Metabolic bone disease is becoming a more recognized disease entity. Endocrine disease often is associated with metabolic bone disease. This article attempts to delineate the current knowledge of osteoporosis and osteomalacia in endocrine diseases. As the population ages, the amount of metabolic bone disease and number of fractures will increase. It is imperative that health care providers screen and treat patients at risk of metabolic bone disease. Much research is ongoing in this field and the number of treatment options will greatly expand. Focusing on ways to maximize the development of the fetal skeleton to improve peak bone mass, such as improving maternal vitamin D levels during pregnancy, may best address the treatment of osteoporosis and osteomalacia for the entire population.
Metabolic bone disease is becoming a more recognized disease entity. Endocrine disease often is associated with metabolic bone disease. This article attempts to delineate the current knowledge of osteoporosis and osteomalacia in endocrine diseases. The World Health Organization (WHO) defines osteopenia as when the dual energy x-ray absorptiometry (DEXA) T-score in a postmenopausal women is between −1.0 and −2.4, and osteoporosis as when the T-score is −2.5 or lower. The bone is normal, but there is an increased risk of fracture because of fragility of the bone. Osteomalacia refers to bone that is abnormal because of abnormal mineralization. The most common cause of osteomalacia is vitamin D deficiency.
Hyperthyroidism
Thyroid hormones have a direct resorptive effect on bone. Low thyroid-stimulating hormone (TSH) and high thyroxine (T 4 ) levels can exert a negative effect on bone resorption. Hyperthyroid patients have a significant negative calcium balance. Bone loss occurs more in cortical than trabecular bone. Hyperthyroid states can be compartmentalized into overt hyperthyroidism, endogenous subclinical hyperthyroidism, and exogenous subclinical hyperthyroidism due to suppressive therapy and thyroid hormone replacement therapy. Overt hyperthyroidism and iatrogenic hyperthyroidism result in significant osteoporosis and fractures. A recent study demonstrated that bone mineral density and level of bone-turnover markers were significantly more favorable in 115 subjects with TSH level between 0.35 and 6.3mU/L than in women with a TSH level less than 0.3 mU/L. It has been estimated that more than 10% of postmenopausal women in the United States receive thyroid hormone replacement and up to 20% of these women are over-replaced, inducing subclincal hyperthyroidism.
Overt hyperthyroidism requires rapid correction of the thyrotoxicosis to prevent skeletal and other manifestations of this syndrome. Data do suggest that treatment of endogenous subclinical hyperthyroidism can minimize further loss of bone density. Treatments with estrogen and bisphosphonates seem to prevent thyroid hormone-mediated bone loss.
Hyperparathyroidism
Hyperparathyroidism is divided into primary and secondary hyperparathyroidism( Table 1 ). Classically, primary hyperparathyroidism is recognized as a disease of “bones, stones, and groans”; now, the condition is asymptomatic in most patients because the current biochemical profile contains serum calcium, and markedly high levels of calcium are detected sooner than in the past. Primary hyperparathyroidism is a generalized disorder of calcium, phosphate, and bone metabolism that results from an increased level of parathyroid hormone (PTH). The prevalence is as high as 1 in 500 to 1 in 1000. Most adults with primary hyperparathyroidism (80%–85%) have a single benign adenoma, whereas 15% to 20% have hyperplasia of all glands. Four-gland hyperplasia is common in patients with chronic kidney disease (CKD) or with multiple endocrine neoplasia Type I or II. Patients with primary hyperthyroidism are at risk of bone loss and osteoporosis. Cortical bone is more affected than trabecular bone; as such, forearm bone mineral density (BMD) is most affected in primary hyperparathyroidism. BMD measurement is essential in all patients with primary hyperparathyroidism. It helps define the extent of disease and helps to monitor ongoing disease activity. A 10-year prospective study of patients with primary hyperparathyroidism who underwent parathyroidectomy demonstrated normalization of biochemical values and increased bone density. The 2002 National Institute of Health guidelines on asymptomatic primary hyperparathyroidism recommend that patients with primary hyperparathyroidism and the following criteria be managed surgically: a calcium level greater than 1.0 mg/dL over the upper limit of normal, a 24 -hour urinary calcium excretion level greater than 400 mg, a reduction in creatinine clearance of 30%, a T-score of −2.5 at any site, or age less than 50 years. If these criteria do not apply, patients can be managed nonsurgically. Nonsurgical patients should test serum calcium level every 6 months and BMD of the lumbar spine, hip, and forearm yearly. Patients can maintain a normal amount of calcium and vitamin D. The 2009 International Task Force on the medical management of asymptomatic primary hyperparathyroidism commented that bisphosphonates and hormone replacement therapy reduce bone loss in the population. Calcimimetics are agents that alter the function of the extracellular calcium-sensing receptor and offer an exciting new approach in patients with primary hyperparathyroidism. In these patients, calcimimetics may reduce serum calcium and PTH levels but have no beneficial effect on bone turnover; further study is needed, in this area. Frequently, patients who undergo removal of a parathyroid adenoma require only observation, because BMD often improves rapidly.
