Noninvasive Biochemical Markers in Osteoarthritis
Patrick Garnero
Introduction
Osteoarthritis (OA) is a highly prevalent age-related disease characterized by an abnormal and degraded cartilage, associated with synovitis and variable subchondral bone reaction, resulting in pain and severe mobility impairment. Synovitis can be evaluated using systemic inflammation. Parameters of inflammation such as highly sensitive assay for C-reactive protein (CRP) or cytokine levels. However, because these biological markers are not specific of alteration of joint tissues, they are poorly correlated with cartilage damage at the individual level. Thus, the most established method for assessing the extent of joint destruction in OA remains plain radiography. This technique provides direct information on bone, but only indirect estimation of cartilage loss via the measurement of the distance between opposing articular cortices (described as joint-space width, JSW). Radiography is rather insensitive and does not allow an early detection of joint tissue damage nor an efficient monitoring of the efficacy of treatment aimed at preventing joint destruction. Magnetic resonance imaging (MRI) is more sensitive than radiography and provides direct, accurate, and precise evaluation of the key joint structures including articular cartilage, osteophytes, bone marrow, synovium, ligaments, and menisci, although most of these studies focused on cartilage assessment.1 Complementary to these imaging modalities, there has recently been a considerable interest in identifying specific biological markers which could reflect quantitative and dynamic variations in joint tissue remodeling. In this chapter we will review the recent development in biochemical markers for bone, cartilage, and synovium tissue turnover and then review their potential clinical role for the management of OA.
Assays for Biochemical Markers of Joint Tissue Turnover
Joints are enclosed in a strong fibrous capsule. The inner surfaces of the joint capsule are lined with a metabolically active tissue, the synovium, which secretes the synovial fluid that provides the nutrients required by the tissues within the joint. Each articular bone end within the joint is lined by a thin layer of hydrated soft tissue, i.e., the articular cartilage. In joint diseases, there is a loss of the normal balance between the synthesis and the degradation of the macromolecules that provide articular cartilage with its biomechanical and functional properties. Concomitantly, changes occur in the metabolism of the synovium and in the microarchiture and turnover of the subchondral bone. Consequently, for a comprehensive assessment of the physiopathological processes that lead to joint failure in OA, it is important to gain access to highly specific biochemical markers of cartilage, bone, and synovium tissue turnover (Table 12-1).
Biochemical Markers of Cartilage Turnover
Articular cartilage is a multiphasic material with two major phases: a fluid phase composed of water and electrolytes, and a solid phase composed of collagen, proteoglycans, glycoproteins, other proteins, and the chondrocytes. Each of
the phases contributes to its mechanical and physiological properties. Of the organic components, the collagens—mostly collagen type II—provide the quantitatively major component, followed by proteoglycans, especially aggrecan2 (Fig. 12-1). Although the other proteins are not major components in terms of the absolute solid phase, they may approach the molar concentration of collagen and aggrecan. The maintenance of cartilage integrity is dependent on a tight balance between the synthesis and degradation activities of chondrocytes, which is altered in OA.
the phases contributes to its mechanical and physiological properties. Of the organic components, the collagens—mostly collagen type II—provide the quantitatively major component, followed by proteoglycans, especially aggrecan2 (Fig. 12-1). Although the other proteins are not major components in terms of the absolute solid phase, they may approach the molar concentration of collagen and aggrecan. The maintenance of cartilage integrity is dependent on a tight balance between the synthesis and degradation activities of chondrocytes, which is altered in OA.
TABLE 12-1 BIOCHEMICAL MARKERS OF BONE, CARTILAGE, AND SYNOVIUM TURNOVER | ||||||||||||||||||||||||||||||||||||
---|---|---|---|---|---|---|---|---|---|---|---|---|---|---|---|---|---|---|---|---|---|---|---|---|---|---|---|---|---|---|---|---|---|---|---|---|
|
Markers of Type II Collagen Turnover
The predominant type of collagen is type II, which is cartilage specific and forms the basic fibrillar structure of the extracellular matrix. Types IX and XI are also cartilage specific and are present together with type II collagen. There is evidence that many collagens, including types II, IX, and XI in cartilage, exist as hybrid molecules. In contrast, type VI collagen forms distinct microfibrils that appear concentrated in the capsular matrix surrounding individual chondrocytes or groups of chondrocytes.
