Traumatic brain injury (TBI) has become the signature injury of the military conflict in Iraq and Afghanistan and also has a high rate of occurrence in civilian populations in the United States. Although the effects of a moderate to severe brain injury have been investigated for decades, the chronic effects of single and repetitive mild TBI are just beginning to be investigated. Data suggest that the different types and severities of TBI have unique long-term outcomes and thus may represent different types of diseases. Therefore, this review outlines the causes, incidence, symptoms, and pathophysiology of mild, moderate, and severe TBI.
Key points
- •
Traumatic brain injury (TBI) has become the signature injury of the military conflict in Iraq and Afghanistan and also has a high rate of occurrence in civilian populations in the United States.
- •
Although the effects of a moderate to severe brain injury have been investigated for decades, the chronic effects of single and repetitive mild TBI are just becoming investigated.
- •
Data suggest that the different types and severities of TBI have unique long-term outcomes and thus may represent different types of diseases.
- •
Therefore, this review outlines the causes, incidence, symptoms, and pathophysiology of mild, moderate, and severe TBI.
Traumatic brain injury: causes, prevalence, and development of the national research action plan
Traumatic brain injury (TBI) may have many different causes, including a blow to the head, penetration of the skull, fast acceleration or deceleration of the head, or exposure to a blast. In the United States, there are more than 5.3 million people living with a disability as a result of a TBI, and each year an additional 1.7 million Americans sustain a TBI. These injuries have both short- and long-term effects on health, ranging from symptoms that have a minimal interference on lifestyle, through to physical, emotional, and psychosocial changes that may interfere with daily activities. As well as the burden to the individual, brain injuries also have an annual economic burden of more than US$60 billion, due to both direct and indirect costs, such as loss of productivity. The reasons for the injuries depend on the age of the individual; for example, more than one-third of brain injuries in the United States are due to people falling, which is the leading cause of TBI among the elderly, whereas transportation-related brain injuries are the leading cause for individuals aged 15 to 24 years.
In recent years, the media have had a strong focus on the long-term outcomes of mild TBI (mTBI) or concussions, such as that which may occur to American football players and military personnel. Pathophysiologic investigations on the brains of these individuals have revealed Alzheimer-like symptoms, termed chronic traumatic encephalopathy (CTE). Although this disease has been known to occur in boxers since the 1960s (with numerous names, including dementia pugilistica and punch-drunk syndrome), the recent media attention has highlighted the high prevalence of this disease in other athletic arenas. This attention was followed by the Obama Administration releasing an Executive Order in 2012 to direct the Departments of Defense, Veterans Affairs, Health and Human Services, and Education to develop a National Research Action Plan (NRAP) on TBI, posttraumatic stress disorder (PTSD), and other common comorbidities. The NRAP was released in August 2013 and aims to (1) accelerate the understanding of the causes and mechanisms of TBI, PTSD, and other common comorbidities; (2) develop clinical innovations to detect disorders early and accurately; and (3) develop proven means to prevent and treat the devastating conditions caused by the injuries. To this end, understanding the acute and chronic pathophysiology of TBI is critical to determining the risk factors for individuals to develop symptoms as well as develop therapeutic innovations and interventions.
Given that current clinical imaging techniques do not detect minor changes in the human brain following injury, much of the knowledge on the cellular sequelae in the injured brain is based on rodent studies. To confirm these findings in the injured human brain, on arrival at the emergency department, an individual can be assessed for physical and cognitive functions, have a computed tomographic (CT)/MRI scan performed, have their intracranial pressure and cerebral blood flow measured, and possibly have bio-specimens collected for analysis of protein and messenger RNA expression. Subsequently, on autopsy, brain samples can be collected and analyzed.
Traumatic brain injury: causes, prevalence, and development of the national research action plan
Traumatic brain injury (TBI) may have many different causes, including a blow to the head, penetration of the skull, fast acceleration or deceleration of the head, or exposure to a blast. In the United States, there are more than 5.3 million people living with a disability as a result of a TBI, and each year an additional 1.7 million Americans sustain a TBI. These injuries have both short- and long-term effects on health, ranging from symptoms that have a minimal interference on lifestyle, through to physical, emotional, and psychosocial changes that may interfere with daily activities. As well as the burden to the individual, brain injuries also have an annual economic burden of more than US$60 billion, due to both direct and indirect costs, such as loss of productivity. The reasons for the injuries depend on the age of the individual; for example, more than one-third of brain injuries in the United States are due to people falling, which is the leading cause of TBI among the elderly, whereas transportation-related brain injuries are the leading cause for individuals aged 15 to 24 years.