Calcium | PTH Level | |
---|---|---|
Primary | ↑ | ↑ |
Secondary | ↓ | ↑ |
Secondary | normal | ↑ |
Hypercalcemia Unrelated to PTH | ↑ | ↓ |
Secondary hyperparathyroidism is common and is due to inadequate calcium and vitamin D intake. It is essential to evaluate patients with osteopenia or osteoporosis for secondary osteoporosis and to correct calcium or vitamin D deficiency. This helps attain maximal response to additional pharmacologic therapy prescribed. It is best to correct significant secondary hyperparathyroidism before initiating antiresorptive or anabolic therapy ( Table 2 ).
Replacement of calcium | |
Calcium citrate 500 mg 3–4 times per day | |
Replacement of vitamin D | |
Vitamin D (ng/ml) | |
<20 | Ergocalciferol (Drisdol) 50,000 IU/wk + vitamin D 3 1000–2000 iu/d |
20–30 | Vitamin D 3 2000–5000 IU/d |
>30 | Vitamin D 3 1000–2000 IU/d |
Hyperparathyroidism
Hyperparathyroidism is divided into primary and secondary hyperparathyroidism( Table 1 ). Classically, primary hyperparathyroidism is recognized as a disease of “bones, stones, and groans”; now, the condition is asymptomatic in most patients because the current biochemical profile contains serum calcium, and markedly high levels of calcium are detected sooner than in the past. Primary hyperparathyroidism is a generalized disorder of calcium, phosphate, and bone metabolism that results from an increased level of parathyroid hormone (PTH). The prevalence is as high as 1 in 500 to 1 in 1000. Most adults with primary hyperparathyroidism (80%–85%) have a single benign adenoma, whereas 15% to 20% have hyperplasia of all glands. Four-gland hyperplasia is common in patients with chronic kidney disease (CKD) or with multiple endocrine neoplasia Type I or II. Patients with primary hyperthyroidism are at risk of bone loss and osteoporosis. Cortical bone is more affected than trabecular bone; as such, forearm bone mineral density (BMD) is most affected in primary hyperparathyroidism. BMD measurement is essential in all patients with primary hyperparathyroidism. It helps define the extent of disease and helps to monitor ongoing disease activity. A 10-year prospective study of patients with primary hyperparathyroidism who underwent parathyroidectomy demonstrated normalization of biochemical values and increased bone density. The 2002 National Institute of Health guidelines on asymptomatic primary hyperparathyroidism recommend that patients with primary hyperparathyroidism and the following criteria be managed surgically: a calcium level greater than 1.0 mg/dL over the upper limit of normal, a 24 -hour urinary calcium excretion level greater than 400 mg, a reduction in creatinine clearance of 30%, a T-score of −2.5 at any site, or age less than 50 years. If these criteria do not apply, patients can be managed nonsurgically. Nonsurgical patients should test serum calcium level every 6 months and BMD of the lumbar spine, hip, and forearm yearly. Patients can maintain a normal amount of calcium and vitamin D. The 2009 International Task Force on the medical management of asymptomatic primary hyperparathyroidism commented that bisphosphonates and hormone replacement therapy reduce bone loss in the population. Calcimimetics are agents that alter the function of the extracellular calcium-sensing receptor and offer an exciting new approach in patients with primary hyperparathyroidism. In these patients, calcimimetics may reduce serum calcium and PTH levels but have no beneficial effect on bone turnover; further study is needed, in this area. Frequently, patients who undergo removal of a parathyroid adenoma require only observation, because BMD often improves rapidly.
