Abstract
Numerous studies have recently been published on improving upper-limb motor function after stroke. There has been a particular interest in brain stimulation techniques, which could promote brain plasticity. In this review, transcranial Direct Current Stimulation (tDCS) and repetitive Transcranial Magnetic Stimulation (rTMS) are presented as techniques that could be relevant in Physical Medicine and Rehabilitation (PM&R) centers in the future. We are presenting a comprehensive literature review on the studies using tDCS or rTMS for upper-limb rehabilitation after a stroke. Both techniques have shown their ability to modify cortical excitability and to transitorily improve upper-limb function after one single stimulation session. The first placebo-controlled, blinded therapeutic trials, which included repeated daily sessions, seem quite promising, and deserve to be validated by further trials.
Résumé
L’amélioration fonctionnelle du membre supérieur de l’hémiplégique suite à un accident vasculaire cérébral (AVC) est l’objet de nombreux travaux et reste un enjeu majeur de la rééducation, avec des résultats encore décevants. Parmi les techniques récemment proposées, émergent les techniques de stimulation cérébrale, dont l’objectif serait d’orienter la plasticité cérébrale. Dans cet article, deux techniques de stimulation cérébrale non invasives, la stimulation transcrânienne par courant continu ( transcranial Direct Current Stimulation [tDCS]) et la stimulation magnétique transcrânienne répétitive ( repetitive Transcranial Magnetic Stimulation [rTMS]), sont présentées ; elles pourraient, à terme, être utilisées de manière courante dans cette déficience. Une revue exhaustive de la littérature proposant d’utiliser tDCS et rTMS chez des patients présentant une atteinte motrice du membre supérieur consécutive à un AVC est réalisée. D’un point de vue fondamental, la capacité de ces deux techniques à moduler l’excitabilité du cortex cérébral et à améliorer, au moins de manière transitoire, la fonction du membre supérieur, semble validée pour une session unique de stimulation. Les résultats des premiers essais thérapeutiques, en aveugle et contre placebo, proposant des sessions répétées et quotidiennes, semblent prometteurs, et méritent d’être confirmés par de nouveaux essais.
1
English version
1.1
Introduction
Optimizing the care management of stroke patients is a national priority. Stroke prevalence is estimated at 400,000 persons in France. It is the third leading cause of death in men and the second in women. Furthermore, it is the first cause of acquired disability. More than 50% of stroke patients retain severe neurological impairments, most often affecting motor function . Among these patients, about 80% will retain some grasping deficits linked to upper limb impairments.
Improving upper limb function has become a major challenge for PM&R teams; however, the results remain disappointing . In daily practice, the principles of upper limb rehabilitation are largely based on the PM&R techniques that emerged in the middle of the 20th century . However, major conceptual developments were recently developed , emerging from fundamental neuroscience that evidenced brain plasticity with brain imaging techniques. The concepts of learning and of task-oriented rehabilitation have become essential . The concepts of learned non-use (initially described by Meige ) and of interhemispheric competition are nowadays more readily admitted . Newly proposed rehabilitation strategies therefore focus on restoring the balance between the two hemispheres in favor of the affected one.
Invasive or non-invasive brain stimulations have the capacity to modulate cortical excitability and to optimize brain plasticity. These techniques have already largely been studied in psychiatry and pain management . In post-stroke rehabilitation, interesting results have been reported for improving phasic disorders , neglect syndrome and swallowing disorders . However, the use of these treatments still remains to be defined in clinical practice. Transcranial Direct Current Stimulation (tDCS), repetitive Transcranial Magnetic Stimulation (rTMS) and Paired-Associative Stimulation (PAS) are non-invasive techniques. Invasive stimulations (implanted epidural electrodes) have been tested in phase I, II and III clinical trials, with quite inconclusive results .
The objective of this article is to describe tDCS and rTMS: these two techniques may be used in neurological rehabilitation in the future. We will also present a literature review on their application for upper limb rehabilitation after stroke.
1.2
Brain stimulation techniques
1.2.1
Transcranial Direct Current Stimulation
1.2.1.1
Overview
The therapeutic use of electrical currents through the scalp has been proposed since the ancient times (writings describing the use of torpedo fish for relieving headaches in authors such as Scribonius Largus, Claudius Galenus, and Pliny the Elder). In the 11th century, the Arab physician Ibn Sidah used this technique to treat epileptic seizures . After the works of Walsh on torpedo fish , the beginnings of electrophysiology were reported by the Italian physicists Galvani and Volta who described the physiological effects induced by electrical currents . Some years later, Giovanni Aldini proposed to use galvanic currents for treating mental disorders .
With the recent development of Transcranial Magnetic Stimulation (TMS), which enables to measure the cortical excitability, Priori et al. could determine the current parameters (intensity, electrode position, stimulation duration) that induce an actual modulation of the primary motor cortex excitability. This has been validated and further developed by Nitsche and Paulus who drew the bases of today’s stimulation paradigms .
1.2.1.2
Presentation
tDCS consists in applying low-intensity current between two electrodes on the scalp. The “active” electrode is placed above the part of the brain to be stimulated and the reference electrode is placed on a neutral position. Part of the current will be short-circuited by the scalp and another part will stimulate the cortex. Using non-metallic electrodes helps avoiding electrolysis reactions. Skin preparation might be useful to reduce the impedance of the skin/electrode interface. It is recommended to reach progressively the targeted stimulation intensity (generally a 10-second ramp at the beginning and at the end of the stimulation). Depending on the electrode polarity, the cerebral cortex excitability will be increased (anodal stimulation) or decreased (cathodal stimulation). The amplitude and the duration of the cortical excitability changes depend on the stimulation intensity and duration. Most published protocols have used intensities ranging from 1 to 2 mA, with stimulation duration up to 25 minutes, using 25 cm 2 to 35 cm 2 electrodes. In these circumstances, changes in corticospinal excitability last for more than one hour. A review describing the technique’s state of the art was proposed by Nitsche et al. .
1.2.1.3
Neurophysiological effects
In an original article, Nitsche and Paulus demonstrated the possibility to modulate cortical excitability by applying tDCS . The active electrode was placed over the primary motor cortex and the reference electrode was placed over the contralateral supraorbital region.
After a 4-seconds stimulation, the motor evoked potentials (MEP) amplitude was increased by about 25% after anodal stimulation and reduced by about 20% after cathodal stimulation. These changes in cortical excitability were measurable during the stimulation and also after it: this lasting effect was called “post-effect”. The duration of this post-effect depended on the intensity and on the duration of the stimulation.
1.2.1.4
Mechanisms of Transcranial Direct Current Stimulation
Human applications for tDCS are relatively new, but understanding of its action mechanisms is derived from animal experiments dating back to the sixties .
