Introduction
Pathologic fractures occur in diseased bone, and, in children, such fractures are caused by a spectrum of conditions different from those in adults. Children’s diseases frequently associated with pathologic fractures include noncancerous benign bone tumors and congenital or genetic abnormalities affecting the skeleton. Polyostotic disease with fractures affecting the immature skeleton is often caused by osteomyelitis, histiocytosis, vascular neoplasms, and metastasis (neuroblastoma and Wilms tumor). Rarely, sarcomas may initially manifest as fractures, and these patients require special consideration in their treatment approach. In contrast, causes of pathologic fractures in the adult skeleton, especially in individuals older than 40 years, include malignancies such as myeloma, metastatic carcinoma, lymphoma, and, rarely, sarcomas of the bone. Occasionally, giant cell tumors and enchondromas are identified. Elderly individuals frequently are seen with pathologic fractures from osteoporosis or Paget disease, in addition to metastasis ( Table 4-1 ).
TUMOR | ||
---|---|---|
AGE (yr) | BENIGN | MALIGNANT |
0–5 | Chondroma Unicameral bone cyst Osteoid osteoma Nonossifying fibroma Fibrous dysplasia | Neuroblastoma (metastatic) Rhabdomyosarcoma (metastatic) Ewing sarcoma Osteosarcoma Lymphoma |
10–40 | Chondroma Osteoid osteoma Aneurysmal bone cyst Unicameral bone cyst Nonossifying fibroma Fibrous dysplasia Eosinophilic granuloma Chondroblastoma (skeletally immature) Giant cell tumor (skeletally mature) | Osteosarcoma Ewing sarcoma Lymphoma |
40+ | Chondroma Giant cell tumor Hemangioma | Carcinoma Multiple myeloma Lymphoma Chondrosarcoma Osteosarcoma Chordoma |
The axial skeleton is frequently the site of metastatic foci because of its rich vascular supply relative to the appendicular skeleton. The site, patient’s age, and plain radiographic appearance of pathologic fractures make for a broad differential diagnosis. As a result, the orthopaedic oncologist must approach each case by taking into account the patient’s age, symptoms, image appearance, and an understanding of bone biology. Algorithms rarely suffice in pediatric orthopaedics or orthopaedic oncology.
The goal of this chapter is to introduce the reader to the multitude of variables involved in the successful treatment of pediatric pathologic fractures. A diagnosis must be made before embarking on any treatment strategy. Tissue documentation by biopsy is highly recommended to confirm the diagnosis of a pathologic fracture as the initial symptom. Depending on the confidence of the treating surgeon, a radiographic diagnosis may substitute for a tissue one, for example, in unicameral bone cysts (UBCs). After these variables are carefully weighed and balanced, an optimal treatment strategy can be formulated for a particular child. A different child with the same fracture may benefit from a different treatment. It can be said that pediatric orthopaedics and orthopaedic oncology are two orthopaedic surgical subspecialties that require a high degree of cognitive decision making. Treatment of children’s pathologic fractures is even more complex.
Bone Physiology
Bone is a specialized connective tissue with matrix consisting predominantly of type I collagen. It is a dynamic organ that receives one fifth of the cardiac output and is one of the only organs capable of true regeneration. Shaping of the skeleton and the buildup of bone mass during childhood and adolescence are a result of the constant interplay between bone formation and bone resorption. Bone remodeling continues throughout life. The average individual reaches peak bone mass in the third decade of life, and the adult skeleton contains approximately 2 million bone-remodeling units. Each unit comprises a spatial and temporal group of organized cells responsible for osteoclastic bone resorption and osteoblastic bone formation in response to local and environmental stimuli. Various pathologic states interfere with this remodeling process.
Bone resorption is mediated by the osteoclast, a multinucleated giant cell derived from granulocyte–macrophage precursors. Bone formation requires the presence and function of the osteoblast, which is derived from mesenchymal fibroblast-like cells. Net bone formation occurs by the process of coupling (contiguous and concurrent bone formation and resorption). Under normal circumstances, 88% to 95% of the bone surface area is quiescent while the remainder is involved in active remodeling. The total time required to complete a remodeling cycle for a typical bone-remodeling unit in a young adult is estimated to be 200 days. One bone-remodeling unit takes approximately 3 weeks to complete bone resorption, whereas it takes 3 months to form bone. Bone formation is quicker in children.