Calcium | PTH Level | |
---|---|---|
Primary | ↑ | ↑ |
Secondary | ↓ | ↑ |
Secondary | normal | ↑ |
Hypercalcemia Unrelated to PTH | ↑ | ↓ |
Secondary hyperparathyroidism is common and is due to inadequate calcium and vitamin D intake. It is essential to evaluate patients with osteopenia or osteoporosis for secondary osteoporosis and to correct calcium or vitamin D deficiency. This helps attain maximal response to additional pharmacologic therapy prescribed. It is best to correct significant secondary hyperparathyroidism before initiating antiresorptive or anabolic therapy ( Table 2 ).
Replacement of calcium | |
Calcium citrate 500 mg 3–4 times per day | |
Replacement of vitamin D | |
Vitamin D (ng/ml) | |
<20 | Ergocalciferol (Drisdol) 50,000 IU/wk + vitamin D 3 1000–2000 iu/d |
20–30 | Vitamin D 3 2000–5000 IU/d |
>30 | Vitamin D 3 1000–2000 IU/d |
Hypoparathyroidism
Hypothyroidism is an uncommon disease caused by insufficient PTH. The most common causes are immunologic destruction, radiation therapy, and surgical removal. Patients develop hypocalcemia and hyperphosphatemia. Idiopathic hypophosphatemia can lead to various musculoskeletal findings, including diffuse ligamentous and entheseal ossifications. A recent report describes a 50-year-old man with idiopathic hypoparathyroidism who was diagnosed with diffuse idiopathic skeletal hyperostosis when 40 years old.
The treatment usually consists of replacement of calcium and calcitriol and being sure to avoid hypercalciuria, which increases the risk of nephrolithiasis. Phosphate binders, such as calcium acetate (PhosLo), sevelamer (Renagel), and lanthanum (Fosrenol), can be used for resistant hyperphosphatemia. Several studies have looked at the use of intact PTH as a treatment for this condition. Patients seem to require less calcium and calcitriol when given PTH. Patients treated with PTH also seem to have correction of the low bone-turnover state associated with hypothyroidism.
Vitamin D deficiency
Vitamin D deficiency has received much attention over the past few years with the recognition of the many people deficient in Vitamin D living in northern latitudes. Vitamin D is obtained from cutaneous production when 7-dehydrocholesterol is converted to vitamin D 3 (cholecalciferol) by ultraviolet B radiation or by oral intake of vitamin D 2 (ergocalciferol) or vitamin D 3 . Fatty fish is the most important dietary source. Fortification of foods is practiced in some countries, including the United States; however, the standardization of this process is poor. After production in the skin, vitamin D is hydroxylated in the liver to 25-hydroxyvitamin D [25(OH)D], which is further hydroxylated in the kidney to 1, 25(OH)D, the active metabolite. Vitamin D is usually assessed by measuring the serum concentration of 25(OH)D. There is increasing agreement that the optimal circulating level of 25(OH)D should be approximately 30 to 32 ng/mL or greater. Ideally, the optimal vitamin D level maximally suppresses PTH; this would ensure adequate intake of vitamin D. It is thought that the recommendation for daily vitamin D would eventually be to keep the serum vitamin D level well above the current recommendation of 32 ng/mL.
Epidemiology
Elderly patients have been found to have decreased dermal synthesis. Older nursing home residents frequently do not go outdoors often and are not exposed to sunshine on a regular basis. In a meta-analysis of randomized controlled trials, a dosage of 700 to 800 IU/d of vitamin D was found to reduce the relative risk of hip fracture by 26% and any new vertebral fractures by23% versus calcium or placebo. There have been smaller studies that failed to show the benefit of vitamin D in fracture reduction, but a Cochrane review did show benefit in fracture reduction when both calcium and vitamin D supplements were given. It has been shown that low vitamin D levels are associated with muscle weakness and an increased risk of falls.