Markers of Type II Collagen Synthesis. Type II collagen is synthesized and secreted by the chondrocytes as a precursor, the procollagen. Type II procollagen is constituted by the type II collagen molecule—comprising the major triple helix ([α1 (II)]3) and the linear N- and C-telopeptides—and the N and C-terminal propeptides at the two extremities. The propeptides are removed by specific proteinases before the mature molecules are incorporated into fibrils in matrix following which they are released into biological fluids, and their levels are believed to reflect type II collagen synthesis. Type II procollagen is synthesized in two splice forms, type IIA and type IIB. Type IIA contains an additional 207 base pair exon (exon 2) encoding the 69 amino acid cysteine-rich domain of the N-propeptide; it is expressed mainly by fetal tissues but can be re-expressed in osteoarthritic cartilage,3 whereas the IIB variant is the major form of adult cartilage. The two forms of aminoterminal propeptide of type III procollagen (PIINP) (PIIANP and PIIBNP) and procollagen type II carboxy-terminal propeptide (PIICP) may thus serve as markers of type II collagen synthesis. An enzyme-Linked immunosorbent assay (ELISA) for PIIANP was recently developed using a specific polyclonal
antibody raised against recombinant exon-2 protein. Compared to healthy sex- and age-matched controls, increased serum levels of PIIANP were reported in early knee OA,4 whereas decreased values5 were found in patients with advanced disease. These results obtained from analysis of systemic levels of PIIANP are in agreement with direct measurements of type II collagen in human OA cartilage using proline incorporation which show that type II collagen synthesis is increased in early stages of cartilage degeneration, but progressively decreased in late stages.6 These data indicate that in early OA, chondrocytes upregulate their biosynthetic activities to compensate for increased damage, but this anabolic response may become deficient in advanced OA. Because type IIB collagen is the major form of adult cartilage, the development of an assay for PIIBNP would be very useful as it may provide different information compared to PIIANP in OA.
antibody raised against recombinant exon-2 protein. Compared to healthy sex- and age-matched controls, increased serum levels of PIIANP were reported in early knee OA,4 whereas decreased values5 were found in patients with advanced disease. These results obtained from analysis of systemic levels of PIIANP are in agreement with direct measurements of type II collagen in human OA cartilage using proline incorporation which show that type II collagen synthesis is increased in early stages of cartilage degeneration, but progressively decreased in late stages.6 These data indicate that in early OA, chondrocytes upregulate their biosynthetic activities to compensate for increased damage, but this anabolic response may become deficient in advanced OA. Because type IIB collagen is the major form of adult cartilage, the development of an assay for PIIBNP would be very useful as it may provide different information compared to PIIANP in OA.
Markers of Type II Collagen Degradation. Type II collagen is degraded by proteolytic enzymes secreted by the chondrocytes and the synoviocytes of the synovium tissue, including the matrix metalloproteinases (MMP) and the cysteine proteases.7,8 Among the MMPs, the collagenases cleave the triple helical region of type II collagen at a single site between residues 778 and 776 generating two fragments representing three fourths and one fourth, respectively, of the intact collagen molecule. Other MMPs, including the gelatinases and stromelysins, especially stromelysin 1 (also named MMP-3), can cleave denaturated collagen within the triple helical domain and the telopeptides. Stomelysin 1, which attacks type II collagen within the telopeptides, may not have a major role in this process9 but could contribute indirectly to collagen breakdown by activating the other MMPs. MMP-13, whose expression is increased in OA, could be one of the major enzymes involved in the increased type II collagen degradation.7,10 Although MMPs are likely to play a major role in degradation of type II collagen in OA, several cysteine proteases have also been suggested to contribute to cartilage destruction. Among them, numerous reports have shown increased expression of cathepsins B, L, K, and S.8 Cathepsins B and L have been shown to cleave type II collagen within the nonhelical telopeptide of collagens, whereas cathepsin K is capable of cleaving collagen at multiple sites within the triple helix of type I and type II collagen11,12 and has recently been suggested to be the major cysteine protease expressed in OA cartilage.13,14 Cathepsin S is unique in that it is the only cysteine protease to be active at neutral and slightly alkaline pH and thus can participate in extracellular matrix degradation of articular
cartilage. Although cathepsin S has a weak collagenolytic activity, it is very efficient in hydrolyzing aggrecan15 and thus may play a deleterious role in the integrity of the aggrecan-type II collagen network.