In recent years, the media have had a strong focus on the long-term outcomes of mild TBI (mTBI) or concussions, such as that which may occur to American football players and military personnel. Pathophysiologic investigations on the brains of these individuals have revealed Alzheimer-like symptoms, termed chronic traumatic encephalopathy (CTE). Although this disease has been known to occur in boxers since the 1960s (with numerous names, including dementia pugilistica and punch-drunk syndrome), the recent media attention has highlighted the high prevalence of this disease in other athletic arenas. This attention was followed by the Obama Administration releasing an Executive Order in 2012 to direct the Departments of Defense, Veterans Affairs, Health and Human Services, and Education to develop a National Research Action Plan (NRAP) on TBI, posttraumatic stress disorder (PTSD), and other common comorbidities. The NRAP was released in August 2013 and aims to (1) accelerate the understanding of the causes and mechanisms of TBI, PTSD, and other common comorbidities; (2) develop clinical innovations to detect disorders early and accurately; and (3) develop proven means to prevent and treat the devastating conditions caused by the injuries. To this end, understanding the acute and chronic pathophysiology of TBI is critical to determining the risk factors for individuals to develop symptoms as well as develop therapeutic innovations and interventions.
Given that current clinical imaging techniques do not detect minor changes in the human brain following injury, much of the knowledge on the cellular sequelae in the injured brain is based on rodent studies. To confirm these findings in the injured human brain, on arrival at the emergency department, an individual can be assessed for physical and cognitive functions, have a computed tomographic (CT)/MRI scan performed, have their intracranial pressure and cerebral blood flow measured, and possibly have bio-specimens collected for analysis of protein and messenger RNA expression. Subsequently, on autopsy, brain samples can be collected and analyzed.
Mild traumatic brain injury
Causes, Diagnosis, and Symptoms
A mild brain injury is often caused by a blunt head trauma, and/or acceleration or deceleration forces to the head, and refers to the initial impact of the injury. The diagnosis of an mTBI is the subject of constant debate because no single test is available to definitively confirm the diagnosis. Currently, a combination of tools is used, including history of the injury, patient symptoms, and CT scans. Although most patients with an mTBI recover within weeks to months of the injury without specific intervention, 30% to 53% of affected individuals may still have disabling symptoms at least 1 year after the injury, and this is often referred to as postconcussive syndrome. Because by definition mTBI is nonfatal and does not alter life expectancy (0.1% mortality ), prolonged symptoms can lead to life-long disabilities. Thus, the term mild brain injury can be misleading, because it does not refer to the severity or time course of injury symptoms.
In the acute period following an mTBI, an individual may experience a brief loss of consciousness (or altered consciousness), transient confusion, disorientation, loss of memory at the time of the injury (amnesia), and other neurologic and neuropsychological dysfunctions, including seizures, headaches, dizziness, irritability, fatigue, and poor concentration. More specifically, diagnosis is based on the Glasgow Coma Scale (GCS) that ranks functional ability from 1 (worst outcome) to 15 (best outcome), with a mild injury defined between 13 and 15. Subsequently, these symptoms may evolve into persistent low-grade headaches, pain, poor attention and concentration, fatigue, anxiety, and depression, all or some of which may continue for months to years following the injury.
Pathophysiology
After a violent hit to the head, the soft brain inside hits the intracranial surface of the skull, which may damage the area of the brain that comes in contact with the skull. This damage may occur in both the forward and the reverse locations as the brain “bounces” within the skull. In American football, which has a high incidence of mild repetitive TBIs, most of these impacts are on the side of the helmet, or from ground contact to the back of the head, whereas the location of impact from concussions not related to football are difficult to document.
In addition to the brain hitting against the cranium, any rotational movement to the brain following the impact may stretch, and sometimes tear, axons within white matter tracts of the brain, which is known as “diffuse axonal injury.” Although traditional CT scans are unable to detect these injuries, modern imaging techniques such as altered fractional anisotropy using diffusion tensor imaging and MRI show promise to detect these changes. Using rodent models, there is recent evidence to suggest this type of injury may impair neuronal function, although the causes of this dysfunction are currently unclear. Some research suggests diffuse axonal injury may induce neuronal degeneration as evidenced by increased Fluoro-Jade staining, whereas other research suggests it may only induce neuronal atrophy due to axotomy of the axon initial segment. For the latter, 2 types of axonal abnormality have been observed. The first is progressive swellings along the axon termed “bulbs” that eventually lead to axonal disconnection and loss of function. The second is a production of axonal varicosities and is thought to be due to microtubule breakage, thus slowing axonal transport to cause the varicosities. It has been proposed these abnormalities may lead to delayed secondary disconnection and/or prolonged neuronal dysfunction.
