The outcome of patients suffering from spondyloarthritis is determined by chronic inflammation and new bone formation leading to ankylosis. The latter process manifests by new cartilage and bone formation leading to joint or spine fusion. This article discusses the main mechanisms of new bone formation in spondyloarthritis. It reviews the key molecules and concepts of new bone formation and ankylosis in animal models of disease and translates these findings to human disease. In addition, proposed biomarkers of new bone formation are evaluated and the translational current and future challenges are discussed with regards to new bone formation in spondyloarthritis.
- •
Chronic inflammation and new bone formation, specifically progressive ankylosis, determine the burden of disease and outcome of patients with spondyloarthritis.
- •
Insights into the cellular and molecular mechanisms that contribute to ankylosis in spondyloarthritis (SpA) are linked to better understanding of the key players in skeletal development and homeostasis.
- •
The paradox of concurrent ankylosis and osteoporosis in close proximity highlights the hypothesis that the mechanisms of new bone formation are different from those involved in the normal bone remodeling cycle.
- •
Different pathways, including bone morphogenetic proteins (BMPs), Wnt, and hedgehog proteins, have been studied in animal models and could become new therapeutic targets.
- •
Biomarkers for bone formation are potential tools for predicting structural bone changes in SpA but have specific challenges because changes in local as well as systemic bone homeostasis affect their serum levels.
- •
The relationship between inflammation and ankylosis remains controversial. Long-term data of therapeutic interventions as well as the effects of treatment in early disease stages may provide better insights into this matter.
Introduction
The iconic image of a fully ankylosed spine in patients with ankylosing spondylitis (AS) represents the final consequence of a chronic inflammatory process and the threatening prospect for patients who have recently been diagnosed with SpA. All subtypes of SpA, including AS, psoriatic arthritis, inflammatory bowel disease–associated arthritis, reactive arthritis, juvenile SpA, and undifferentiated SpA, are characterized by inflammation and structural damage to the skeleton. Damage presents as new bone formation leading to bony fusion (ankylosis) of the sacroiliac joints, syndesmophyte formation eventually bridging the intervertebral spaces, and enthesophytes originating from tendon or ligament insertion sites in the peripheral skeleton ( Fig. 1 ).

For most patients with SpA, the burden of disease results from a combination of inflammation and structural bone changes. The inflammatory process manifests by osteitis, enthesitis, and synovitis and may clinically appear as pain, stiffness, local swelling, warmth, redness, and loss of function. Structural bone changes not only affect patients by causing disability and permanent loss of function but also with secondary effects; thus, ankylosis changes the balance of loads and forces in the skeletal system, leading to muscle stiffness and accelerated degenerative spine disease. For instance, ankylosis of the sacroiliac joints has only a limited impact on the direct mobility of the pelvis but changes the biomechanical loads in the spine because vertical forces can no longer be translated toward the limbs in the optimal way.
Clinicians and researchers face several challenges with respect to investigating the mechanism, kinetics, prediction, and impact of new bone formation and ankylosis in SpA. In general, ankylosis is a slow process but has a considerable and often underestimated individual variability. Analyses of progression of ankylosis in cohort studies and clinical trials, therefore, usually require long-term sequential observations. Also, the assessment of ankylosis is challenging due to the complexity of scoring systems and the challenge of whether such scoring systems detect not only statistically but also clinically meaningful changes. In addition, access to human tissue from the spine for pathology studies is limited. As discussed later, different animal and in vitro models have been used to understand cellular and molecular events leading to ankylosis and the relationship between inflammation and new tissue formation. None of these animal models, however, exactly mimics human SpA and the direct clinical relevance of the available models is sometimes heavily debated.
Fundamentals of bone formation
To understand pathologic new bone formation in SpA, it is necessary to consider the basic mechanisms of skeletal development and turnover ( Fig. 2 ). New bone formation leading to ankylosis is a process in which the new skeletal tissue is always formed in connection with existing bone but extends beyond its original border. For this feature the term, osteoproliferation , has been introduced, but it may not well reflect the different cellular and molecular processes involved. The processes involved in new bone formation in SpA are orchestrated by proliferation, differentiation, maturation, and migration of cells as well as cell death. Osteproliferation in SpA is a complex tissue remodeling process rather than simple proliferation of bone and shares similarities with joint remodeling in osteoarthritis.