cartilage. Although cathepsin S has a weak collagenolytic activity, it is very efficient in hydrolyzing aggrecan15 and thus may play a deleterious role in the integrity of the aggrecan-type II collagen network.
The development of assays specific for type II collagen breakdown represents a breakthrough in the field of biological markers for OA, given that degradation of collagen fibers is associated with irreversible cartilage destruction. Antibodies recognizing different type II collagen fragments have been developed (Fig. 12-2). Those directed against the neoepitopes generated by the collagenases include the so-called COL2-3/4 long mono or C2C which is specific of type II collagen and the COL2-3/4C Short or C1,2C which detects cleavages of both type II and type I collagen10 and the type II collagen neoepitope (TIINE). These collagenase neoepitopes have mainly been used to demonstrate increased type II collagen cleavage in OA cartilage explants, although more recently they have been applied in synovial fluid and serum immunoassays both in animal models of OA16 and in patients with knee OA.17 More recently, other type II collagen markers have been identified including fragments of the triple helical domain (Helix-II, Coll 2-1)18,19 and a fragment of the C-telopeptide (CTX-II)20 (Fig. 12-2). Helix-II, Coll 2-1, and CTX-II have been shown to be increased in patients with knee and hip OA and rheumatoid arthritis (RA). The different type II collagen degradation fragments are likely to be released from articular cartilage by different biological pathways. Indeed, in vitro studies showed that Helix-II and CTX-II are released from human cartilage by different cathepsins and MMPs, and immunohistochemistry experiments also indicate that Helix-II and CTX-II have distinct distributions in tissue sections of human articular OA cartilage.21,22 Thus, their combination may better characterize the complex mechanisms of cartilage damage in arthritis than the use of one of these two biochemical markers alone. This also provides a biological basis for the independent and additive information given by Helix-II and CTX-II on disease progression in patients with hip OA23 and in early RA.18
Cartilage matrix molecules including type II collagen can undergo post-translational modifications which can either be mediated by an enzymatic process or be spontaneous and age related. Measuring post-translational-modified cartilage matrix proteins may lead to the development of biochemical markers which can give valuable information on altered biological processes related to OA. Chondrocytes can express high levels of inducible and neuronal forms of nitric oxide synthetase which generate nitric oxide. Nitric oxide can then react with superoxide radical to form peroxynitite, a potent oxidizing radical that can in turn react with tyrosine residues of proteins to form nitrotyrosine. Two different assays recognizing a sequence—which can be either un-nitrosylated (Coll 2-1) or nitrosylated (Coll 2-1 NO2) of the triple helix of type II collagen—have recently been developed.19 Increased serum levels of Coll 2-1 and Coll 2-1 NO2 have been reported in patients with knee OA. One-year changes of their urinary levels—but not baseline values—were modestly related to more rapid disease
progression of knee OA over 3 years.24 It remains unclear, however, from these studies whether there is an additive value of investigating the nitrosylated form of type II collagen fragments in OA.
progression of knee OA over 3 years.24 It remains unclear, however, from these studies whether there is an additive value of investigating the nitrosylated form of type II collagen fragments in OA.