What is a pathologic fracture? Is it a radiologic, clinical, or combination diagnosis? Must the bone be completely or incompletely broken or displaced (or both) in one or more planes? Must the patient have symptoms or pain with activity? Can the bone be microscopically but not macroscopically fractured? These issues are pertinent because it is important to understand and develop proper treatment strategies on the basis of the answers to these questions. For the purposes of discussion, a pathologic fracture is defined as a clinically symptomatic interruption in the cortex of a diseased bone. Although fractures are typically macroscopic, they do not necessarily have to be so. A child’s bone is much more plastic than that of an adult. Bending without complete fiber separation occurs in a child and may be clinically relevant.
Bone strength is related to a combination of material and structural properties. The mineral component of bone is responsible for most of its compressive strength, whereas both mineral and protein components are important for strength in tension. Normal activity results in forces of compression, tension, and torsion. However, bone is weakest in torsion, and even a small cortical defect can significantly reduce torsional strength. For example, a 6-mm drill hole in the tibial shaft cortex, such as that generated to obtain a bone biopsy specimen, reduces torsional strength by 50%.
A tumor present at a fracture delays, alters, or prevents bone healing. In certain instances, the rapid growth of the tumor cells overwhelms the reparative process of bone. In metastatic bone disease, damage to the skeleton is usually much more extensive than can be expected simply from the amount of malignant cells present. Much evidence has now shown that most of the tumor-induced skeletal destruction is mediated by osteoclasts. Malignant cells secrete factors that both directly and indirectly stimulate osteoclastic activity. These factors include a variety of cytokines: interleukin-1 (IL-1), IL-6, tumor necrosis factor, IL-11, IL-13, and IL-17. IL-1 is the most powerful stimulator of bone resorption in vitro. Growth factors identified in tumorous bone include transforming growth factor α, transforming growth factor β, and epidermal growth factor. Paracrine factors that also stimulate osteoclastic activity include prostaglandin E and parathyroid hormone–related protein (PTHrP), and these factors are typically produced by malignant cells. PTHrP is immunologically distinct from parathyroid hormone, but the two hormones have significant homology at the amino terminal of the molecule, which is necessary for osteoclast stimulation. This peptide has recently been found to be important in the osteolysis induced by metastatic breast cancer and in the hypercalcemia of lung cancer.
Healing of pathologic fractures has been found to correlate most closely with tumor type and patient survival. Resection of the tumor deposit is an important part of the management of pathologic fractures. Thus, the biology of the bone and its biomechanics in conjunction with tumor pathology are important contributing variables to understand when the overall treatment of pathologic fractures is planned.
The most important task to be performed when a pathologic fracture is first detected is to establish the diagnosis with certainty. A radiographic diagnosis can be accurate, especially for UBCs; however, it is not a substitute for a tissue-confirmed diagnosis. Consequently, the authors recommend that a biopsy be strongly considered for all initial pathologic manifestations and other neoplasms of bone. Biopsy is a complex cognitive skill that depends on a careful physical examination, history, and interpretation of radiographic staging studies, including proper assessment of local, regional, and distant disease. It is crucial to determine whether polyostotic bone involvement is present. The surgeon is best able to interpret the diagnostic, anatomic, and pathologic significance of musculoskeletal disease and should thus review the images personally.
Biopsies are best performed by individuals with frequent experience. Complications from an improperly selected biopsy site can be devastating. Nondiagnostic or nonrepresentative harvesting of lesional tissue delays the diagnosis, and biopsy performed before complete imaging can hamper treatment planning. Care must be taken when choosing image-guided biopsy versus open biopsy as harm and delay can occur with a seemingly innocuous core/needle biopsy. Only after a tissue diagnosis is made can proper treatment ensue. Treatment must be based on an accurate diagnosis and understanding of bone biology (component 1) in conjunction with the pathology (component 2). Function is the third key element (component 3) considered when developing an overall treatment strategy.
Outcome Triangle
It is an error to treat a pathologic fracture as though it were a fracture through normal bone. Unfortunately, it is far too common for an orthopaedic surgeon to emphasize the type and choice of internal fixation implant rather than the timing and planning of surgery for a pathologic fracture. To achieve satisfactory treatment and outcomes in individuals with pathologic fractures requires that this dangerous reflex be harnessed. Proper treatment planning depends on weighing and taking into account all variables about a particular pathologic fracture and the individual patient before developing a treatment strategy. A multitude of treatment variables can be schematically represented in the three points of the outcome triangle ( Fig. 4-1 ). A thorough and properly weighted evaluation of the triad of variables in the outcome triangle is more likely to result in a favorable outcome for a patient with a pathologic fracture than will the more traditional orthopaedic surgical approach. The three variables are bone biology (component 1), including biomechanics; pathology (component 2); and function (component 3).