Bone remodeling in SpA should be distinguished from classical bone remodeling, which is the continuous renewal of the skeleton by the balanced activity of bone-forming osteoblasts and bone-resorbing osteoclasts, which is orchestrated by the mechanosensitive osteocytes. The distinction between this physiologic process and pathologic bone formation becomes particularly clear in SpA: whereas new bone is excessively built up at the outer surface of the cortical bone, SpA patients paradoxically develop osteoporosis due to degradation of the trabecular bone of the vertebral bodies. These diverging remodeling types in the cortical and the trabecular bone of patients with SpA suggest that pathologic bone remodeling in SpA is fundamentally different from classical bone turnover. Moreover, control of inflammation reverts the trabecular bone loss but does not seem to affect the progression of ankylosis.
Therefore, pathologic bone formation in SpA can be best understood from its parallels with bone development and growth, the so-called bone modeling (in contrast to remodeling) process. Two different types of bone formation have been extensively described and cooperate to skeletal development and growth: (1) endochondral bone formation defines the skeletal elements through an intermediate cartilage stage, whereas (2) membranous bone formation results in direct new bone formation. The former process builds up most of the skeletal elements, in particular in the appendicular skeleton, whereas the latter is important for the calvarial bones but also contributes to later stages of the development of long bones. In endochondral bone formation, skeletal progenitor cells first form cell condensations at the prospective sites (so-called anlagen) and thereby trigger a cascade of chondrogenic differentiation processes. Thus, mesenchymal progenitor cells first commit toward chondrocytes and start producing collagen type II and extracellular matrix proteoglycans, like aggrecan. Starting off as proliferating chondrocytes, they subsequently become prehypertrophic chondrocytes and finally terminally differentiated hypertrophic chondrocytes. These cells express vascular endothelial growth factor and matrix metalloproteinase (MMP) 13 and produce a different type of extracellular matrix, typically rich in type X collagen. The proangiogenic factors attract blood vessels and other progenitor cells, which allow breakdown of the calcified matrix and their replacement by bone based on the influx of osteoblast precursors. Typically, such ossification centers are found on both sides of the long bones and shape into the growth plates. As the skeletal element grows and develops its specific shape, a bony collar is formed at the borders of the bone through membranous bone formation.
Fundamentals of bone formation
To understand pathologic new bone formation in SpA, it is necessary to consider the basic mechanisms of skeletal development and turnover ( Fig. 2 ). New bone formation leading to ankylosis is a process in which the new skeletal tissue is always formed in connection with existing bone but extends beyond its original border. For this feature the term, osteoproliferation , has been introduced, but it may not well reflect the different cellular and molecular processes involved. The processes involved in new bone formation in SpA are orchestrated by proliferation, differentiation, maturation, and migration of cells as well as cell death. Osteproliferation in SpA is a complex tissue remodeling process rather than simple proliferation of bone and shares similarities with joint remodeling in osteoarthritis.
Bone remodeling in SpA should be distinguished from classical bone remodeling, which is the continuous renewal of the skeleton by the balanced activity of bone-forming osteoblasts and bone-resorbing osteoclasts, which is orchestrated by the mechanosensitive osteocytes. The distinction between this physiologic process and pathologic bone formation becomes particularly clear in SpA: whereas new bone is excessively built up at the outer surface of the cortical bone, SpA patients paradoxically develop osteoporosis due to degradation of the trabecular bone of the vertebral bodies. These diverging remodeling types in the cortical and the trabecular bone of patients with SpA suggest that pathologic bone remodeling in SpA is fundamentally different from classical bone turnover. Moreover, control of inflammation reverts the trabecular bone loss but does not seem to affect the progression of ankylosis.
Therefore, pathologic bone formation in SpA can be best understood from its parallels with bone development and growth, the so-called bone modeling (in contrast to remodeling) process. Two different types of bone formation have been extensively described and cooperate to skeletal development and growth: (1) endochondral bone formation defines the skeletal elements through an intermediate cartilage stage, whereas (2) membranous bone formation results in direct new bone formation. The former process builds up most of the skeletal elements, in particular in the appendicular skeleton, whereas the latter is important for the calvarial bones but also contributes to later stages of the development of long bones. In endochondral bone formation, skeletal progenitor cells first form cell condensations at the prospective sites (so-called anlagen) and thereby trigger a cascade of chondrogenic differentiation processes. Thus, mesenchymal progenitor cells first commit toward chondrocytes and start producing collagen type II and extracellular matrix proteoglycans, like aggrecan. Starting off as proliferating chondrocytes, they subsequently become prehypertrophic chondrocytes and finally terminally differentiated hypertrophic chondrocytes. These cells express vascular endothelial growth factor and matrix metalloproteinase (MMP) 13 and produce a different type of extracellular matrix, typically rich in type X collagen. The proangiogenic factors attract blood vessels and other progenitor cells, which allow breakdown of the calcified matrix and their replacement by bone based on the influx of osteoblast precursors. Typically, such ossification centers are found on both sides of the long bones and shape into the growth plates. As the skeletal element grows and develops its specific shape, a bony collar is formed at the borders of the bone through membranous bone formation.