Markers of Aggrecan Turnover
Aggrecans are proteoglycans composed of a protein (core protein) and glycosaminoglycan (GAGs) chains that are covalently attached to the core protein (Fig. 12-1). The core protein of aggrecan has a molecular mass of approximately 230 kDa and consists of three globular domains, G1, G2, and G3, and two GAGs attachment domains, the keratan sulfate (KS) and the chondroitin sulfate (CS) domains. The total molecular mass can reach approximately 2.200 kDa.25 The G1 domain has a structure consisting of three disulfide-bonded loops and interacts with hyaluronan in the formation of proteoaglycan aggregates. The interglobular domain (IGD) between the G1 and G2 domains is 90 amino-acid residues long, has a rod-shape, and contains proteolytic cleavage sites susceptible to a variety of proteinases including MMPs, aggrecanases including members of the A Disintegrin And Metalloproteinase with ThromboSpondin motifs family (ADAMTS) such as ADAMTS-5,26 serine proteases such as plasmin and leukocyte elastase, and cysteine proteases such as cathepsin B and K.7,8 After synthesis of the core protein by the chondrocytes, up to 50 KS chains [Gal β(14) GlucNAc β(1-3)] and 100 CS chains [GlcA β(1-3) GalNAc β](1-4)] are added during post-transcriptional processing, and together these carbohydrates make up more than 90% of the molecular mass. The G3 domain located at the C-terminus consists of three modules: epidermal growth factor (EGF)-like module, C-type lectin module, and complement regulatory proteins module. With aging, the population of aggrecan without the G3 domain increases in cartilage because of its proteolytic cleavage.
Putative markers of aggrecan synthesis include epitopes located on the CS chains of the aggrecan such as the 3-B-3, 7-D-4, and 846 epitope. The 3-B-3 (-) antibody recognizes atypical structures at the nonreducing terminal of the CS glycosaminonoglycan side chains of the proteoglycans. The 7-D-4 antibody is directed against another atypical structure (sulfation pattern) in native CS GAGs of proteoglycans.27,28 Epitopes 3-B-3 (-) and 84629 are present in high concentration in fetal cartilage and almost absent in mature normal cartilage.30,31,32 In contrast, epitope 7-D-4 is frequently found in normal adult cartilage.
The development of a specific biochemical marker of aggrecan degradation would be very interesting as aggrecan is more easily degraded than type II collagen and may thus be a sensitive indicator of early cartilage damage. However, increased levels of aggrecan fragments in synovial fluid and serum should be interpreted with caution. Indeed, although newly synthesized aggrecan molecules are particularly susceptible to degradation, the concentration of aggrecan fragments can actually increase as a result of an upregulation of aggrecan synthesis. Keeping this limitation in mind, it has been shown that the majority of aggrecan fragments found in the joint fluid from injured or OA joints are large, but have lost the G1 domain.33 These degradation fragments can be measured by immunoassays using antibodies against KS or the core protein. The ELISA using the antibody 5D4 (also called AgKS) remains the most used assay for quantifying smaller aggrecan-related molecules in serum.34 Although several research groups have recently developed assays for the various neoepitopes in the protein portion of aggrecan, it is still unclear which of these epitopes is the most relevant to be measured in biological fluids, and clinical data are still limited.
Nonaggrecan and Noncollagen Proteins
Cartilage Oligomeric Matrix Protein (COMP). Among noncollagenous proteins, the most investigated cartilage marker is the protein so-called COMP. COMP is a 524 kDa homopentameric extracellular matrix glycoprotein (5 identical units of 755 amino acid), which belongs to the thrombospondin family. Each monomer is composed of an amino-terminal cysteine-rich domain, four epithelial growth factor (EGF)-like domains, eight calmodulin-like repeats, and a C-terminal globular domain.35,36,37 The biological function of COMP is still unclear. Bovine and human proteins—but not rat protein—contain an Arg-Gly-Asp (RGD) sequence, suggesting that COMP may mediate cell binding through integrin. The carboxy-terminal globular domain binds to collagen I, II, and IX, suggesting that COMP may be involved in regulating fibril formation and maintaining integrity of collagen network. The fact that COMP may have important functions is also illustrated by the data showing that two human dominant skeletal dysplasias, pseudoachondroplasia and multiple epiphyseal dysplasia,38,39,40 are associated with a mutation in the potentially Ca-binding domain of COMP gene. However, COMP-deficient mice do not have cartilage abnormalities,41 suggesting that these human diseases are probably not caused by a reduced amount of COMP but by other mechanisms such as folding defects or extracellular assembly alterations due to potentially dysfunctional mutated COMP.