25(OH)D is the major form of circulating vitamin D, but 1, 25(OD)D (calcitriol) is the active metabolite. This metabolite binds to the nuclear vitamin D receptor (VDR). By binding to the appropriate receptor, vitamin D can stimulate intestinal calcium absorption, stimulate bone resorption, and decrease PTH secretion. Vitamin D seems to be able to enhance receptor-activated nuclear factor-κB ligand (RANKL) and osteoprotegerin (OPG) and stimulate osteoclast formation and genes involved in bone formation, such as osteocalcin and osteopontin Vitamin D deficiency results in poor mineralization of the growth plate, which becomes thick, wide, and irregular. In adults who are vitamin D-deficient, the newly formed bone matrix, the osteoid, does not mineralize. Vitamin D receptors exist on many cells other than bone cells and active investigation is under way to investigate other functions of vitamin D.
Clinical Aspects of Vitamin D Deficiency
Rickets presents with decreased longitudinal growth, widening of the epiphyseal zones, and painful swelling around involved joints, with bowing of the tibia caused by poor mineralization and swelling of rib cartilage. Children evidence developmental delay. Osteomalacia can be associated with bone pain, muscle weakness, and difficulty walking. Proximal muscle is affected more than distal strength, so activities such as climbing stairs and getting out of a low chair can be particularly difficult.
Rickets and osteomalacia are often seen when vitamin D levels get to 10 ng/mL or lower. Coexistent laboratory findings often include hypocalcemia, hypophosphatemia, and elevated alkaline phosphate level. PTH levels are usually elevated, suggesting a component of secondary hyperparathyroidism.
Radiographs in patients with rickets reveal decreased mineralization around the epiphysis, unsharp bone margins, and less contrast. These findings rapidly improve with treatment. In osteomalacia, radiographs show less contrast and are less sharp. Looser zones represent pseudofractures. These areas can also be seen on bone scan. DEXA results can improve rapidly with treatment.
Treatment of Vitamin D Deficiency
Rickets and osteomalacia are fairly quickly treated with vitamin D supplementation unless there is a component of malabsorption, as in patients with celiac disease or inflammatory bowel disease or who have undergone gastric bypass. Vitamin D 3 can be supplemented at dosages of 1000 to 2000 IU/d. Alternatively, vitamin D 2 (ergocalciferol) can be given at dosages of 50,000 IU/wk. A calcium supplement should always be added. After treatment of vitamin D deficiency, serum calcium and phosphorus levels should correct quickly. PTH levels may lag behind and take some time to normalize, correcting a state of secondary hyperparathyroidism. Children may require lower doses of vitamin supplementation. Vitamin D levels should be followed to ensure repletion.
Vitamin D intoxication is rare. Signs and symptoms may include those of hypercalcemia, such as thirst, polyuria, and headache. Laboratory testing may reveal hypercalcemia and normal or increased phosphate, increased serum creatinine or blood urea nitrogen, very high 25(OH)D, normal 1, 25(OH)D, and suppressed PTH levels. Patients respond to withdrawal of supplemental calcium and vitamin D. Patients with granulomatous diseases or lymphoproliferative disorders have macrophages containing 1α-hydroxalase, thus permitting conversion of 25(OH)D to 1, 25(OH)D. Supplementing these patients with vitamin D should be handled carefully, because vitamin D intoxication can occur much more easily. Glucocorticoids can decrease the hydroxylation of 25(OH)D to 1, 25(OH)D and help reverse this process in this select group of patients.
Hypophosphatemia
The 3 most common causes of hypophosphatemia are redistribution of phosphorus from extracellular fluid into cells, increased urinary excretion, and decreased intestinal absorption. The clinical manifestations depend on the severity of the condition. Early on, hypophosphatemia can cause increased bone resorption. Impaired bone mineralization causes rickets in children and osteomalacia in adults. X-linked hypophosphatemic rickets is the most common disorder of renal wasting, occurring in about 1 of 25,000 live births. A recent report describes lateral subtrochanteric pseudofractures occurring in adults with osteomalacia from hypophosphatasia and X-linked hypophosphatemia, suggesting that a low bone-turnover state played a role in the fractures. A mutation in the type 2a sodium-phosphate cotransporter has been associated hypophosphatemia, nephrolithiasis, and osteoporosis ; this mutation results in abnormal renal phosphate reabsorption.