Bone formation in spondyloarthritis
The limited histology data available from SpA patients suggest that both endochondral and membranous bone formation seem to contribute to ankylosis. Nevertheless, these processes appear less well orchestrated and structured than those during development and normal growth. Thus, so-called cartilage metaplasia, in which cartilage cells are found in a calcified matrix, was also identified in bone samples from patients with SpA. Although formal proof is lacking, it seems likely that in SpA, joint-associated or spine-associated progenitor populations commit toward differentiation into either chondrocytes or osteoblasts. The multilineage potential of cell types, such as synovial, periosteal, and bone marrow cells, which are involved in SpA, has been demonstrated. Nevertheless specific influx of already commited cells, such as fibrocytes and pericytes, as well as local endothelial-mesenchymal transition cannot be excluded as contributing mechanisms.
Molecular signaling pathways in bone development
The developmental processes (described previously) are steered by several molecular pathways, including BMPs, Wnt proteins, hedgehog proteins, and fibroblast growth factors. BMPs, Wnts, and hedgehogs have been studied in animal models of arthritis and in patient samples (discussed later) ( Fig. 3 ).
BMPs were originally identified as protein factors isolated from bone matrix that can ectopically induce a full cascade of endochondral bone formation, when injected intramuscularly or subcutaneously in vivo. These potent morphogens were subsequently demonstrated to play important roles not only in skeletal development but also in other organs systems and in early stages of development. Increasing evidence suggests that BMPs have homeostatic or regulatory roles in different organ systems, including the skeleton (bone and cartilage), the kidney, and the endocrine system postnatally. BMPs are currently used in the clinic for the treatment of nonunion fractures and to obtain spinal ankylosis in patients with intervertebral disk degeneration.
BMPs consist of a large family of ligands that can be divided into different subgroups. The numerous ligands and promiscuous receptor associations that are leading to the activation of distinct signaling cascades allow BMPs to have wide-ranging effects on distinct cell types. The best-known downstream signaling event is activation of Smad proteins and activation of mitogen-activated protein kinases (MAPKs), such as p38 and ERK. In addition, regulatory mechanisms, including extracellular and intracellular antagonists, and complex association of coactivators and corepressors at the level of transcription are essential for the fine-tuning of this signaling system. In skeletal development, BMPs play an essential role in the early phases of chondrogenesis by stimulating chondrogenic differentiation as well as in the late hypertrophic stages. Most of these effects seem mediated by Smad signaling.
Wnt proteins were originally identified in cancer research and fruit fly development and much like BMPs direct early developmental processes as well as postnatal tissue homeostasis. The Wnt family also mediates its effects by using complex ligand-receptor interactions as well as activation of different intracellular signaling cascades. Canonical Wnt signaling primarily involves β-catenin translocation to the nucleus. Other downstream events include increased calcium signaling and direct effects on the cytoskeleton and cell polarity. In skeletal development and biology, Wnts have stimulatory roles on bone formation and their expression seems regulated by the mechanosensing osteocytes. Wnt stimulate new bone formation by direct effects on osteoblasts. Moreover, Wnt proteins tightly regulate articular cartilage homeostasis.
Hedgehog proteins provide an essential feedback loop in bone development. In combination with parathyroid hormone–related protein, Indian hedgehog defines the rate of chondrocyte proliferation and hypertrophy. Indian hedgehog binds to the Smoothened (SMO) and Patched (PTCH) receptor complex and leads to an activation of the Gli transcription factors. Gli1 and Gli2 are considered transcriptional activators, whereas Gli3 acts as a transcriptional repressor.