Originally felt to be cartilage specific, over the last years COMP has been identified in all structures of the joints including ligaments, meniscus, tendons, and synovium.42 COMP was also found to be secreted by osteoblasts and vascular smooth muscle. In cartilage, synovial fluid, and serum of patients with OA, COMP has been shown to be present as the intact molecule and several fragments.43 These fragments are likely to result from the activity of MMPs such as MMP-1, MMP-13, MMP-9, and ADAMTS4,44 although it remains unknown which of these enzymes play the major role of COMP degradation in vivo. A careful epitope mapping is required and monoclonal antibodies specific for intact molecules and fragments would be very useful, especially for assessing the efficacy of MMP inhibitors in preventing cartilage destruction in patients with OA. Currently, however, available immunoassays based on polyclonal45 or monoclonal antibodies46 appear to detect both the intact molecule and fragments in body fluids.
Chitinase 3-like Protein 1 (YKL-40) and Chitinase 3-like Protein 2 (YKL-39). Chitinase 3-like protein 1 (YKL-40, also named human cartilage glycoprotein 39) and Chitinase 3-like protein 2 (YKL-39) are two different mammalian glycoproteins related in sequence to family 18 of bacterial and fungal Chitinases.47 YKL-40 does not exhibit glycosidase activity against Chitinase substrates, as the glutamate in the active site Trp-Glu-Tyr-Pro is replaced by another amino acid. It was originally described as a major gene product of chondrocytes and synovial cells.48 Subsequently however, mRNA for YKL-40 has also been detected in high amounts in the liver, which may be the main source of circulating YKL-40-, weakly in brain, kidney, and placenta, and undetectable in heart, lung, skeletal muscle, mononuclear cells, and skin fibroblasts.48 49 The function of YKL-40 is unknown. One hypothesis is that increased expression of this protein by human articular chondrocytes and/or synovial cells in patients with OA/RA could increase the degradative capacity of these cells, although no proteolytic activity has been yet demonstrated including against hyaluronan.48 Although serum YKL-40 has been reported to be increased in patients with knee OA or hip OA in some,50,51 but not in all52 studies, its clinical utility in OA remains unclear as it does not correlate with radiological damage52 and progression.53 YKL-40 may be a more interesting marker of disease progression in patients with RA54 or cancer.55,56,57
It has been shown that YKL-39, but not YKL-40, was overexpressed in cartilage from patients with OA compared to healthy cartilage.58,59 Autoantibodies to YKL-39 have also been reported in a proportion of patients with OA similar to patient with RA, suggesting that humoral response to this molecule may be involved in the pathophysiology of these arthritic diseases.60,61 All together these preliminary data suggest that YKL-39 may be more specific for cartilage that YKL-40 and may prove to be a more sensitive biochemical marker of joint damage in OA, although data for synovial fluid and serum YKL-39 are still lacking.
Biochemical Markers of Bone Turnover
Bone turnover is characterized by two opposite activities, the formation of new bone by osteoblasts and the resorption of old bone by osteoclasts. The rate of formation or degradation of bone matrix can be assessed either by measuring an enzymatic activity of the bone-forming or bone-resorbing cells—such as alkaline and tartrate resistant acid phosphatase (TRACP)—or by measuring bone matrix components released into the circulation during formation or resorption (Table 12-1). Current bone turnover markers cannot discriminate between turnover changes in a specific skeletal envelope, i.e., trabecular versus cortical, or compartment, i.e., skeletal versus subchondral, but mainly reflect whole body net changes. These markers are usually separated into markers of formation and resorption, but it should be kept in mind that in disease states or following certain treatment where both events are coupled and change in the same direction, any marker will reflect the overall rate of bone turnover. Increasingly, specific biochemical markers for bone remodelling have been identified in recent years and used mainly in osteoporosis (reviewed in Garnero and Delmas62).