Outcome, Part I—Bone Biology
Bone biology, the first part of the triad, includes the cellular makeup of the fractured bone and its biomechanical environment. Each bone is different, and each site within a bone is different, depending on age. The healing potential of a bone is a function of certain variables, including remodeling potential, patient’s age, vascularity, position relative to the physis, and the density of bone-remodeling units. Of course, the pathologic process can affect these variables. Osteopetrosis, or marble bone disease, is a congenital disease manifested by deficient or absent osteoclasts. It frequently results in pediatric pathologic fractures ( Fig. 4-2 ). The lineage and the sequence of growth and differentiation factors necessary to convert primitive monocyte precursors to functioning osteoclasts have recently been elaborated in an elegant series of experiments. The first regulating factor converts the primitive monocyte–macrophage stem cell into a macrophage precursor. This transcription factor is labeled PU.1. Experimental knockout mice deficient in PU.1 lack macrophages and osteoclasts, and the condition is lethal. Mouse mutants deficient in macrophage colony-stimulating factor lack osteoclasts but contain immature macrophages; osteopetrosis develops in these mice but is cured by bone marrow transplantation.

Osteoprotegerin (OPG), a novel glycoprotein that regulates bone resorption, is a member of the tumor necrosis factor receptor superfamily. It is a decoy receptor that competes with RANK (receptor for activation of nuclear factor κB [NFκB]) for RANK ligand, which is responsible along with c- fos for differentiating the macrophage into an early osteoclast. OPG itself is a macrophage receptor similar to RANK, and it impedes osteoclastogenesis. Mice lacking αvβ3 integrin or c- src have substantial osteoclast numbers, but the cells fail to polarize. It allows conversion of an immature osteoclast into a functioning osteoclast with a ruffled border. Src knockout experiments yield nonfunctional osteoclasts lacking cathepsin K, carbonic anhydrase II, or the proton-transporting adenosine triphosphate synthase pump. These nonfunctional osteoclasts are incapable of creating an acidic microenvironment and thus cannot resorb bone. Therefore, overproduction of OPG results in limited osteoclast formation and the clinical syndrome of osteopetrosis. Decreased OPG production results in too many osteoclasts being produced and the clinical syndrome of osteoporosis. Administration of recombinant OPG to normal adult mice causes a profound yet nonlethal form of osteopetrosis. Thus, age and cellular makeup can help in determining the extent and healing potential of the bone and can serve as a guide for treatment.
Figure 4-2 depicts a subtrochanteric fracture in an osteopetrotic pediatric femur with no evidence of osteoclast function. Marrow formation is absent. A transverse fracture has occurred as a result of the pathologic process and brittleness of the bone. The fracture can heal but will not have significant remodeling potential. This outcome can be anticipated by the lack of bone resorption units, or osteoclasts. Understanding the disease process and the healing potential of the bone helps guide the treatment strategy. For instance, intramedullary implants are probably difficult or impossible to insert in such cases, and extramedullary implants create significant stress risers and subject the bone to additional delayed fractures. Therefore, biologic manipulation (bone marrow transplantation) of the fracture and secondary bone healing may offer the most advantageous treatment strategy.
Neurofibromatosis provides another example of how understanding bone biology and its relationship to disease affects the healing potential when a physician deals with a pathologic fracture. Union is compromised, and fracture healing is adversely affected because of the nonanatomic biomechanics and poor vascularity at the site. An anterolateral tibial bow is associated with neurofibromatosis type 1 approximately half the time. Pseudarthrosis is not usually present at birth and therefore not truly congenital, but it occurs during the first decade of life. The dysplastic, hamartomatous bone is poorly vascularized and unable to withstand the continual stresses applied, with resultant fracture and angulation ( Fig. 4-3 ). Manifestations of the disease alter with time, thereby limiting the usefulness of many of the morphologic classification systems currently in use. Union is best achieved by correcting the biomechanical and biologic environment because the pathology is of limited consequence in neurofibromatosis. Excision of the pseudarthrosis in conjunction with bone grafting and intramedullary fixation is a common procedure after failure of early treatment, including total contact bracing. Autogenous bone grafting is of assistance. A vascularized fibular graft or distraction osteogenesis can also be considered, depending on the circumstances. Realignment of the limb to create compressive rather than tension forces at the pseudarthrosis site is beneficial. A Syme amputation can result in spontaneous union.