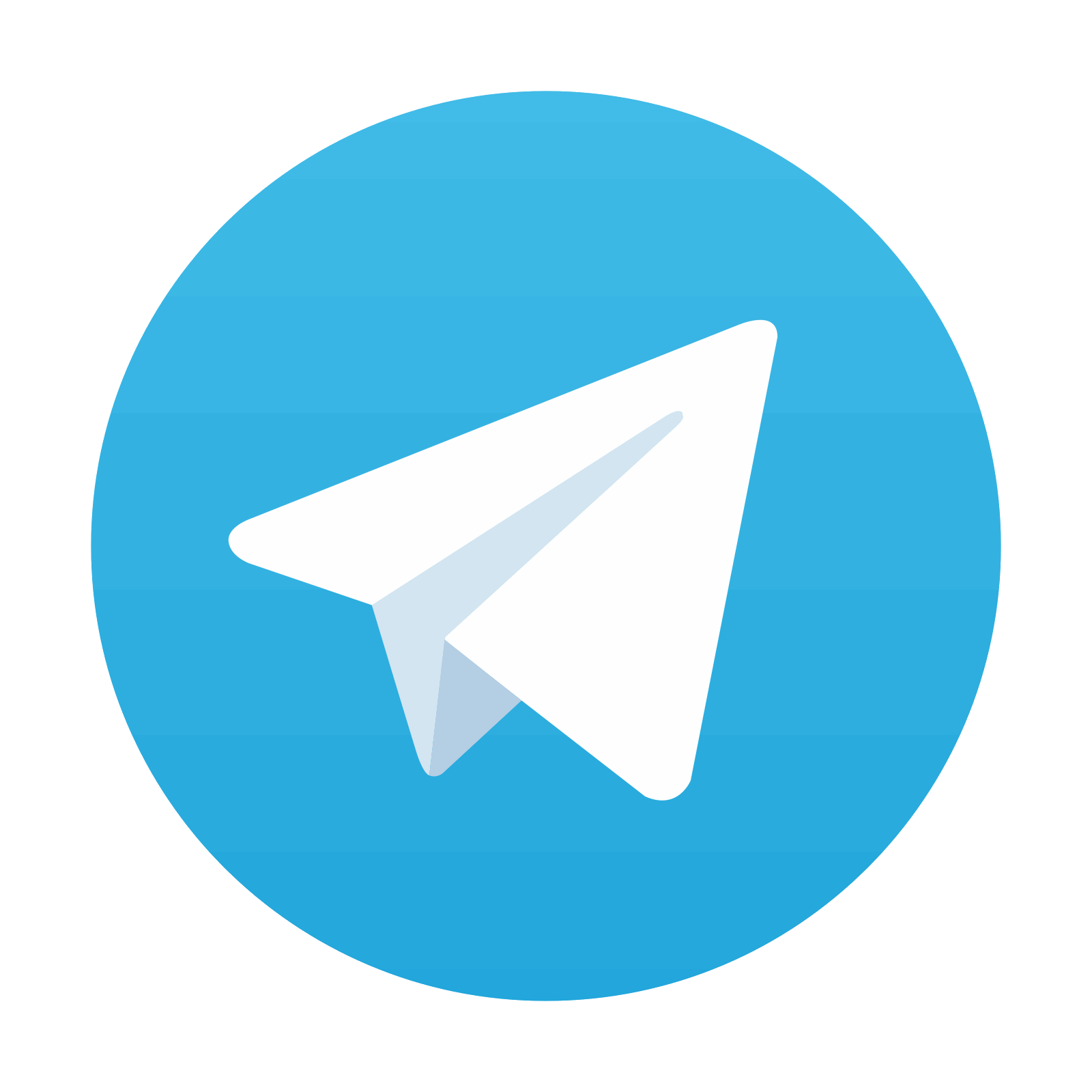
Stay updated, free articles. Join our Telegram channel

Full access? Get Clinical Tree
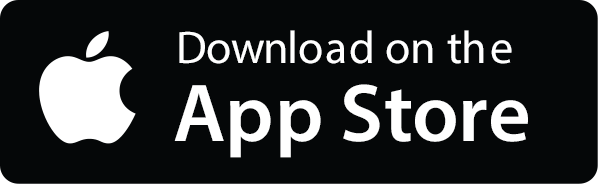
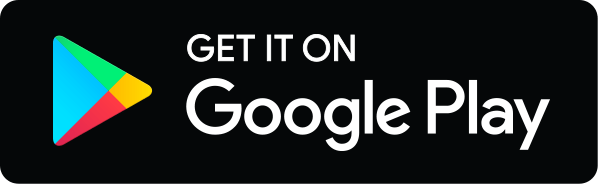