TDCS is different from other brain stimulation techniques since it doesn’t directly induce action potentials, unlike TMS and transcranial electrical stimulation (TES). Thus, it is considered as having a neuromodulation effect . It induces a polarization of brain tissues, enabling changes in neuronal excitability by modifying the resting membrane potential (depolarization by anodal stimulation, inducing an increased excitability or hyperpolarization by cathodal stimulation, triggering a decreased excitability).
Most authors agree that the post-effect is due to changes in synaptic effectiveness, similar to Long-Term Potentiation (LTP) and Long-Term Depression (LTD) . In a rat model , it was shown that anodal tDCS induced LTP, depending on NMDA receptors. Repeated low-frequency synaptic activation was required to induce this effect. Brain Derived Neurotrophic Factor (BDNF) secretion (that depends on the activation of NMDA receptors) and activation of its TrkB receptor are both associated with LTP. Those molecular mechanisms could explain the interpersonal differences in regards to neurostimulation effectiveness (varying genotypes for BDNF) . Furthermore, drugs interfering with NMDA receptors could modify the effectiveness of a neuromodulation .
1.2.1.5
Safety, adverse events and contraindication of Transcranial Direct Current Stimulation
Several thousand subjects have been stimulated with tDCS without any reported severe adverse event . Side effects of tDCS are well documented : sensation of tingling or rash at the electrode site (temporary at the beginning of the stimulation), erythematous skin rash (due to vasodilatation). One case of skin lesion has been reported in the literature (daily stimulations for one week), with spontaneous and complete healing in the 3 weeks following the end of the stimulation. Headaches, nausea or vertigo have been reported in some patients.
It is not recommended to use tDCS on persons with uncontrolled epileptic seizures. Furthermore, any circumstance lowering a person’s potential threshold for epileptic seizure is an exclusion criterion (alcoholism, sleep deprivation). Implanted device (pacemaker, cochlear implant, surgical clips and any intracranial material) is also an exclusion criterion to tDCS.
1.2.2
Transcranial Magnetic Stimulation
1.2.2.1
History
The ability to induce an involuntary muscle contraction with electrical stimulation of the primary motor cortex dates back to the beginning of the 20th century. Penfield , using cortical electrical stimulation during surgery, described the somatotopic organization of the primary motor cortex and the cortical homunculus. Later on, some TES trials were reported .
Anthony Barker, in 1985, proposed to use a magnetic field to stimulate the motor cortex : according to the principle initially described by Faraday in the 19th century, a magnetic field varying over time induces an electrical current in a conductive material. Barker identified the magnetic field intensity that produces an electrical field generating an action potential on the cerebral cortex.
Since then, TMS has become a powerful, non-invasive tool to study brain functions in neurophysiology and cognitive neurosciences labs . Functional integrity of the corticospinal tract, excitability of the cerebral cortex and brain plasticity can be explored with this tool.
1.2.2.2
Presentation of the technique
A high-intensity current is directed to a coil and generates the magnetic field. This very short-lived (< 1 ms), high intensity (1 to 3T) magnetic field is perpendicular to the scalp. It induces an electrical field. This electrical field, tangential to the scalp, generates action potentials on the pyramidal cells of the motor cortex via synaptic connections of cortical interneurons. These action potentials lead to peripheral muscle activation which can be recorded via surface electrodes (Motor Evoked Potential).
1.2.2.3
Repetitive Transcranial Magnetic Stimulation and excitability of the motor cortex
In the nineties, authors demonstrated that repetitive magnetic stimulations could change cortical excitability : eepeated high-frequency stimulations (≥ 5 Hz) increase cortical excitability while low-frequency stimulations (≤ 1 Hz) decrease it. This change in cortical excitability lasts beyond the stimulation time.
Therapeutic applications derive directly from this observation: any neurological or psychiatric disease that would be associated with cortical excitability modifications could become a therapeutic target. A large number of studies explored the supposed therapeutic effect of rTMS these past 15 years, mainly in neurology, neuro-rehabilitation (dystonia, Parkinson, stroke), psychiatry (depression, schizophrenia), but also in other medical fields (tinnitus, chronic pain) .
1.3
Brain stimulation and post-stroke rehabilitation: state of the art for upper-limb impairment
This literature review was based on English language publications indexed in PubMed reporting the use of tDCS or rTMS for functional rehabilitation in stroke patients with hemiparesis. The following terms were combined: “rTMS OR tDCS OR repetitive transcranial magnetic stimulation OR transcranial direct current stimulation OR cerebral stimulation” AND “stroke OR rehabilitation OR hemiparesis”. The main author of this manuscript selected from the titles and abstracts original articles or literature reviews. The references of the selected articles were also studied to find additional articles. Selected articles are listed in Tables 1–4 . Some of these studies will be described in details here, in order to illustrate the different strategies (inhibitory or excitatory tDCS or rTMS, or comparing both strategies, single session vs multiple sessions).