Pathologic fractures of certain bones can help dictate their management. High stress is concentrated on the tension areas of weight-bearing bones, including the femoral neck and diaphysis of the tibia. Far more load is applied to weight-bearing bones than to non–weight-bearing bones. Fractures of vertebral bodies create the potential for spinal cord injuries. Vertebrae planae is caused by localized histiocytosis (eosinophilic granuloma) of the vertebral body. The natural history is one of spontaneous resolution without operative intervention, but a tissue diagnosis is often necessary, especially if monostotic disease is present, to exclude a malignant etiology. Infrequently, vertebrae planae can result in a neurologic deficit. Figure 4-4 portrays the natural history of vertebrae planae without surgical intervention.

The biology of pathologic fracture healing is affected by the administration of chemotherapy or by irradiation of the injured site, an issue especially important in malignant pathologic fractures. The administration of chemotherapy or radiation therapy to pathologic fractures secondary to sarcoma typically facilitates fracture healing. Although it is generally recognized that chemotherapy and radiation therapy delay the normal bone’s cellular response to healing a fracture, in the presence of rapidly dividing malignant cells, cytotoxic therapy provides a net positive result. Chemotherapy or external-beam radiation therapy, by destroying more cancerous than normal cells, creates a more favorable milieu for fracture healing. This effect of therapy on bone is another example demonstrating the need to understand bone biology for facilitation of fracture healing.
Outcome, Part II—Pathology
The pathology component of the outcome triangle is based on an understanding of the disease and its biologic behavior and natural history, factors that are paramount in determining treatment. Treatment also depends on knowing the diagnosis, which can only be established with certainty from a properly obtained and representative biopsy sample. Although myriad pathologic entities may affect bone, thus making it susceptible to fracture, the modes of