At present, the most sensitive markers for bone formation are serum total osteocalcin—an hydroxyapatite-binding noncollagenous protein exclusively synthesized by osteoblasts, odontoblasts, and hypertrophic chondrocytes—bone alkaline phosphatase, and the procollagen type I N-terminal propeptide (PINP). Procollagen propetides are cleaved by specific propeptidases and are partly released into the circulation from where they are cleared by liver endothelial cells. Most of the circulating propeptide pool originates from bone formation.
The majority of bone resorption markers are degradation products of collagen type I, which is the most abundant protein of bone tissue, except for TRACP isoenzyme 5b which reflects mainly the number of osteoclasts, some specific fragments of osteocalcin, and bone sialoprotein (BSP). During bone resorption, osteoclasts secrete different factors such as acid, matrix MMPs, and cathepsin K. These enzymes degrade type I collagen into several products including the hydroxypyridinium crosslinks of collagen pyridinoline (PYD) and deoxypyridinoline (DPD), the MMP product carboxyterminal telopeptide of type I collagen (ICTP), and the combined MMP and cathepsin K products type I cross-linked N- and C-telopeptide (NTX and CTX).62 Partly degraded type I collagen is taken up by the osteoclast into vesicles. To these vesicles the enzyme TRACP 5b is added intracellularly which can further degrade the type I collagen breakdown products. The content of this vesicle is excreted from the cell at the apical side.63 Immunological assays are now available for PYD and DPD in urine, for ICTP in serum, and for CTX, NTX both in serum or urine. Most of these biochemical marker assays are now available on automatic platforms with increased precision over manual assays and high throughput which allow convenient accurate measurements in large number of individuals.
The different type I collagen-related markers can respond differently in the presence of diseases and treatments, although there is limited data in OA. For example, serum and urine CTX and NTX levels are markedly increased in postmenopausal women with osteoporosis and their values decrease markedly and rapidly with antiresorptive therapy, contrasting with the slight and nonsignificant modifications of ICTP in these two conditions.64 In contrast, serum ICTP is a sensitive marker in other pathological conditions including malignant bone diseases and RA.65,66 These different responses are likely to result form differences in the enzymatic pathways leading to the release of CTX/NTX and ICTP from bone type I collagen (Fig. 12-3). Indeed, it has been shown that the peptide ICTP recognized by the antibody used in the immunoassay can be cleaved by cathepsin K, an osteoclastic-specific cysteine protease which is the key enzyme responsible for bone collagen degradation in normal physiological conditions. Thus, when cathepsin K is active, ICTP will be destroyed and not anymore recognized by the immunoassay resulting in low serum levels. Conversely, the peptide ICTP can be released from type I collagen by some MMPs including MMP-2.67,68 Because of the important role played by MMPs in pathological bone degradation including bone
metastases and arthritis, ICTP may be a sensitive marker in these conditions, although no data have yet been published in OA. In contrast, the CTX and NTX peptides are directly generated by the action of cathepsin K on collagen and their immunoreactivity can be further increased by subsequent degradation by MMPs.68 The understanding of these enzymatic pathways is of crucial importance for the clinical interpretation of data under treatments especially with anti proteases.
metastases and arthritis, ICTP may be a sensitive marker in these conditions, although no data have yet been published in OA. In contrast, the CTX and NTX peptides are directly generated by the action of cathepsin K on collagen and their immunoreactivity can be further increased by subsequent degradation by MMPs.68 The understanding of these enzymatic pathways is of crucial importance for the clinical interpretation of data under treatments especially with anti proteases.