In addition to these neuronal morphologic and functional alterations, a mild injury may also induce other pathophysiologic responses. Following mild injury, there is frequently a sustained proinflammatory cytokine upregulation, a reduction in oligodendrocyte cell numbers, and glial reactivity. Furthermore, a mild injury may also disrupt the function of the cerebral vasculature. In a healthy brain, cerebral blood flow is tightly controlled to provide an adequate supply of oxygen and nutrients through a dense network of arteries and capillaries. Following a traumatic injury, there is an initial reduction in cerebral blood flow (within hours of the injury), which can remain low for days, depending on injury severity. This reduced blood flow could be attributed to increased nitric oxide expression after injury, causing vasodilation instead of a pressure-induced increase in vasoconstriction. These changes are also associated with a reduction in blood vessel density in the perilesional region during the first few days after injury. Over the next few days to weeks, there is usually a return of normal cerebral blood flow, which coincides with an increase in blood vessel density in the affected region. Similar to the uninjured brain, the constant supply of blood then needs to be maintained, and this occurs by cerebral vessels undergoing vasodilation in response to dilatory stimuli, termed cerebrovascular reactivity. Unfortunately, following a brain injury, the cerebral blood vessels may be less able to respond to dilatory stimuli, and this can lead to a poor prognosis, including a terminal event.
In addition to the immediate effects of axonal injury and cerebral vascular dysregulation, in recent years there has been much attention paid to the discovery of CTE in athletes and some Veterans, whom have undergone repetitive mild brain injuries throughout their careers. This disease is a form of tauopathy and is characterized by unusually high levels of tau deposition occurring as neurofibrillary tangles. CTE has been classified into 4 clinical stages ranging from perivascular tangles in the initial stages of the disease, through to widespread tau deposition decades later. Because only individuals with repetitive mild injuries appear to develop CTE, it is thought that this type of injury does not simply represent a milder form of moderate to severe TBI, but rather it represents a separate type of injury that can manifest to a different disease endpoint. However, unlike other neurodegenerative diseases such as Alzheimer, the development of CTE symptoms often occurs earlier in life and leads to an altered neuropathology. Unfortunately, at this moment, definitive diagnosis of the disease can only be performed on postmortem analysis, although current technological advances with PET imaging may soon be used to detect tau accumulation in living individuals. At present, the risk factors for developing CTE are under investigation.
Moderate and severe traumatic brain injury
Causes, Diagnosis, and Symptoms
According to the Centers for Disease Control and Prevention, the most common causes of a moderate or severe TBI are falls (40.5%), motor vehicle accidents (14.3%), assaults (10.7%), or being struck by or against (15.5%). Moderate TBIs are diagnosed by a possible loss of consciousness lasting up to a few hours, confusion lasting from days to weeks, and physical, cognitive, and/or behavioral impairments lasting for months, or are sustained permanently, with a GCS score between 9 and 12. In comparison, a severe brain injury is determined by a prolonged unconscious or vegetative state that can last for days to months, with a GSC score of less than 9. Following a severe injury, patients may experience physical limitations (eg, headaches, nausea/vomiting, pupil dilation, slurred speech, aphasia, sensory deficits), along with cognitive (eg, memory, attention, concentration) and emotional (eg, motivation, irritability, aggression) dysfunctions. Although a patient with a moderate brain injury has the potential to make a good recovery through treatment, and/or learning to modify their behavior to compensate for any acquired deficits, a patient with a severe injury has the possibility of remaining permanently unresponsive. Therefore, a risk factor for subsequent symptoms is injury severity, but also includes the location of the brain injury as well as the individual’s age and gender.
Pathophysiology
Similar to a mild injury, a moderate or severe brain injury may cause the brain to hit against the intracranial portion of the skull, with or without penetration (from either skull fragments or foreign objects). As indicated above, the location and severity of the impact, as well as the depth and amount of brain penetration, likely have a significant impact on the patient’s outcome. Any penetration of the brain’s fragile structure can mechanically tear apart neurons and shear their axons to disrupt neuronal circuitry as well as damage the vasculature, allowing movement of blood and leukocytes into the normally immune privileged brain. These effects have an immediate impact on the brain by inducing necrotic cell loss as well as inducing apoptosis of the surrounding cells. Within minutes of these changes, a local inflammatory response occurs whereby astrocytes and microglia secrete proinflammatory cytokines, such as tumor necrosis factor, interleukin-6, and interleukin-1β, into the perilesional region. These proinflammatory cytokines mobilize immune and glial cells to the injury site, causing edema and further inflammation. This phase is associated with gliosis, demyelination, and continued apoptosis. Simultaneously, the cerebral vasculature is also undergoing further changes. Although there is some discrepancy within the literature, hypoperfusion may occur acutely after injury, likely caused by reduced blood pressure, impaired vasodilation (vascular reactivity), and elevated intracranial pressure. Over the next few days, this may be followed by hyperemia, after which hypoperfusion may return and be accompanied by vasospasms. At the same time, there may also be a reduction in the cerebral metabolic rate for oxygen, suggesting impairments in energy metabolism; however, there are discrepancies in the literature on this matter, and it is unclear how much this may play a role in each patient’s injury pathophysiology.