treatment are far fewer. Groups of pathologic entities that can result in pathologic fracture include (1) genetic or metabolic bone disease abnormalities (e.g., osteogenesis imperfecta [OI] or osteopetrosis), (2) nutritional or environmental disturbances (e.g., rickets), (3) benign bone tumors (e.g., cystic, cartilaginous, and fibrous tumors), (4) skeletal sarcomas (e.g., osteosarcoma and Ewing sarcoma), and (5) skeletal metastases (e.g., neuroblastoma and rhabdomyosarcoma). These five disease categories represent a multitude of diagnoses, both neoplastic and nonneoplastic. Rather than elaborating on each pathologic entity, it is convenient to group them by pathobiologic activity ( Table 4-2 ).
DISEASE | INHERITANCE | GENETICS | DEFECT |
---|---|---|---|
Ewing sarcoma | — | t(11,22) | Loss of tumor suppressor gene(s) and creation of a fusion product |
Osteosarcoma | — | 17p13, p53 13q14 | Loss of tumor suppressor gene(s) Retinoblastoma gene type 1 |
Achondroplasia | AD | 4p16 | FGFR-3 gene Abnormal enchondral bone formation |
Neurofibromatosis (NF) NF1 NF2 | AD AD | 17q11 22q11 | Neurofibromin Schwannomin |
Osteogenesis imperfecta Clinical group I mild Clinical group II lethal Clinical group III deforming Clinical group IV moderate | AD AR AR AD | α1, #17 α2, #17 | 50% decreased type I collagen Unstable triple helix Abnormal type I Shortened pro-α chains |
Osteopetrosis Mild, tarda Malignant, infantile | SRC, OPG, RANK M-CSF; PU.1 | Osteoclasts lack complete differentiation Defective osteoclastogenesis | |
Rickets Vitamin D-deficient diet Vitamin D-dependent Vitamin D-resistant | AR X-linked dominant | 12q14 | Decreased vitamin D intake Leads to secondary hyperparathyroidism Lack renal 25 (OH)-vitamin D 1 α-hydroxylase Impaired renal tubular phosphate resorption ( PEX, cellular endopeptidase) |
Fibrous dysplasia | — | 20q13.2–13.3 | G s α (receptor-coupled signaling protein for cAMP) |
Osteochondromatosis | — | 8q24.1/11p11 | EXT1, EXT2 genes |
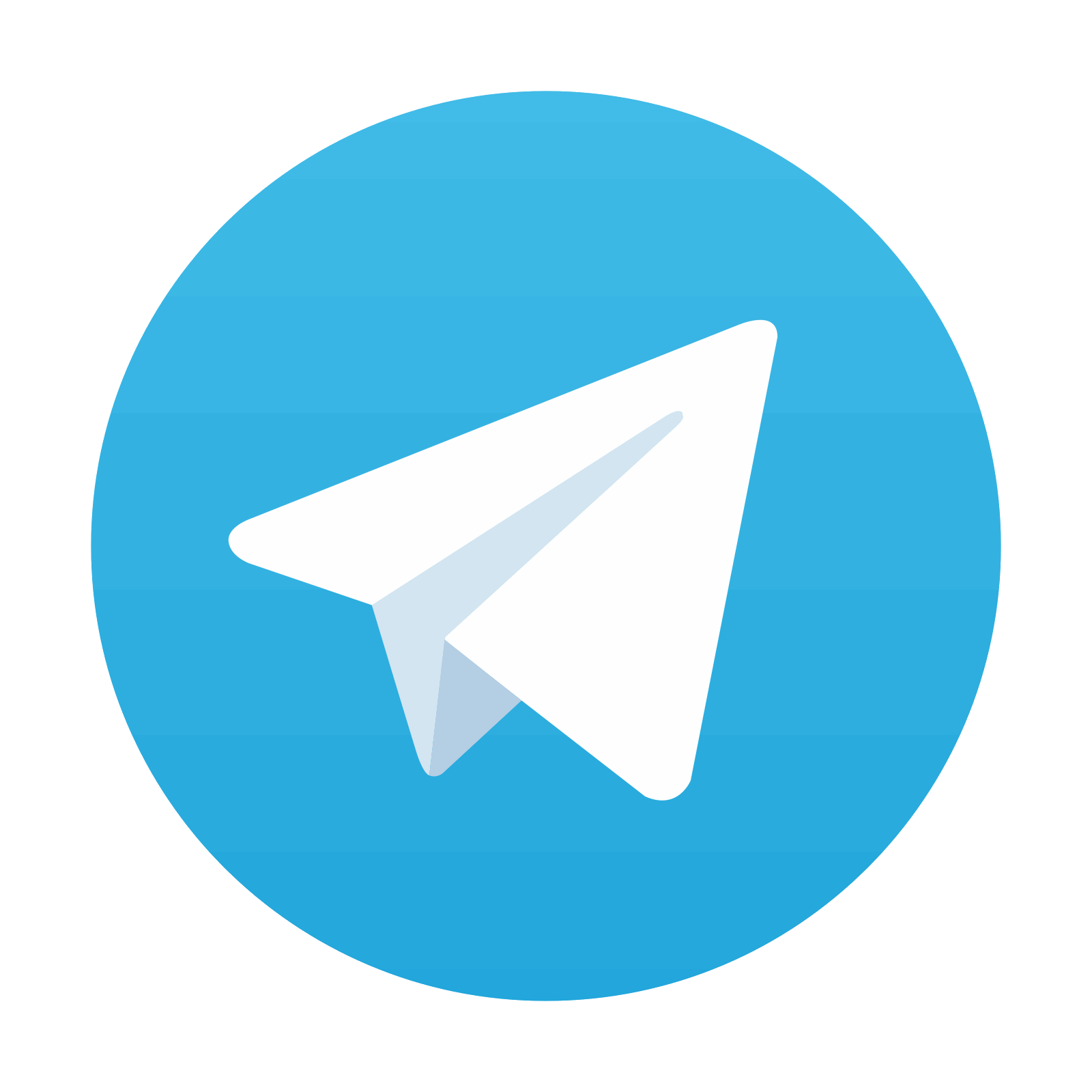
Stay updated, free articles. Join our Telegram channel

Full access? Get Clinical Tree
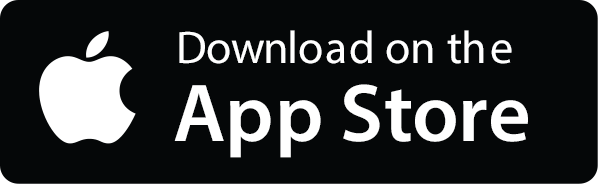
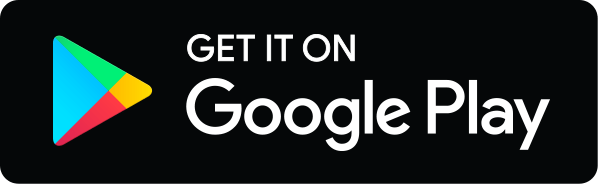
