Patients with cerebral palsy present with a variety of adaptations to muscle structure and function. These pathophysiologic symptoms include functional deficits such as decreased force production and range of motion, in addition to changes in muscle structure such as decreased muscle belly size, increased sarcomere length, and altered extracellular matrix structure and composition. On a cellular level, patients with cerebral palsy have fewer muscle stem cells, termed satellite cells, and altered gene expression. Understanding the nature of these changes may present opportunities for the development of new muscle treatment therapies.
Key points
- •
Muscle from patients with cerebral palsy shows functional deficits such as decreased force production and range of motion.
- •
Muscle is altered at a structural level, with decreased muscle body size, smaller-diameter fibers, and highly stretched sarcomeres (the force-producing unit of muscle).
- •
Muscle from patients with cerebral palsy has altered extracellular matrix and connective tissue.
- •
Decreased muscle stem cell numbers and altered gene expression have been reported in cerebral palsy.
Introduction
Nature of the Problem
Cerebral palsy (CP) is a motor disorder caused by a nonprogressive injury to the developing brain. The injury occurs perinatally and, though causes are rarely known, CP is common in infants born preterm with small birth weights. CP occurs in 2 to 3 of every 1000 live births and has heterogeneous symptoms, anatomic involvement, and functional impairment, including lifelong changes in motor function. These alterations stem from both changes in the neural drive to muscles and changes to muscles themselves.
Symptoms
Spastic CP, which involves injury to the pyramidal system, is the most common form of CP, making up nearly 75% of all cases. Spasticity has been defined as a “velocity dependent resistance to stretch.” Limb involvement varies, with patients showing symptoms in either all 4 limbs (tetraplegia or quadriplegia), primarily on one side of the body including one upper and lower extremity (hemiplegia), or primarily in the lower extremities (diplegia). Patients’ functional mobility can be classified using several rating scales, including the Gross Motor Function Classification System (GMFCS), which rates patient mobility on a scale of 1 to 5 from high to low function, respectively. Although the injury associated with CP initially occurs in the developing brain, symptoms are commonly treated at the muscle level. Because the population affected with CP is large and heterogeneous, a better understanding, especially among clinicians and therapists, of muscular adaptations in CP may lead to improvements in treatment or even development of completely novel therapeutic strategies.
To understand the adaptations that occur in muscle from CP patients, it is important to review the function of typically developing muscle.
Introduction
Nature of the Problem
Cerebral palsy (CP) is a motor disorder caused by a nonprogressive injury to the developing brain. The injury occurs perinatally and, though causes are rarely known, CP is common in infants born preterm with small birth weights. CP occurs in 2 to 3 of every 1000 live births and has heterogeneous symptoms, anatomic involvement, and functional impairment, including lifelong changes in motor function. These alterations stem from both changes in the neural drive to muscles and changes to muscles themselves.
Symptoms
Spastic CP, which involves injury to the pyramidal system, is the most common form of CP, making up nearly 75% of all cases. Spasticity has been defined as a “velocity dependent resistance to stretch.” Limb involvement varies, with patients showing symptoms in either all 4 limbs (tetraplegia or quadriplegia), primarily on one side of the body including one upper and lower extremity (hemiplegia), or primarily in the lower extremities (diplegia). Patients’ functional mobility can be classified using several rating scales, including the Gross Motor Function Classification System (GMFCS), which rates patient mobility on a scale of 1 to 5 from high to low function, respectively. Although the injury associated with CP initially occurs in the developing brain, symptoms are commonly treated at the muscle level. Because the population affected with CP is large and heterogeneous, a better understanding, especially among clinicians and therapists, of muscular adaptations in CP may lead to improvements in treatment or even development of completely novel therapeutic strategies.
To understand the adaptations that occur in muscle from CP patients, it is important to review the function of typically developing muscle.
Healthy skeletal muscle structure and function
Muscle Structure
The fundamental unit of muscle force production is the sarcomere. Sarcomeres produce force by the interaction between 2 proteins, actin and myosin. Force production is affected by both muscle velocity and the amount of overlap between these 2 proteins, or sarcomere length. The sarcomere length-tension relationship has been characterized in the length-tension curve. Sarcomeres are joined end to end (in series) to form myofibrils. Bundles of myofibrils form myofibers, or multinucleated muscle cells. These muscle fibers are joined into muscle fiber bundles, or fascicles ( Fig. 1 ).