Blast-induced traumatic brain injury
Causes, Diagnosis, and Symptoms
A blast TBI (bTBI) is a unique subtype of traumatic injury that occurs due to direct or indirect exposure to an explosion, primarily in combat situations. A “blast wave” generated from an explosion consists of a front of high pressure that quickly compresses the surrounding air, giving rise to negative pressure, but which rapidly expands and displaces an equal volume of air. This displacement of air then generates a “blast wind.” The Centers for Disease Control and Prevention have identified 4 types of injuries caused by exposure to a blast based on how the injury occurred. These injuries include injuries from the initial blast pressure wave (primary blast), penetrating or blunt injuries from flying debris and fragments, and injuries from individuals being thrown by the blast wind. Although blast injuries are typically polytraumatic, here the focus is entirely on the effects of the pressure wave, because other aspects of the injury, such as penetration of the brain from flying debris, are covered in other sections of this review.
In recent years, retrospective studies have identified that approximately half of combat-related injuries sustained during the conflicts in Iraq and Afghanistan are due to explosions, of which up to 15% of the affected service members experienced a primary blast injury, although most are likely polytraumatic in nature. The Centers for Disease Control and Prevention have identified that the severity of symptoms depends on factors such as the type of explosive used, the surrounding environment, the distance between the individual and the blast, and the presence of any protective or hazardous items between the individual and the blast. A retrospective study on service members shows an increased severity of the blast injury is associated with an increase in disability upon discharge. In support of this, rodent data have revealed a dose-response relationship between blast intensity and behavioral deficits. Diagnosis of whether the blast injury has caused a mild, moderate, or severe brain injury may include evaluation of the length of unconsciousness and GCS outcome, along with identifying any loss of memory at the time of the event, as well as any alteration in mental state. Similar to a nonblast civilian mTBI, a blast injury may commonly cause a concussion, with the individual experiencing physical, emotional, and/or cognitive difficulties. However, individuals with a blast-related concussion are likely to also experience other comorbid conditions, such as depression or PTSD.
Pathophysiology
The blast wave caused by an explosion may interact with the brain in several ways. First, as the kinetic energy passes through the skull, it may directly induce acceleration or rotation of the brain, causing diffuse axonal injury and subsequent secondary injury mechanisms. Although these types of injuries may at first appear similar to nonblast civilian TBI, recent data suggest the type of axonal injury caused by a blast is unique to bTBI. Diffusion tensor imaging of military service members following brain injury indicates the axonal damage from bTBI is more widespread and spatially variable compared with nonblast civilian TBI and includes brain regions such as the superior corona radiata of the frontal cortex, the cerebellum, and optic tracts. In support of this, recent data from rodent models of bTBI, which induce similar behavioral outcomes to patients, also show that rotational brain injury produces a different set of behaviors compared with blast-induced neurotrauma. Therefore, bTBI-induced axonal damage may need to be considered a separate type of diffuse axonal injury. Comparatively, the secondary mechanisms of injury produced from a bTBI appear to be similar to non-blast TBI, whereby a robust inflammatory response occurs that includes increased proinflammatory cytokines expression and glial reactivity. In addition to these effects, edema and vasospasms are also far more prevalent following bTBI, compared with nonblast civilian mTBI, which has been proposed to be due to narrowing of primary arteries, and are supported by reduced vascular integrity following a blast injury in rodents, thus providing support for a vascular-related mechanism of tissue damage.
In addition to the direct effects of a blast wave on the brain tissue, the blast wave may also act indirectly through 2 possible mechanisms. First, the blast may cause gas-containing compartments in the brain to compress, and subsequently expand, leading to damage of surrounding tissues. Second, the blast wave may also cause shock waves in the blood or cerebrospinal fluid to be delivered to the brain within seconds of the impact. This shock wave can lead to an acceleration of the elements of the tissue from their resting state to a rate dependent on the density of the medium, which may subsequently cause deformation and/or rupture of the affected brain tissue.
