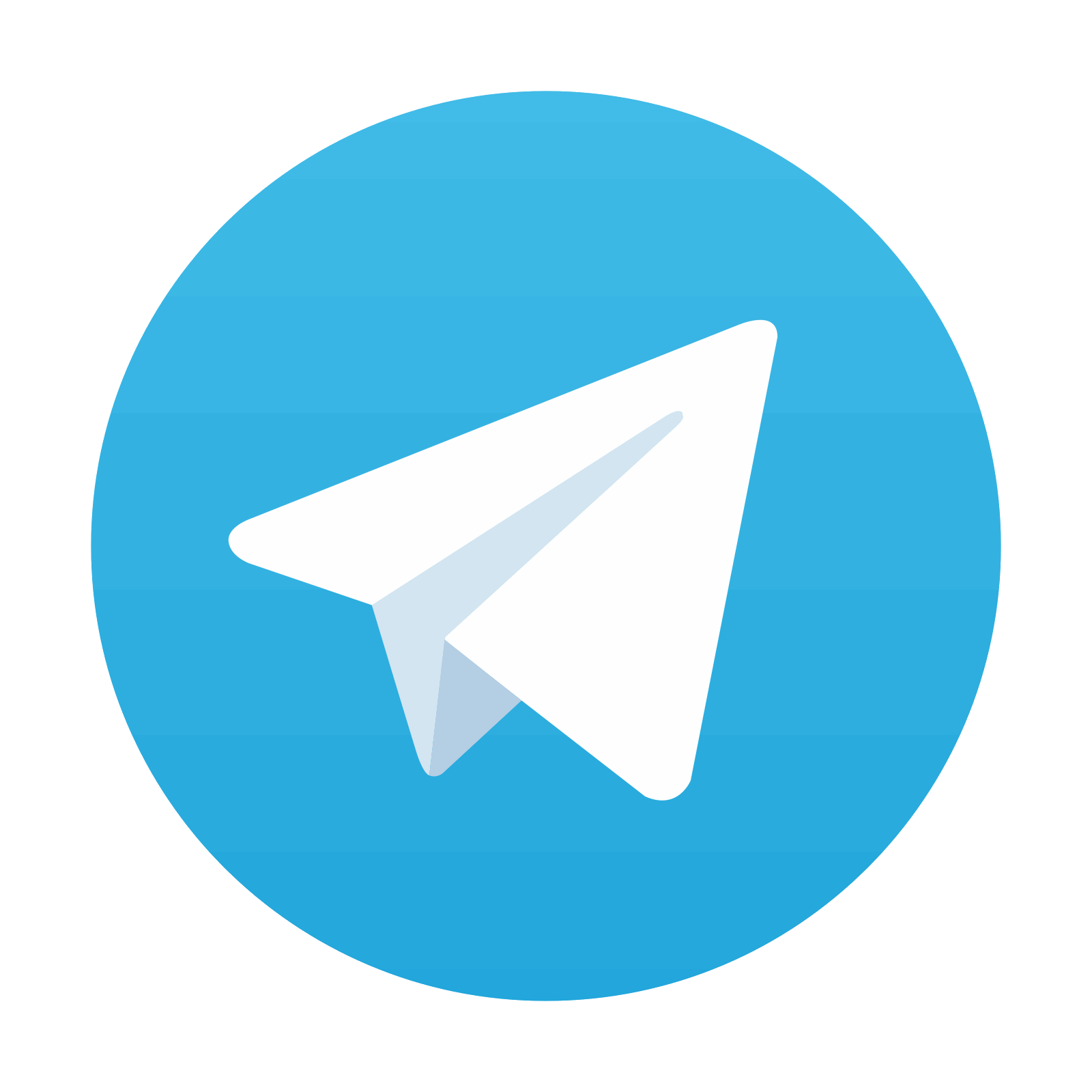
Stay updated, free articles. Join our Telegram channel

Full access? Get Clinical Tree
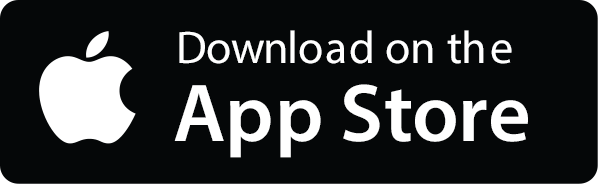
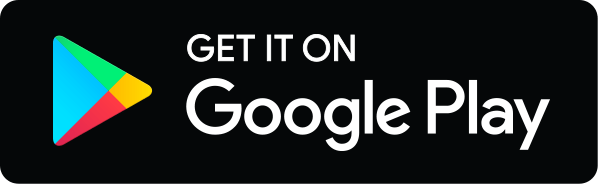