At each increasing size scale, extracellular matrix (ECM), the surrounding connective tissue, encapsulates muscle structures. Endomysium surrounds individual myofibers, perimysium surrounds muscle fascicles, and epimysium surrounds the whole muscle (see Fig. 1 ). The composition and arrangement of these structures is important to muscle function, and can vary in muscle disorders.
The extensive growth and regeneration capacity seen in muscle is due to its intrinsic stem cell population. Most of these stem cells are called satellite cells and are found below the basal lamina of myofibers; they are normally quiescent except when activated during times of muscle disease or injury. Satellite cell number and viability, rather than being constant throughout life, decreases with age or diseases that are characterized by extensive regeneration. Conditions such as muscular dystrophy, which require constant regeneration of muscle fibers, are believed to eventually lead to exhaustion of the satellite cell population and the concomitant loss in a muscle’s ability to adapt to the new functional demand.
Plasticity
Muscle has strong regenerative capacity, and can respond and change based on functional demands; for example, muscle fibers atrophy (leading to a decrease in muscle fiber size) when subject to decreased use, aging, and some diseases. Serial sarcomere number can also change in response to growth as well as limb immobilization with the muscle in a shortened or lengthened position. This serial change in sarcomere number resulting from chronic change in muscle length was shown in several classic studies in both mouse and cat. These muscles, immobilized in a shortened position, rapidly adjust their sarcomere number to restore sarcomere length to previous values. A similar response was reported in a human case study of distraction osteogenesis in which a leg-length discrepancy was corrected as a patient’s bone was gradually lengthened over time. Sarcomere lengths were measured and sarcomere number calculated over the course of the treatment. As stretching of the bone and muscle occurred, sarcomere number rapidly increased and sarcomere length nearly returned to the pretreatment value. Serial sarcomere number from patients with CP appears to be altered in comparison with typically developing muscles, however, suggesting that the plasticity seen in typically developing muscles may not be present to the same extent in CP.
Muscle pathology of cerebral palsy
Force Production and Muscle Function
Alterations in gait, balance, and force production have been reported for patients with CP. For example, knee extensor force decreases with CP, which can significantly inhibit mobility. Voluntary force production in general is decreased, as shown by many investigators. There is also evidence that greater cocontraction, or simultaneous activation of a muscle and its antagonist, occurs in CP. Compounding the problem of decreased force production, ankle stiffness was also shown to be 51% higher in CP, indicating increased resistance to passive ankle flexion.
Muscle Architecture
Muscles in spastic CP often develop contractures, whereby joint range of motion is limited and muscles appear functionally “short.” Many researchers have measured changes in muscle properties such as muscle belly size, muscle length, fascicle length, and sarcomere length, all of which may help to explain this observation. Ultrasonography is probably the most common tool used to describe basic muscle structural changes, such as fiber length and tissue thickness ( Fig. 2 ). Previous architecture studies focused primarily on the gastrocnemius muscle, an ankle plantarflexor and knee flexor that is commonly implicated in ankle equinus contractures and also plays a role in knee flexion contractures. Ultrasonographic measurements show that gastrocnemius muscle volume is smaller in patients with CP. When affected and unaffected limbs were compared in hemiplegic patients, muscle volume was decreased by 28% in the affected gastrocnemius and by nearly 50% more than muscles of typically developing children. Muscle belly lengths have also been shown to decrease in CP. One 3-dimensional ultrasonography study of the medial gastrocnemius showed decreased muscle belly lengths, but no change in fascicle length when normalized to tibia length. Based on the description of muscle structure presented herein, it is clear that ultrasonography alone cannot make functional predictions based on gross tissue dimensions because the composite sarcomeres cannot be detected using this modality.
In contrast to what might be expected for contractures, which are often considered permanently contracted or shortened muscles, previous fascicle length measurements in patients with CP have been inconclusive. Whereas some studies report shorter muscle fascicles in CP, others report no difference between typically developing and CP fascicle lengths. In contrast to the variety of results reported for fascicle length, sarcomere length, the best predictor of active muscle force, has been consistently shown to be longer in CP patients ( Fig. 3 ). Whereas one study questioned the functional significance of sarcomere lengthening based on force measurements across the joint range of motion, previous direct studies of sarcomere length show long sarcomeres in CP in both upper and lower extremity flexors. It therefore appears that regulation of sarcomere length does not occur similarly in patients with typical development and those with CP, as CP sarcomeres do not maintain a relatively constant length as in normal development.