Hypomagnesemia
Hypomagnesemia is usually caused by loss of magnesium from the gastrointestinal tract or kidney. Hypocalcemia is a common manifestation of moderate-to-severe magnesium depletion. Both can be associated with neuromuscular hyperexcitability and tetany, as elicited by positive Chvostek and Trousseau signs. Epidemiologic studies suggest that a low magnesium diet may be a risk factor of osteoporosis. Magnesium deficiency in rats can cause a decrease in bone mass and skeletal fragility. Magnesium supplements may be associated with increased bone mass. The physician must screen those at risk of hypomagnesemia with a serum magnesium level.
Gonadal steroids
Estrogen and testosterone play an important role in maintaining skeletal architecture. The estrogen and testosterone decrease with aging has profound effects on the skeleton. At menopause, women undergo rapid trabecular bone loss. The duration of rapid bone loss can begin 1 to 2 years before menopause and continue for up to 5 years after, when there can be a loss of 20% to 30% of trabecular bone and 5% to 10% of cortical bone. About 8 to 10 years after menopause, there is a slower and continuous rate of bone loss in which cortical and trabecular bone loss is similar. Men demonstrate slow, equal loss of cortical and trabecular bone from midlife until death. As men reach a higher peak bone density and do not have the accelerated rate of loss that women have at menopause, bone generally does not fracture in men as frequently as in women.
Changes in bone quantity and structure help account for the varying rates of fractures in women and men with aging. Distal forearm fractures increase markedly in women around the time of menopause and plateau about 15 years after menopause. Vertebral and hip fracture rates increase after menopause; then vertebral fracture rate plateaus but hip fracture rate continues to increase. The incidence of vertebral and hip fractures in men parallel that of women but are delayed by one decade, due to the lack of a manpower. Men do not have a significant increase in wrist fractures with aging.
In women, at menopause, there is an increase in the basic multicellular unit activation frequency, an extension of the resorption period, and a shortening of the formation period. Estrogen has a central role in osteoblastic differentiation and limits apoptosis of osteoblasts and osteocytes. Estrogen supplementation can have an anabolic effect on bone. Estrogen suppresses production of RANKL and increases production of OPG, which ultimately decreases osteoclastic production and differentiation. Estrogen can also promote apoptosis of osteoclasts.
In men, with aging, there is an increase in sex hormone binding globulin (SHBG) resulting in less bioavailable testosterone and estrogen. Although testosterone is the major sex steroid in men, many studies demonstrate that male BMD at many sites correlates better with bioavailable estradiol levels than testosterone levels. Clinically, it is still useful to check testosterone levels in men with osteoporosis or osteomalacia and consider replacement if levels are low.
During pregnancy, there is increased intestinal absorption of calcium, in part, due to increased 1,25(OH)D levels. Although this has not been studied extensively, there is probably no significant change in BMD during pregnancy. During lactation, because of increased parathyroid hormone-related protein (PTHrP) levels, there is increased bone resorption to provide the calcium needed for breast milk (280–400 mg/d). There is also reduced renal excretion of calcium during lactation. Calcitonin may play a role in limiting bone resorption during lactation. The temporary skeletal loss of calcium during lactation is completely restored after weaning. Overall, pregnancy and lactation seem not to have a significant detrimental effect on the maternal skeleton. Women who are vitamin D-deficient during pregnancy also seem to give birth to children with normal skeletons because of increased maternal calcium absorption.
Medications that lower estrogen levels, such as the aromatase inhibitors, are associated with bone loss and fractures. Depo-Provera suppresses menstruation and is associated with bone loss and fracture. The newer, very low-dose estrogen oral contraceptives, which tend to suppress menstruation, may also be associated with bone loss and fracture. Men treated with androgen deprivation therapy, such as prostate cancer treatment with Lupron, are also at risk of bone loss and fracture.
