osteomyelitis is common in patients who are immunocompromised, those with indwelling catheters, previous spinal instrumentation or spine trauma, and systemic bacteremia from another site. Similar to septic arthritis, Staphylococcus aureus is the most common organism in both extremity and vertebral osteomyelitis and represents up to half of all cases with a high incidence of methicillin resistance in certain populations (Table 1).
Table 1 Summary of the Epidemiology of Major Categories of Deep Musculoskeletal Infection | |||||||||||||||
---|---|---|---|---|---|---|---|---|---|---|---|---|---|---|---|
|
isolated from DFI with Pseudomonas aeruginosa typically being the most common (Table 1).
Table 2 Summary of Selected Cell Wall-Anchored and Secreted Proteins Involved in Staphylococcus aureus Pathogenesis | |||||||||||||||||||||||||||||||||
---|---|---|---|---|---|---|---|---|---|---|---|---|---|---|---|---|---|---|---|---|---|---|---|---|---|---|---|---|---|---|---|---|---|
|
aureus coagulase cleavage of fibrinogen.11 A peripheral ring of necrotic neutrophils then forms around the nidus, which are unable to effectively remove the S aureus and contribute to soft-tissue injury.11 Staphylococcal virulence proteins such as nuclease and adenosine synthase A convert NETs into products that induce macrophage cytotoxicity, which may be a significant mechanism in protecting bacteria within the SAC.12 Eventually, these SACs can rupture, releasing the bacterium into the surrounding tissue.10
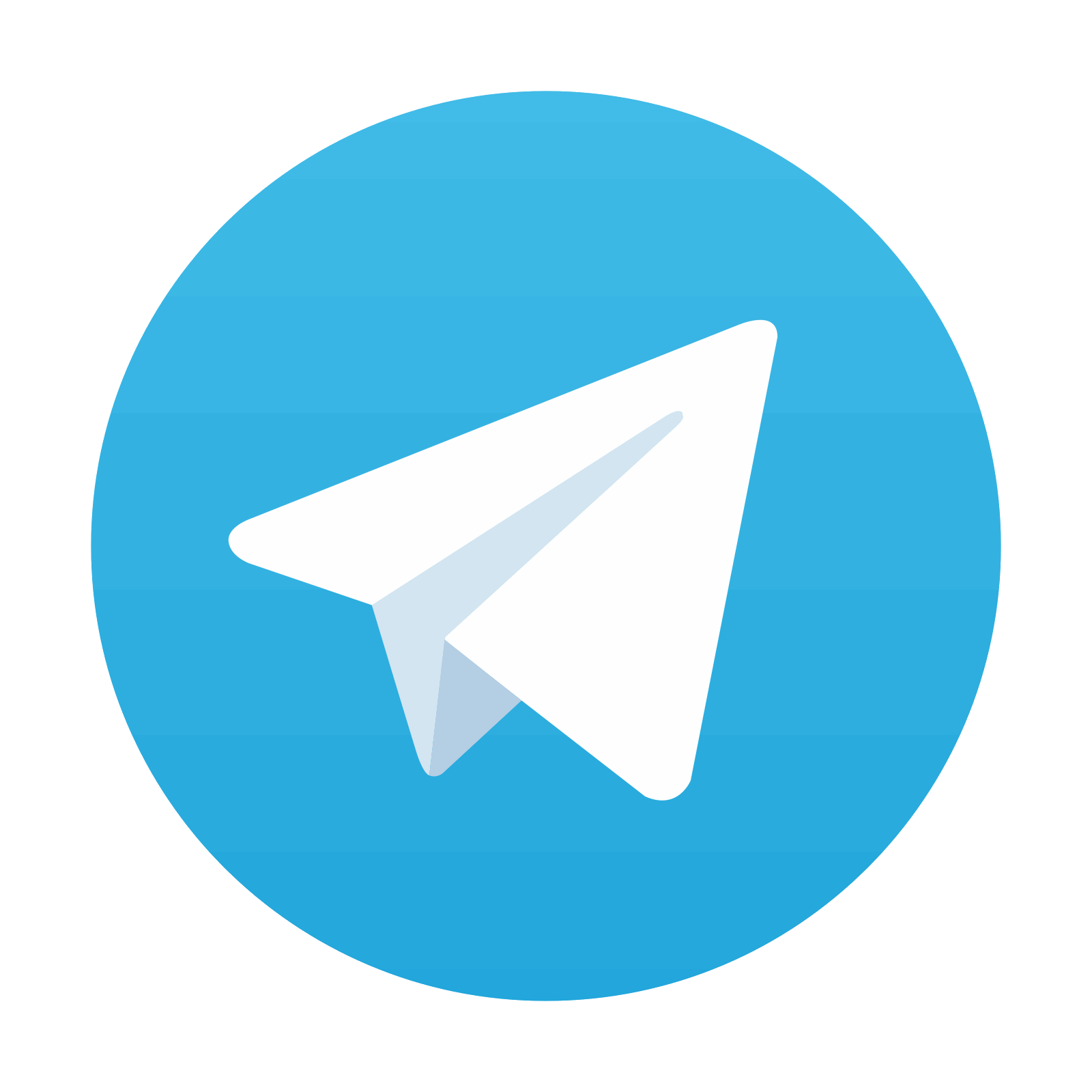
Stay updated, free articles. Join our Telegram channel

Full access? Get Clinical Tree
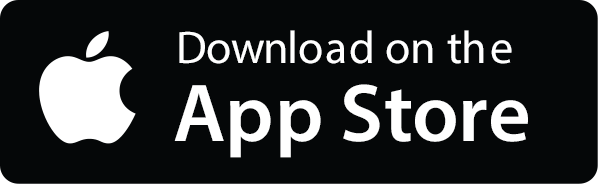
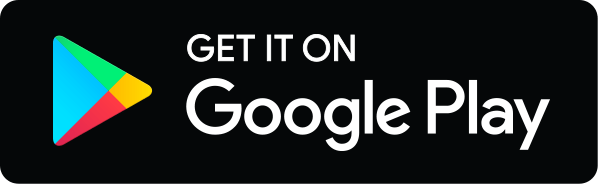