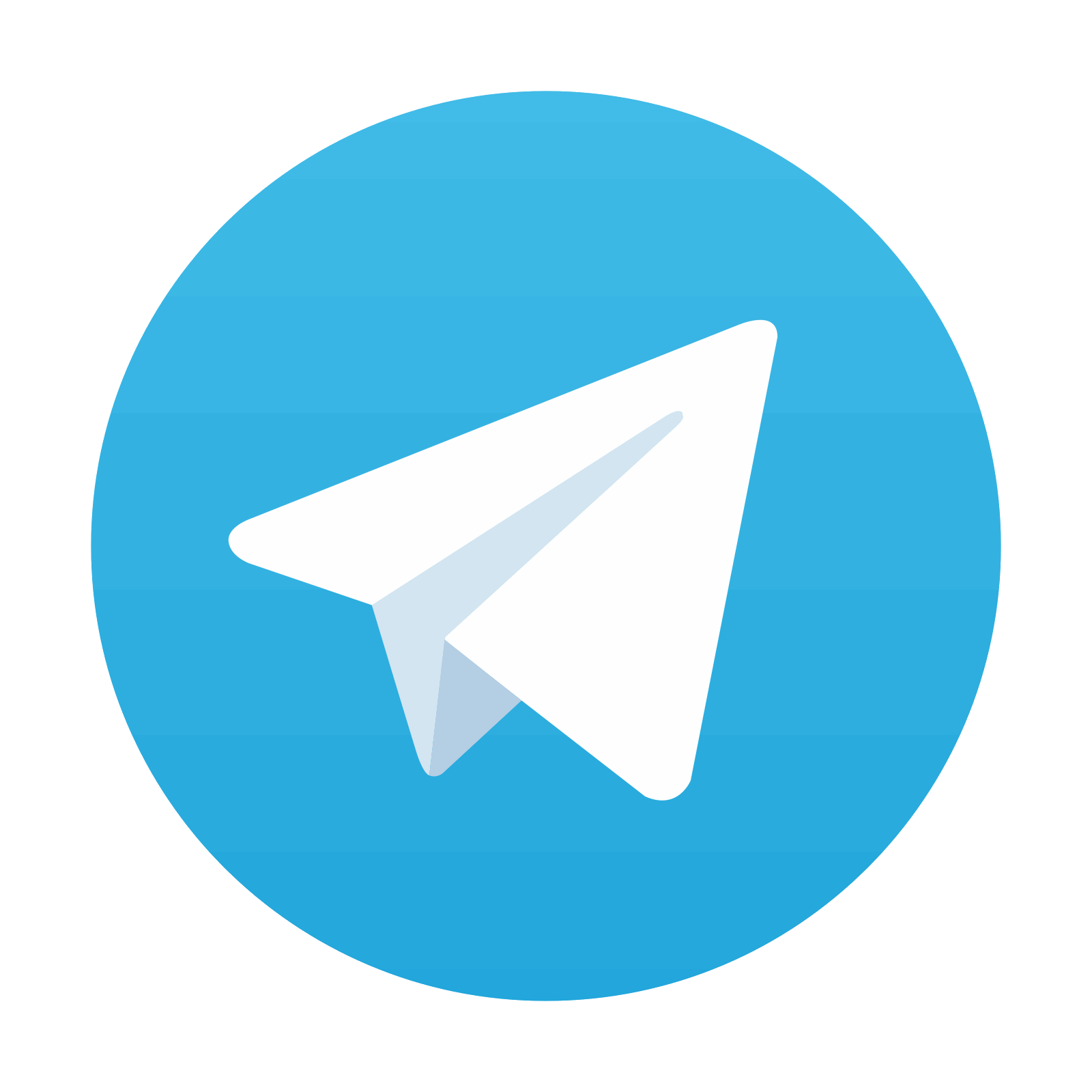
Stay updated, free articles. Join our Telegram channel

Full access? Get Clinical Tree
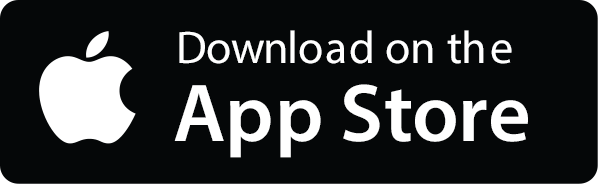
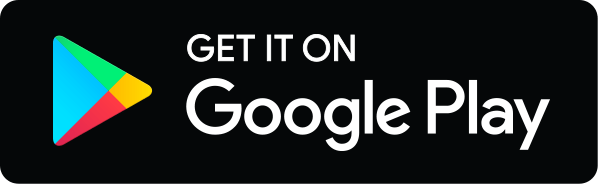