Authors, year | Type of study | Stimulation parameters | Patients | Post stroke delay | Evaluations | Significant results |
---|---|---|---|---|---|---|
Khedr et al., 2005 | Therapeutic, randomized, controlled vs sham, double blind study | Ipsilesional M1, 3 Hz, 10 trains of 10s separated by 50s, 1 per day for 10 days. Sham condition: coil at a distance from the scalp | 52 patients, ischemic stroke | 5–10 days | Barthel Index, Scandinavian Stroke Scale, NIHSS | Improvement of clinical parameters lasting 10 days |
Kim et al., 2006 | Randomized, controlled vs sham, cross-over, single blind study | Ipsilesional M1, 10 Hz, 80% of the motor threshold, 2s trains, 8 trains. Sham stimulation: coil at 90° | 15 patients stroke preserving M1 | > 3 months | Typing precision and speed on a numeric keyboard; amplitude of motor evoked potentials (MEP) | Improvement of the performance in the treated group correlated to the increased MEP amplitude |
Malcolm et al., 2007 | Randomized, controlled vs sham, blind study | Ipsilesional M1, 40 pulses at 20 Hz, 90% of the motor threshold, every 30s, 50 trains amounting to 2000 pulses. Sham: fake coil. Associated to constraint-induced therapy | 19 patients | > 1 year | Wolf Motor Function Test, Box and Block Test, Motor Activity Log | Improvement in both groups without any statistical difference, but initially heterogeneous groups. BBT improvement better in the rTMS group |
Yozbatiran et al., 2009 | Pilot safety study | Ipsilesional, 20 Hz for 20 minutes, single session | 12 patients with moderate to severe impairment | Mean of 4.7 years | Grasp strength, Purdue Pegboard Test | Modest improvement of grasp strength and dexterity, lasting after one week |
Ameli et al., 2009 | Controlled vs sham | Ipsilesional M1, 10 Hz, 80% of the motor treshold, 5s every 30s (1000 pulses). Sham: idem on the vertex | 29 patients | 1–88 weeks | Movement analyses of typing with the index finger and the hand, fMRI analysis | rTMS responders (sub-cortical stroke): normalization of the fMRI pattern. Improvement correlated to the recorded M1 initial activity of the affected hemisphere |
Koganemaru et al., 2010 | Randomized, controlled and cross-over study | 3 conditions: Eex: exercises of extension of the fingers and wrist TMS: rTMS 5 Hz, cortical representation of finger extensors, 100% of the motor threshold; 1 train of 8s every minute, 15 min Eex-TMS: association of both interventions | 9 patients, sub-cortical stroke; 9 healthy controls | > 5 months | Exp1: active extension amplitude, spasticity Exp2 (healthy subjects): cortical excitability parameters Exp3 (patients): 2 sessions/week, 6 weeks; spasticity and active extension | Exp 1: active extension, spasticity and grasp strength improved in the Eex-TMS condition Exp2: unchanged excitability in the Eex condition, diffuse increase in TMS condition, increase for the extensor in the Eex-TMS condition Exp3: improvement of the active extension (10–15°), active flexion (5–10°), spasticity and grasp strength |
Khedr et al., 2010 | Randomized, controlled vs sham, blind study | Ispilesional M1, 3 or 10 Hz, or sham stimulation 1/day during 5 consecutive days | 48 patients | < 1 week | Cortical excitability – Motor Impairment Scale, 1-year follow-up | Better improvement in the stimulated groups correlated to the changes in cortical excitability |
Authors, year | Type of study | Stimulation parameters | Patients | Delay post stroke | Evaluations | Significant results |
---|---|---|---|---|---|---|
Single session tDCS | ||||||
Fregni et al., 2005 | Randomized, controlled vs sham, cross-over study | M1: anodal over the ipsilesional hemisphere, cathodal over the contralesional hemisphere or sham, 1 mA, 35 cm 2 , 20 minutes | 6 patients | > 12 months | Jebsen Taylor Test | Performance improvement (6,8% with anodal stimulation, 11.7% with cathodal stimulation) |
Hummel et al., 2005 | Randomized, controlled vs sham, double-blind study | M1, ipsilesional anodal or sham, 1 mA, 25 cm 2 , 20 minutes | 6 patients, sub-cortical stroke | > 12 months | Jebsen Taylor Test | Performance improvement |
Hummel et al., 2006 | Randomized, vs sham, cross-over, double-blind study | M1, ipsilesional anodal or sham, 1 mA, 25 cm 2 , 20 minutes | 11 patients, sub-cortical stroke | > 12 months | Reaction time, pinch strength | Performance improvement |
Celnick et al., 2009 | Randomized, cross-over, blind study | M1, ipsilesional anodal associated with peripheral stimulation | 9 patients | > 30 months | Typing speed on a keyboard | Performance improvement after combined stimulation of M1 and peripheral nervous system |
Multiple tDCS sessions | ||||||
Boggio et al., 2007 | Randomized, vs sham, double-blind and cross-over study | M1: anodal over the ipsilesional hemisphere, cathodal over the contralesional or sham, 1 mA, 35 cm 2 , 20 minutes; 1 weekly session for 1 month or 1 daily session during 10 consecutive days | 9 patients, sub-cortical stroke | > 12 months | Jebsen Taylor Test | Transitory motor improvement after anodal and cathodal weekly stimulation. Cumulative and lasting effect with daily stimulation |
Hesse et al., 2007 | Safety and feasibility pilot study | Ipsilesional anodal M1, 1.5 mA, 7 minutes, during daily robot-assisted rehabilitation, 6 weeks | 10 patients, cortical (8) or sub-cortical (2) stroke, severe impairment (Fugl Meyer < 18) | 4–8 weeks | Fugl Meyer, Aachener aphasia test | 3 patients with clinical improvement including 2 with sub-cortical stroke. Unexpected improvement of phasic disorders |
Lindenberg et al., 2010 | Randomized, vs sham | M1: anodal over the ipsilesional hemisphere, cathodal over the contralesional hemisphere, 1.5 mA, 30 minutes, or sham stimulation 5 consecutive days, associated with physical therapy | 20 patients Sylvian ischemic stroke. Sham group: 10 patients | > 5 months | Fugl Meyer (upper limb) and Wolf Motor Function Test | Significant improvement in the treated group, lasting one week after the intervention |
Kim et al., 2010 | Randomized, controlled vs sham, double blind | M1: anodal over the ipsilesional hemisphere, cathodal stimulation over the contralesional, 2 mA, 25 cm 2 , or sham – 10 sessions associated with occupational therapy | 18 patients | < 2 months | Fugl Meyer (upper limb) and Barthel Index | Functional improvement in the three groups. Significantly better improvement of the Fugl Meyer score in the cathodal stimulation group at 6 months |
Authors, year | Type of study | Stimulation parameters | Patients | Post stroke delay | Evaluations | Significant results |
---|---|---|---|---|---|---|
Takeuchi et al., 2005 | Randomized, controlled vs sham, double-blind study | Contralesional M1, 1 Hz, 90% of the motor threshold, 25 minutes (1500 pulses). Sham condition: coil at 90° | 20 patients, sub-cortical stroke | > 6 months | Amplitude of MEPs; pinch strength | Decreased MEP amplitude and interhemispheric inhibition after real stimulation. Improvement of movement speed of the affected hand |
Mansur et al., 2005 | Randomized, cross-over, blind study | Contralesional M1 or premotor cortex or sham (fake coil) 1 Hz, 100% of the motor threshold, 10 minutes (600 pulses) | 10 patients | < 12 months | Reaction time, Purdue Pegboard Test, typing speed | Improvement of reaction time and of dexterity after M1 stimulation vs sham |