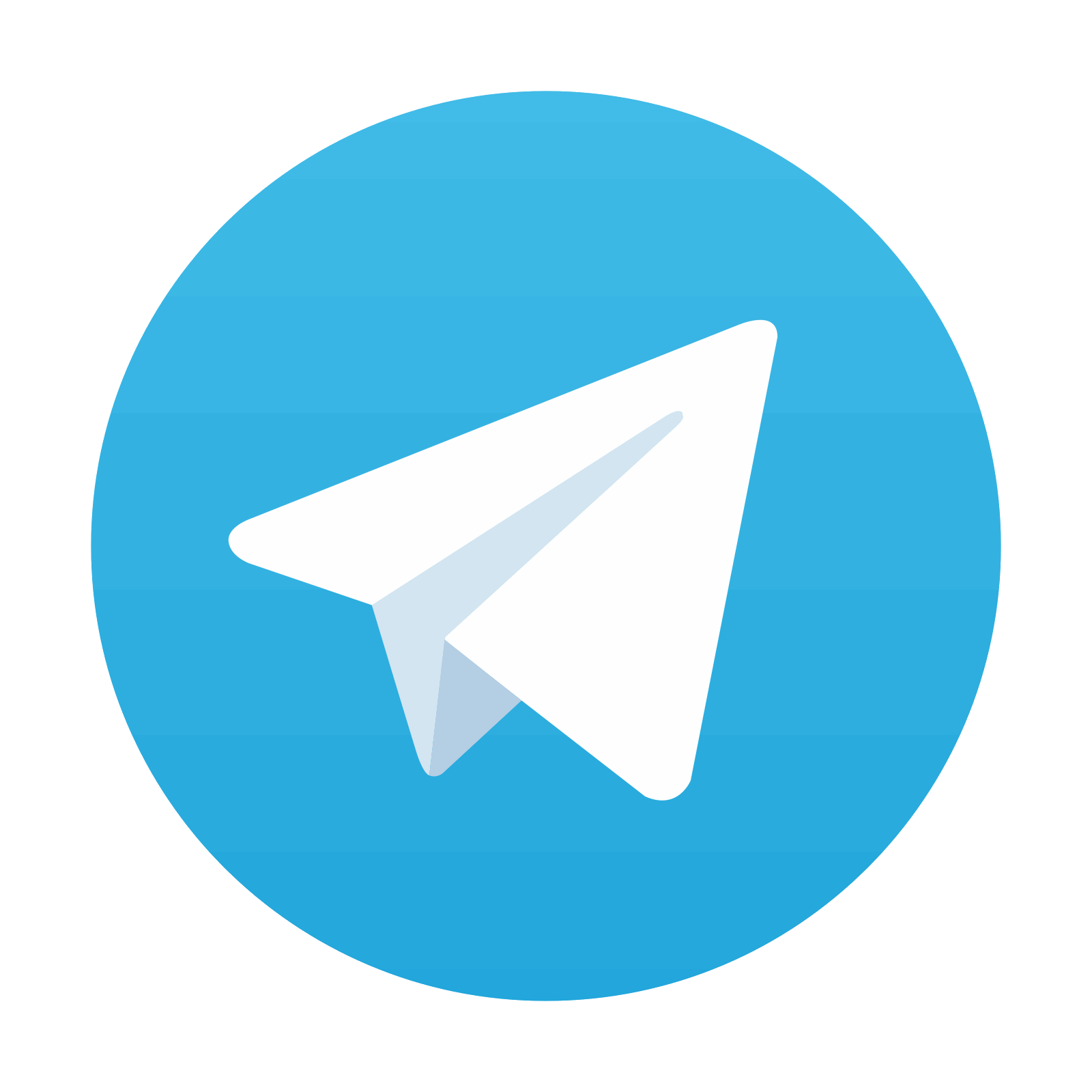
Stay updated, free articles. Join our Telegram channel

Full access? Get Clinical Tree
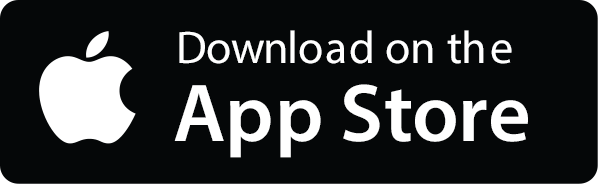
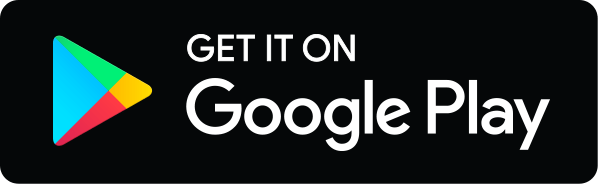