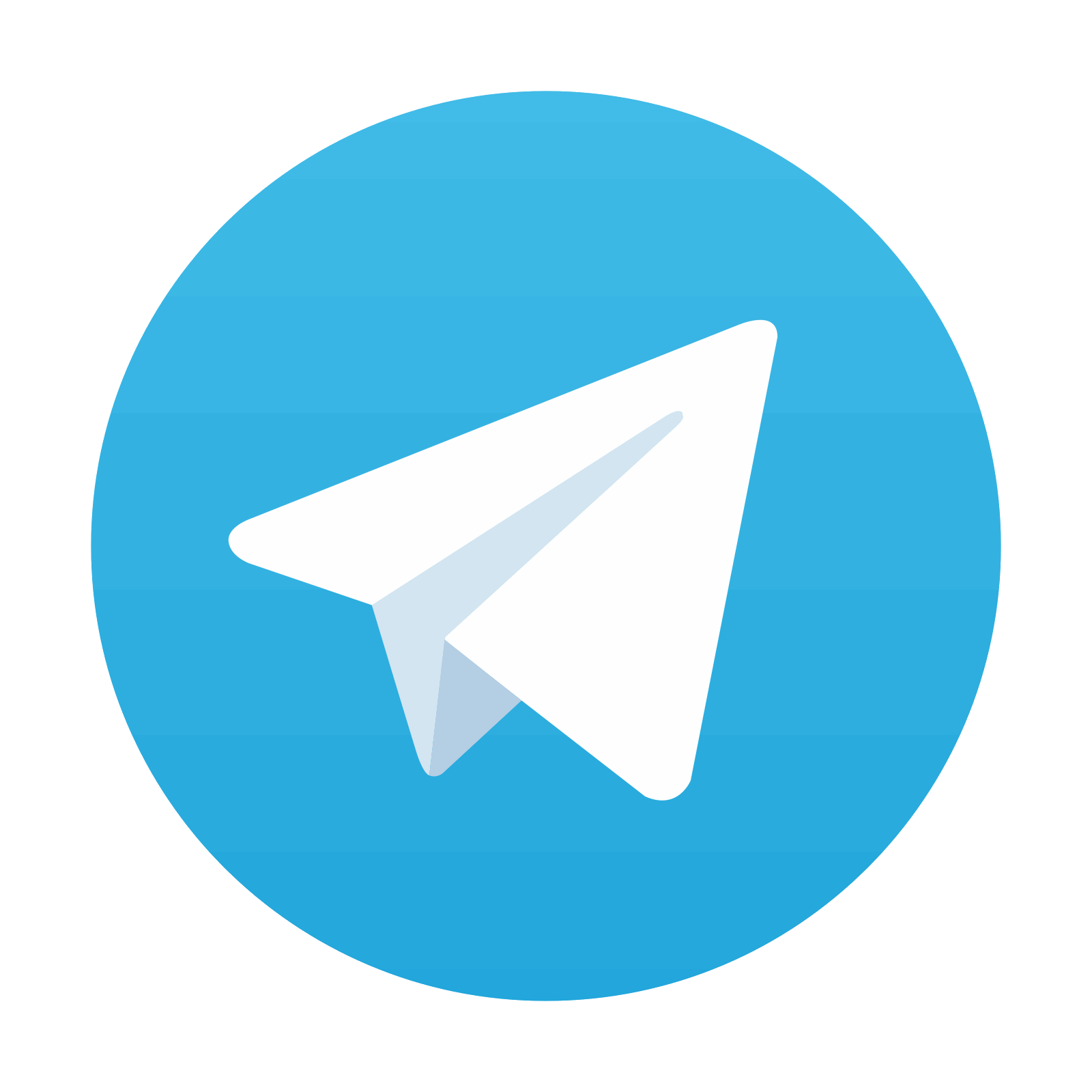
Stay updated, free articles. Join our Telegram channel

Full access? Get Clinical Tree
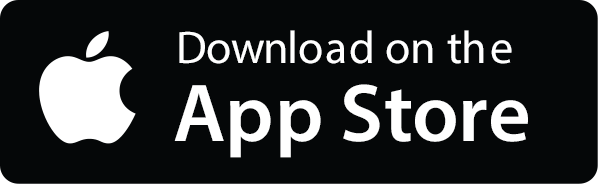
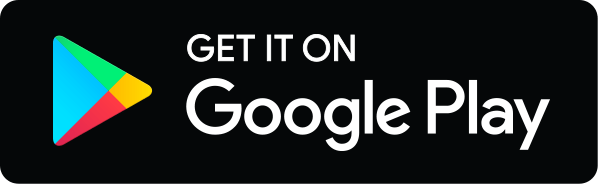