Fregni et al., 2006 | Therapeutic phase II randomized, vs sham, double-blind study | Contralesional M1, 1 Hz, 1200 stimuli (20 minutes), 5 consecutive days or Sham: fake coil | 15 patients ischemic stroke | > 12 months | Corticospinal excitability, reaction time, Purdue Pegboard Test, Jebsen Taylor Test | Improvement of reaction times and motor performances in the treated group, lasting 2 weeks. Decreased corticospinal excitability of the unaffected hemisphere, increase of the affected hemisphere |
Liepert et al., 2007 | Randomized, vs sham, cross-over study | Contralesional M1, 1 Hz, 90% of the motor threshold, 1200 pulses or sham | 12 patients sub-cortical stroke | Acute phase (mean 7 days post-stroke) | Grasping strength, Nine Hole Peg Test | Transitory improvement of the dexterity in the real stimulation group |
Takeuchi et al., 2008 | Controlled, Randomized, vs sham, double-blind study | Contralesional M1, 1 Hz, 25 minutes, or sham | 20 patients, sub-cortical ischemic stroke | > 7 months | Cortical excitability, thumb-finger grasp | Improvement of the kinematic parameters and grasp strength of the impaired limb in the stimulated group |
Nowak et al., 2008 . | Controlled, vs sham, cross-over study | Contralesional M1 or vertex, 1 Hz, 10 minutes, 100% of the motor threshold | 15 patients, deep sylvian stroke | 1–4 months | Clinical parameters (typing speed of the index finger, grasp) and fMRI (grasping tasks) | Stimulation M1: improvement of the kinematic parameters, normalization of fMRI activation pattern |
Dafotakis et al., 2008 | Controlled vs sham | Contralesional premotor cortex, 1 Hz, 10 min or sham (vertex) | Sub-cortical stroke | 1–4 months | Grasp analysis | Improvement of the kinematic parameters in the stimulated group |
Mally and Dinya, 2008 | Pilot study | 4 groups according to the MEP profile, 1 Hz, 100 pulses, 30% of 2.3 Tesla, 2 sessions per day, 5 days | 46 patients | > 5 years | Spasticity, movement and use scores. 3-month follow-up | Improvement of spasticity in all groups. Motor improvement was group-dependent |
Kirton et al., 2008 | Randomized, vs sham, blind study | Contralesional M1, 1 Hz, 20 min, 8 consecutive days, or sham | 10 children > 7 years | > 2 years | Grasp strength, Melbourne Assessment of upper extremity function | Improvement of grasp strength at day 10, lasting up to day 17. Improvement of the clinical score at day 10 |
Grefkes et al., 2010 | Controlled vs sham | Contralesional M1, 1 Hz, 10 min or sham (vertex) | 11 patients | 1–3 months | fMRI evaluation, hand closure, at 1 and 3 months | Improvement of motor parameters in the stimulated group, correlated to the inhibitory influence of contralesional M1 on the affected hemisphere. Increase in the ipsilesional SMA-M1 coupling |
Authors, year | Type of study | Stimulation parameters | Patients | Post stroke delay | Evaluations | Significant results |
---|---|---|---|---|---|---|
Talelli et al., 2007 | Randomized vs sham | Ipsilesional M1, excitatory (iTBS, 3 to 5 100 Hz pulses, repeated at 5 Hz) or inhibitory: cTBS or sham | 6 patients | > 1 year | Reaction time (grasping task), MEP amplitude | Decreased reaction time and increased MEP amplitude after iTBS. Decreased MEP amplitude after cTBS |
Di Lazzaro et al., 2008 | Pilot study | Concomitant stimulations on ipsilesional M1 (excitatory TBS) and contralesional M1 (inhibitory TBS) | 12 patients and 12 healthy controls | < 10 days | MEP amplitude | Increased of the excitability of the affected hemisphere |
Khedr et al., 2009 | Randomized, controlled vs sham, double-blind study | Ipsilesional M1, 3 Hz, 130% of the motor threshold, 30 trains of 10 s or Contralesional M1, 1 Hz, 15 minutes, 100% of the motor threshold or sham (coil at a distance from the scalp). 1/day, 5 consecutive days | 3 groups of 12 patients, Sylvian ischemic stroke | 7 to 12 days | Cortical excitability, dexterity (Purdue Pegboard Test, typing speed), NIHSS, Barthel Index | Clinical improvement at 3 months, better in the “1 Hz” and “3 Hz” groups, better for the “1 Hz” vs “3Hz” group “1 Hz” group: decreased excitability of the stimulated cortex increased contralateral excitability “3 Hz” group: increased excitability of the stimulated cortex |
Emara et al., 2010 | Randomized, controlled vs sham, blind study | Ipsilesional M1, 5 Hz, 80–90% of the motor threshold, 150s or contralesional M1, 1 Hz, 110–120% of the motor threshold, 150s or sham: coil at 90° 1/day, 10 consecutive days | 3 groups of 20 patients, ischemic stroke | > 1 month | Dexterity (typing speed), modified Rankin Scale, Activity Index Scale | Improvement of typing speed, activity index and autonomy in the stimulated groups, lasting up to 12 weeks |
Previous studies essentially proposed single session stimulation protocols that included few patients at a chronic stage. Kinematic movement parameters (alternative movements such as typing on a keyboard, grasping movements) measures of cortical excitability were evaluated. Functional improvements using stimulation were demonstrated and improvements were correlated to changes in cortical excitability. However, the short-lasting nature of the functional improvement after a single stimulation session was consistently described.
In recent papers, the number of sessions had increased (most often one daily session during 5 to 10 consecutive days). Stimulations were performed during the acute or subacute phase of the neurological event and the number of included patients was higher. Evaluations were most often targeted at upper-limb functional improvement, with specific scales (for example the Jebsen Taylor Test [JTT] ), but also non-specific scales evaluating patient’s autonomy (Functional Independence Measure scale , Barthel index , modified Rankin Scale ).
Finally, the most recently published papers study the effects of stimulation on cortical activation patterns during motor tasks with functional MRI.
1.3.1
Bases
The application of neuromodulation in stroke patients with hemiplegia is based on: reducing interhemispheric imbalance and improving brain plasticity.
1.3.1.1
Interhemispheric imbalance
Through transcallosal connections, each hemisphere has an inhibitory activity on the other one . After a stroke, corticospinal excitability increases in the unaffected hemisphere and decreases in the affected one : the inhibitory activity by the affected hemisphere on the healthy one is decreased because of the lesion. The increased excitability of the unaffected hemisphere could induce an overemphasized inhibitory activity on the affected hemisphere; this inhibition could have a functional impact, the impaired hemisphere being twice penalized, by the vascular lesion itself and by this exaggerated transcallosal inhibition.
The functional significance of this increased excitability in the unaffected hemisphere is still under debate . Some authors have interpreted it as a manifestation of the brain’s ability to adapt to the lesion and considered it as the trace of the unaffected hemisphere’s role in functional recovery. Conversely, some have considered it as a determining factor in the impairment’s pathophysiology . In spite of the debatable nature of this interpretation, most authors that proposed neurostimulations have based their studies on this theory to justify the intervention : it would be possible to improve the function of the impaired limb if one was able to restore this interhemispheric imbalance ( Fig. 1 ). This is possible by either increasing the excitability of the affected hemisphere (high frequency rTMS or anodal tDCS), or decreasing the excitability of the unaffected hemisphere (low frequency rTMS or cathodal tDCS).