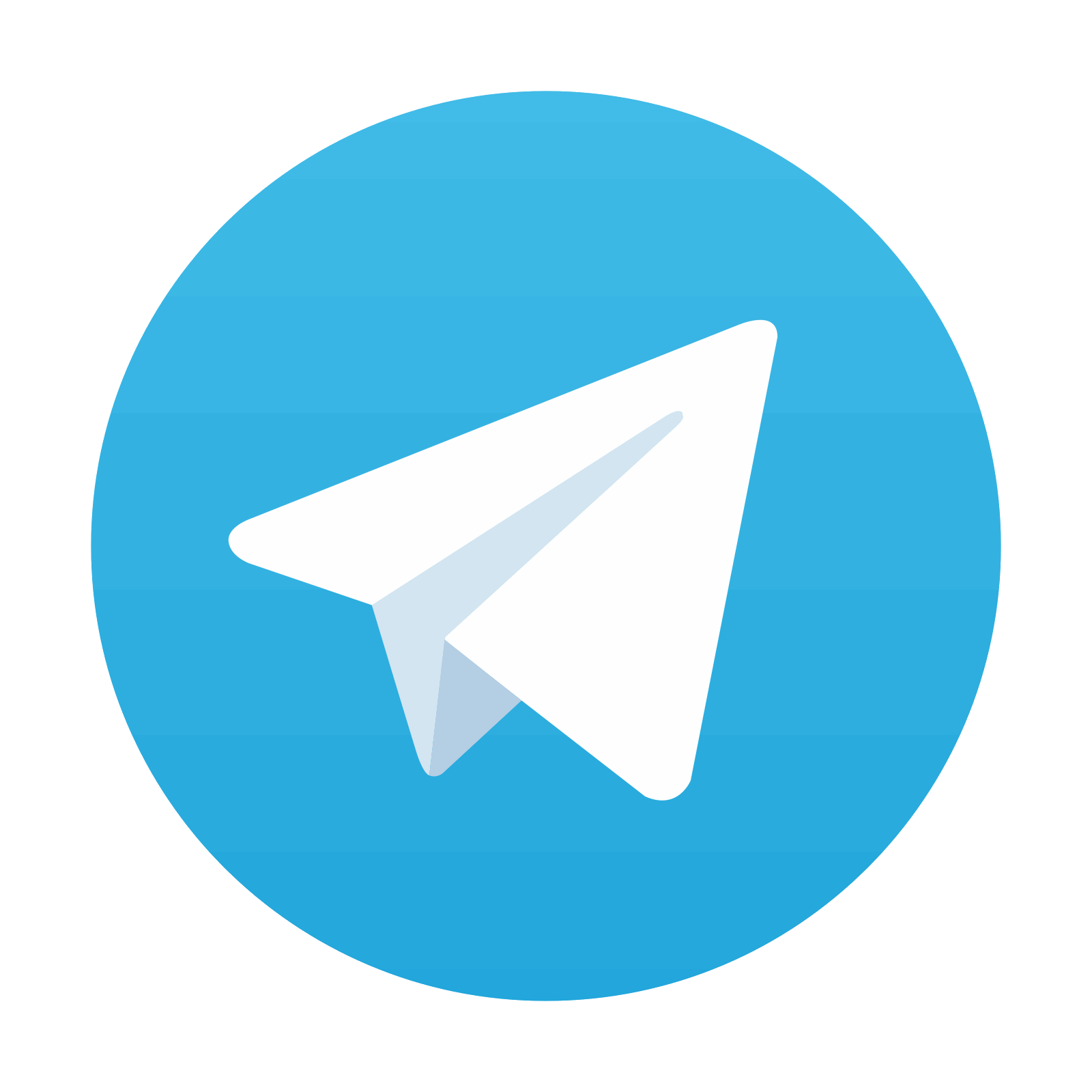
Stay updated, free articles. Join our Telegram channel

Full access? Get Clinical Tree
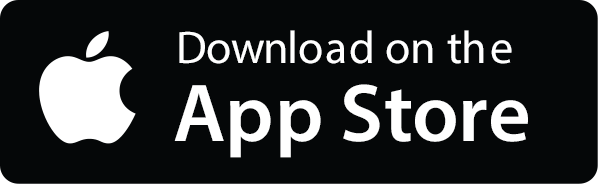
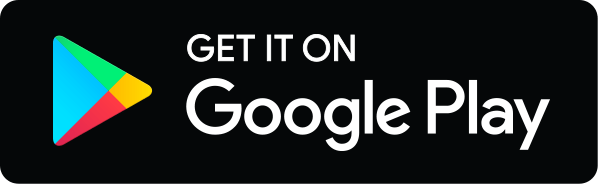