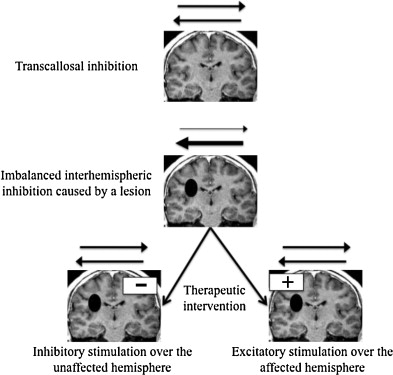
1.3.1.2
Brain plasticity potentiation induced by the exercise
Results in healthy subjects showed an improvement in motor learning with excitatory stimulation of the contralateral primary motor cortex . According to the hypothesis that rehabilitation of the paretic upper limb is comparable to motor learning process , we can extrapolate that neurostimulation could potentiate motor learning in stroke patients with hemiplegia as shown by Koganemaru et al. .
1.3.2
Single-session studies
1.3.2.1
Excitatory strategy of the affected hemisphere
1.3.2.1.1
High-frequency repetitive Transcranial Magnetic Stimulation
Kim et al. included 15 patients and evaluated the effects of excitatory rTMS associated to a specific motor task on motor performance and on cortical excitability ( Table 1 ). This was a sham-controlled, cross-over and single-blind study. The patients were included at a chronic stage.
When stimulation was real, a significant improvement of the task’s performance was noted, (movement accuracy and speed) and MEP amplitude had significantly increased. This study suggested the capacity of an excitatory stimulation of the affected hemisphere to improve upper-limb function of stroke patients. This improvement would be correlated with excitability changes of the primary motor cortex of the affected hemisphere. More recent studies validate this result ( Table 1 ).
1.3.2.1.2
Anodal Transcranial Direct Current Stimulation
Hummel et al. proposed methodological bases to evaluate the effect of one session of tDCS ( Table 2 ). Anodal excitatory tDCS was applied on the primary motor cortex of the affected hemisphere during a test of Activities of Daily Living, the JTT. The effect on patients’ motor performance was compared to sham stimulation. Six patients were included at a chronic stage. After a familiarization period, patients participated in two experimental sessions (real or sham stimulation), in a random order, in a cross-over and double-blind study design.
With real stimulation, a significant performance improvement was shown compared to sham stimulation; this improvement was associated with an increased cortical excitability of the affected hemisphere. Furthermore, patients were not able to differentiate the type of stimulation conducted (real or sham) and there was no difference between both conditions in terms of discomfort or pain. The effect lasted at least 25 minutes beyond the stimulation, but disappeared during the post-test evaluation (11 days later).
1.3.2.2
Inhibitory strategy of the unaffected hemisphere
1.3.2.2.1
Low-frequency repetitive Transcranial Magnetic Stimulation
A placebo-controlled, double-blind study (20 patients, more than 6 months after an ischemic, sub-cortical stroke) evaluated the influence of inhibitory rTMS on cortical excitability and on the performance for a simple motor task (pinch test) ( Table 3 ). Results suggested a significant decrease (about 20%) of MEP amplitude by stimulation of the contralesional motor cortex, as well as improved motor performance. A significant decrease of interhemispheric inhibition was correlated to an increased peak in movement acceleration.
Recent studies ( Table 3 ) validate this result and suggest that motor performances can be improved using inhibitory rTMS on the unaffected hemisphere; this functional improvement was associated to decreased excitability on the unaffected hemisphere. These results underline the functional importance of interhemispheric competition in these patients.
1.3.2.2.2
Inhibitory cathodal Transcranial Direct Current Stimulation
Based on the same protocol than Hummel et al. , excitatory and inhibitory stimulations were compared. Authors observed respectively an 11.7% and 6.8% improvement after cathodal and anodal stimulations, and a rather degraded performance after sham stimulation ( Table 2 ). The improvement was statistically significant for the two real stimulations, compared to sham. One week after stimulation, patients went back to their initial motor performances. There was no significant difference between the two stimulation strategies.
1.3.2.3
Relevance of a single stimulation session in stroke rehabilitation
Results from the different studies proposing a single stimulation session were quite coherent, whether with rTMS or tDCS: both techniques, with excitatory stimulation of the affected hemisphere or inhibition of the unaffected one, can lead to a transitory functional improvement of the impaired upper limb.
Nevertheless, transitory functional improvement has little relevance in neurorehabilitation. This is why most of the recent studies proposed repetitive sessions. The goal was to determine whether the improvement could be cumulative and long lasting.
1.3.3
Studies on repeated sessions
1.3.3.1
Repeated Transcranial Direct Current Stimulation sessions
Boggio et al. conducted a randomized, sham-controlled, double-blind, cross-over study using the methodology proposed by Hummel et al. . Patients were included at a chronic stage (more than 12 months from a sub-cortical ischemic stroke). In a first experience, the authors evaluated the relevance of a weekly stimulation session (anodal or cathodal vs sham) for one month. The improvement described in previously published studies was validated after each of the sessions (7.3% improvement with anodal stimulation and 9.5% improvement with cathodal stimulation). However, no long-lasting effect was observed.
In a second experience, the patients received one daily cathodal stimulation of the unaffected hemisphere during 5 consecutive days. A cumulative effect was measured (total of 16.5% of improvement) which lasted during the follow-up evaluations (one and two weeks after the intervention). However, this last experience had no control group; thus, the effectiveness of the technique with the proposed dosage was not validated.
Nevertheless, this result seems quite promising since patients were included at a distance from their ischemic stroke, had all stabilized their upper-limb function before inclusion in the protocol, and were familiarized with the test before treatment in order to ensure scores’ stability before the intervention.
The effect of repeated tDCS (5 consecutive days) with both excitatory stimulation of the affected hemisphere and inhibitory stimulation of the unaffected one was evaluated in a recent study . This was a placebo-controlled, randomized, double-blind study. Patients were included at least 5 months after an ischemic stroke. They benefited from stimulation during their rehabilitation sessions. The evolution of upper-limb motor functions was better in the stimulated group (20.7% according to the Fugl Meyer score, vs 3.2% in the sham group) with a long-lasting effect observed one week post-treatment. This last study underlines the potential relevance of bihemispheric stimulations.
1.3.3.2
Repeated repetitive Transcranial Magnetic Stimulation sessions
Very promising results have been published ( Tables 2 and 3 ). Khedr et al. included 52 stroke patients recruited in the acute phase, randomly assigned into two groups (excitatory or sham stimulation). Evaluations (Scandinavian Stroke Scale, National Institute of Health Stroke Scale [NIHSS], Barthel Index) were performed before treatment, immediately after the last stimulation session and finally 10 days later. The functional improvement was significantly better in the treated group vs sham. During the last evaluation, there were significantly more independent patients (Barthel score > 75) in the treated group; this last result appears to have a high clinical relevance. However, the impact of this study is limited by its short follow-up period (only one month post stroke).
Fregni studied the relevance of daily inhibitory stimulation sessions of the healthy cortex vs placebo in 15 patients with an ischemic stroke with mild to moderate impairment. Neurophysiological (MEP amplitude) and clinical (reaction time, JTT, Purdue Pegboard test) parameters were evaluated before, during and 2 weeks after treatment. The various clinical parameters were evaluated for the impaired and healthy upper limb. Results showed an improvement of clinical parameters for the impaired limb that lasted up to 2 weeks post-treatment in the stimulation group but not in the control group. This improvement was correlated to increased cortical excitability of the affected hemisphere and decreased excitability in the unaffected one.
Finally, two recent publications described clinical trials withrespectively 36 and 60 patients. Therapeutic effect of repeated rTMS sessions, either with stimulation of the affected hemisphere or with inhibition of the unaffected one vs sham was evaluated. Khedr et al. included patients in the acute phase post stroke. Thirty-six patients were randomly affected into three groups (inhibitory rTMS of the unaffected hemisphere, excitatory rTMS of the affected hemisphere, placebo stimulation). Patients had a daily rTMS session during 5 consecutive days, and benefited from usual medical and rehabilitation treatments. Neurophysiological (cortical excitability of both hemispheres) and clinical (motor testing, typing speed, Purdue Pegboard test, NIHSS, Barthel index) evaluations took place before the intervention, during treatment and at M1, M2 and M3 follow-up visits. Each group showed significant improvement of performances over time for all various parameters. In groups with real stimulation, patients showed significantly better improvements with dexterity, NIHSS score and Barthel Index. Furthermore, patients in the group with inhibitory rTMS showed better improvements than the group with excitatory rTMS. Improvements are quite important: 81% (Barthel index at 3 months for the inhibitory rTMS- group) vs 33% (sham group). Further prospective studies seem necessary to validate this approach.
Neurophysiological measures showed, as expected, a decreased excitability of the unaffected hemisphere with the 1 Hz stimulation, but also an increased excitability of the affected hemisphere. With the 3 Hz stimulation, the excitability of the affected hemisphere had increased, but no significant effect was measured on the unaffected hemisphere. The results thus suggest that inhibitory stimulation would be more effective on interhemispheric imbalance than excitatory stimulation of the affected hemisphere, with both a local impact (decreased excitability of the underlying cortex), but also at distance (increased excitability of the contralateral hemisphere). This improved balance of the interhemispheric inhibition could have a more effective clinical effect.
Clinical improvements are more important than in previous studies (33 to 69% according to the score used vs sham). The authors hypothesized that this better improvement could be attributed to the fact that the intervention was performed during the acute phase, when brain plasticity was more important.
These findings were consistent with a recent study that support a significant improvement in the stimulated groups according to an independence scale (modified Rankin Scale) without any impact on the control group.
1.3.4
Functional MRI activation pattern
1.3.4.1
Inhibitory stimulation of the unaffected hemisphere
It is commonly admitted that the fMRI activation pattern during motors tasks is different in stroke patients than in healthy subjects, and that this pattern changes during recovery . Furthermore, the quality of functional motor recovery is better in patients regaining motor cortex activity in the affected hemisphere . A question is whether these activation patterns can be modified by neurostimulation and whether these changes could be correlated to motor improvements.
Nowak et al. studied the impact of inhibitory rTMS on the unaffected hemisphere in 15 stroke patients with motor disorders. Kinematic movement parameters of the upper limb and associated fMRI activation pattern were assessed.
fMRI activation associated to movements of the healthy upper limb was comparable to the activation described in healthy subjects (contralateral M1, bilateral supplementary motor area, bilateral premotor cortex and bilateral occipital cortex). With movements on the healthy side, the stimulation condition had no impact on the activation pattern. No kinematic changes were noted.
fMRI activation associated to movements on the impaired side was comparable to that described in previous publications (contralateral M1, bilateral supplementary motor area, bilateral premotor cortex and bilateral occipital cortex, but also a wider and more diffuse bilateral activity, mainly frontal and parietal). This activation pattern was not modified in the control group, but was modulated by an inhibitory stimulation of the contralesional motor cortex: cortical activations could be normalized after a stimulation. This change to fMRI activation pattern was associated to an improvement of motor parameters.
1.3.4.2
Excitatory stimulation of the affected hemisphere
This strategy has also been explored with Ameli et al. . Twenty-nine patients with middle cerebral artery stroke were recruited. The behavioral evolution (frequency, amplitude and typing speed of the index and the hand) was evaluated before and after excitatory primary motor cortex stimulation of the affected hemisphere. In the control group, the stimulation was applied on the vertex.
Depending on their behavioral response, patients were classified into two groups: “rTMS responders” (significant improvement of kinematic parameters) and “rTMS non-responders” group (no significant improvement or slight deterioration). Fourteen of 16 patients whith sub-cortical lesion did respond to stimulation. None of the patients with a cortical stroke responded to stimulation. Furthermore, the pathological fMRI motor cortex activation ipsilateral to the movement was decreased after stimulation for 11 of the patients with sub-cortical stroke. For seven patients with cortical stroke, the excitatory rTMS induced a diffuse bilateral recruitment of primary motor and sensory areas. Finally, the clinical improvement induced by the stimulation was correlated to the initial activity of the ipsilesional motor cortex.
These results suggest interesting information regarding the excitatory strategy of the affected hemisphere. This strategy could also normalize the fMRI activation pattern associated to a motor task, and induce motor improvement. However, this strategy does not seem adapted to patients with cortical lesions of primary motor or sensory areas.
1.3.5
Potentiation of use-dependent plasticity
Koganemaru et al. focused on the concept of use-dependent plasticity and explored the possibility to potentiate plasticity with repetitive excitatory TMS of the hemisphere corresponding to the trained limb. Nine patients (sub-cortical stroke) were included in three experimental sessions:
- •
“Eex” session (training exercises with active extension of the impaired fingers and the wrist, triggered by functional electrical stimulation of the extensor muscles);
- •
“TMS” session (high-frequency rTMS above the motor cortex);
- •
“Eex-TMS” session (associating both interventions).
Motor function (active fingers and wrist extension amplitude) and spasticity (modified Ashworth Scale) were evaluated before and after the intervention. Theses parameters did not change during the “Eex” and “TMS” sessions, but significantly improved after the “Eex-TMS” session.
The same experimental sessions were proposed to nine healthy subjects, in order to evaluate the cortical excitability changes induced by these interventions: in the “Eex” condition, no neurophysiological change could be evidenced; in the “TMS” condition, as expected, there was a diffuse increase of the motor cortex excitability for both the flexor and extensor muscles. Finally, in the “Eex-TMS” condition, the authors reported an excitability increase specific to the trained muscles (common extensor of the fingers): there was no excitability change in the cortical representation of flexor muscles.
These two experiences convincingly illustrate the possibility to potentiate use-dependent plasticity with neuromodulation. It seems therefore relevant to combine neuromodulation with physical therapy for clinical applications.
1.4
Conclusion
There are numerous arguments to suggest that tDCS and rTMS may become interesting tools to promote upper limb motor recovery in stroke patients. The ability of these techniques to modulate cortical excitability was demonstrated. Several experimental results suggest an improvement of performances, transitory after a single session and maybe long lasting after repeated sessions. Finally, functional imaging has also shown the effectiveness of these stimulations on the cortical activation associated to a movement.
To our knowledge, only one study has reported negative results with an association of excitatory rTMS combined to constraint-induced therapy ; however, in this study, treated and control group (rTMS and sham rTMS) were heterogeneous before the intervention.
The current research has provided preliminary evidence for the feasibility and clinical efficacy of neurostimulation after stroke. Research identified factors predictive of improvement (repeated daily sessions, performed at the acute phase post ictus). Furthermore, it is essential to note that these tools are adjuvant therapies, able to potentiate physical therapy.
However, limitations to the research methods (number of patients, evaluated parameters far from the clinical needs) exist. Further prospective studies including detailed ADL data and large sample are needed to confirm these findings.
Disclosure of interest
The authors declare that they have no conflicts of interest concerning this article.
2
Version française
2.1
Introduction
L’optimisation de la prise en charge des accidents vasculaires cérébraux (AVC) est actuellement une priorité nationale. La prévalence est estimée à 400 000 personnes en France, et l’AVC représente la troisième cause de mortalité chez l’homme, la deuxième chez la femme, et la première cause de handicap acquis de l’adulte 1
1 Plan d’actions national « accidents vasculaires cérébraux 2010–2014 », ministère de la Santé et des sports.
. Plus de 50 % des patients conservent à la suite d’un AVC des déficiences neurologiques sévères, le plus souvent motrices . Parmi ces patients, environ 80 % conserveront un déficit de la préhension lié à une atteinte motrice d’un membre supérieur.L’amélioration de la fonction de préhension est un enjeu majeur de la rééducation, avec des résultats encore décevants . En pratique clinique quotidienne, les principes de rééducation du membre supérieur sont aujourd’hui encore largement inspirés des écoles de rééducation ayant émergé depuis le milieu du xx e siècle , mais on a assisté ces 20 dernières années à des évolutions conceptuelles majeures , nourries par les neurosciences fondamentales, notamment avec la démonstration par l’imagerie cérébrale de la plasticité cérébrale. La notion d’apprentissage prend une part importante, et la notion de rééducation orientée sur la tâche s’impose peu à peu . Les concepts de non-utilisation acquise, initialement décrite par Meige , et de compétition interhémisphérique sont de plus en plus communément admises , et un certain nombre d’interventions sont proposées, visant à rééquilibrer cette compétition en faveur de l’hémisphère lésé.
Les stimulations cérébrales, invasives ou non invasives, permettent de moduler l’excitabilité corticale et de potentialiser la plasticité cérébrale. Elles ont déjà largement été explorées en psychiatrie et en algologie . En rééducation post-AVC, des résultats intéressants dans l’amélioration des troubles phasiques , du syndrome d’héminégligence et des troubles de la déglutition sont rapportés. La place de ces traitements reste cependant à définir dans la pratique clinique.
La Transcranial Direct Current Stimulation ou stimulation transcrânienne par courant continu (tDCS), la repetitive Transcranial Magnetic Stimulation ou stimulation magnétique transcrânienne répétitive (rTMS) et la Paired-Associative Stimulation (PAS ) sont des techniques non invasives. Elles s’opposent à des essais de neurostimulation invasive réalisés à l’aide d’électrodes épidurales implantées, avec des essais de phase I, II et III peu concluant .
L’objectif de cet article est de décrire deux de ces techniques, susceptibles d’être utilisées en milieu de rééducation neurologique : la tDCS et la rTMS, et de proposer une revue de la littérature concernant leur application dans la rééducation du déficit moteur du membre supérieur consécutif à un AVC.
2.2
Les stimulations cérébrales : données générales
2.2.1
La stimulation transcrânienne par courant continu
2.2.1.1
Historique
L’utilisation thérapeutique de courants électriques à travers le scalp a été proposée depuis l’antiquité : écrits décrivant l’utilisation de poissons torpilles pour le soulagement de céphalées, chez des auteurs tels que Scribonius Largus, Claudius Galenus, et Pline le Vieux. Au xi e siècle, le médecin arabe Ibn Sidah a utilisé ce moyen pour le traitement de l’épilepsie .
Les prémisses de l’électrophysiologie ont été proposées, suite aux travaux de Walsh sur le poisson torpille , par les physiciens Italiens Galvani et Volta, qui ont décrit les effets physiologiques induits par des courants électriques . Giovanni Aldini a proposé, quelques années après, d’utiliser des courants galvaniques dans le traitement de maladies mentales .
Récemment, des connaissances neurophysiologiques ont suscité un regain d’intérêt pour l’utilisation de courants continus sur le scalp : en effet, l’apparition de la stimulation magnétique transcrânienne (TMS) comme outil de mesure de l’excitabilité corticale a permis de mettre en évidence les niveaux d’intensité de courant, les durées de stimulation, et les positions d’électrodes qui ont un effet significatif sur l’excitabilité corticale. Priori et al. ont observé une modulation de l’amplitude de potentiel évoqué moteur (PEM) par une TMS suite à une stimulation par courant continu du cortex moteur primaire (M1). Cela a été confirmé et développé par Nitsche et Paulus, qui ont jeté les bases des paradigmes de stimulation actuels .
2.2.1.2
Présentation de la technique
La tDCS consiste en l’application d’un courant électrique de faible intensité entre deux électrodes placées sur le scalp. Une électrode, dite « active » est placée en regard de la région cérébrale que l’on veut stimuler, et une électrode de référence est placée sur une position neutre. Une partie du courant sera court-circuitée par le scalp et une partie du courant stimulera le cortex cérébral. L’utilisation d’électrodes non métalliques permet d’éviter la survenue de réactions d’électrolyse au niveau du scalp. Une préparation cutanée peut être utile afin de diminuer l’impédance de l’interface peau/électrode. Il est recommandé d’atteindre l’intensité de stimulation voulue de manière progressive (en général, rampe de dix secondes au début et à la fin de la stimulation). Selon la polarité de l’électrode, l’excitabilité du cortex cérébral sera augmentée (anode) ou diminuée (cathode). L’amplitude et la durée de la modification de l’excitabilité corticale dépendent de l’intensité et de la durée de la stimulation. La plupart des protocoles publiés utilisent des intensités de 1 à 2 mA, avec une durée de stimulation pouvant aller jusqu’à 25 minutes, à travers des électrodes de 25 à 35 cm 2 . La durée de modification de l’excitabilité cortico-spinale est, dans ces conditions, supérieure à une heure.
Une revue décrivant l’état de l’art de la technique a été proposée par Nitsche et al. .
2.2.1.3
Effets neurophysiologiques
Nitsche et Paulus ont démontré dans un article princeps la possibilité de moduler l’excitabilité corticale par l’application de tDCS , évaluée par l’amplitude des PEM du muscle court abducteur du V évoqués par TMS ; l’électrode active était placée en regard du cortex moteur primaire, et l’électrode de référence était placée en zone supraorbitaire controlatérale. Après stimulation de quatre secondes, l’amplitude du PEM était augmentée d’environ 25 % suite à une stimulation anodique, et diminuée de 20 % environ suite à une stimulation cathodique. Ces modifications d’excitabilité sont mesurables pendant la stimulation, mais aussi après celle-ci : cet effet, dépassant la durée de la stimulation, a été nommé « effet consécutif » ou « post effet ». La durée de l’effet consécutif dépend de l’intensité et de la durée de la stimulation.
2.2.1.4
Mécanismes d’action de la stimulation transcrânienne par courant continu
La tDCS est une technique relativement récente dans ses applications à l’homme, mais les connaissances quant à ses mécanismes d’action sont nourries des expérimentations animales ayant eu lieu dans les années 1960, notamment chez le rat .
La tDCS se distingue des autres techniques de stimulation cérébrale par le fait qu’elle n’induit pas directement de potentiels d’action, à la différence de la TMS et de la stimulation électrique transcrânienne (TES). Elle est donc considérée comme une intervention ayant un effet de neuromodulation . Son application aux tissus cérébraux induit une polarisation de ces tissus, qui permettrait de modifier l’excitabilité des neurones, par modification du potentiel membranaire de repos (dépolarisation par stimulation anodique, induisant une augmentation de l’excitabilité, ou hyperpolarisation par stimulation cathodique, induisant une diminution de l’excitabilité).
S’il est relativement simple de comprendre l’effet neurophysiologique d’une neuromodulation pendant la stimulation, la rémanence de la modification d’excitabilité (effet consécutif) est plus difficilement comprise. La plupart des auteurs s’accordent pour penser que ces effets consécutifs sont la conséquence de modifications d’efficacité synaptique, comparables aux phénomènes largement décrits en neurosciences fondamentales de Long-Term Potentiation (LTP) et Long-Term Depression (LTD) . Il a été montré chez le rat qu’une tDCS anodique induit une potentialisation synaptique à long terme (LTP), dépendant des récepteurs NMDA, et nécessite un couplage avec une activation synaptique répétée à basse fréquence. La sécrétion d’une protéine ( Brain Derived Neurotrophic Factor [BDNF] dont la sécrétion dépend de l’activation de récepteurs NMDA) et l’activation de son récepteur TrkB sont associées à la LTP. Ces mécanismes moléculaires expliquent, d’une part, les différences d’efficacité des neurostimulations entre sujets présentant différents génotypes pour le gène codant pour la protéine BDNF , d’autre part, les modifications de l’efficacité des neurostimulations par des médicaments interférant avec le fonctionnement des récepteurs NMDA .
2.2.1.5
Sécurité, effets indésirables et contre-indications de la stimulation transcrânienne par courant continu
On peut évaluer le nombre de sujets ayant reçu une tDCS à plusieurs milliers , sans qu’il ait été rapporté d’effet indésirable grave. Les effets secondaires de la stimulation par tDCS sont bien documentés. Parmi les effets secondaires notables , on retiendra une sensation de picotements ou de prurit en regard des électrodes (transitoire en début de stimulation), une réaction cutanée érythémateuse (transitoire, consécutive à une vasodilatation). Un cas de lésion cutanée est rapporté dans la littérature (stimulations quotidiennes pendant une semaine), avec une guérison spontanée et complète dans les trois semaines suivant l’arrêt de la stimulation. Des céphalées, nausées ou sensations vertigineuses ont pu être rapportées chez quelques sujets.
Il n’est pas recommandé d’appliquer une tDCS à un sujet présentant une épilepsie non contrôlée. De même, toute situation diminuant le seuil épileptogène est une contre-indication relative (éthylisme non sevré, dette de sommeil). Enfin, la présence de matériel implanté (pacemaker, implant cochléaire, clips chirurgicaux, tout matériel intracrânien) contre-indique l’application de tDCS.
2.2.2
Stimulation magnétique transcrânienne
2.2.2.1
Historique
La capacité d’induire, à l’aide d’une stimulation électrique au niveau du cortex moteur primaire, un mouvement involontaire, date du début du xx e siècle. Penfield et al. , à l’aide de stimulations électriques corticales per-chirurgicales, ont décrit l’organisation somatotopique au niveau du cortex moteur primaire, et l’homoncule inversé. Par la suite, des essais de stimulations électriques à travers le scalp (TES) ont été réalisées .
Anthony Barker, en 1985, a proposé l’utilisation d’un champ magnétique pour stimuler le cortex moteur : selon le principe décrit initialement par Faraday au xix e siècle, un champ magnétique variable dans le temps induit un courant électrique dans un milieu conducteur. Barker a identifié l’intensité de champ produisant un champ électrique capable de générer un potentiel d’action au niveau du cortex cérébral, de manière indolore et avec une innocuité désormais bien établie.
Depuis ces travaux, la TMS est devenue un outil puissant, non invasif, d’étude du fonctionnement cérébral dans les laboratoires de neurophysiologie et de neurosciences cognitives . Elle permet d’explorer l’intégrité fonctionnelle du faisceau cortico-spinal, l’excitabilité du cortex cérébral et la plasticité cérébrale.
2.2.2.2
Présentation de la technique
Afin de générer le champ magnétique, un courant de haute intensité et de très courte durée est imposé à une bobine (ou coil). Le champ magnétique, de très courte durée (< 1 ms) et de haute intensité (1 à 3T), perpendiculaire au scalp, induit un champ électrique (nommé champ électrique induit), tangentiel au scalp. Ce champ électrique induit génère des potentiels d’action au niveau des cellules pyramidales du cortex moteur via des connections synaptiques d’interneurones corticaux. Ces potentiels d’action conduisent à une activation musculaire périphérique, qu’il est possible d’enregistrer à l’aide d’électrodes de surface (PEM).
2.2.2.3
Stimulation magnétique transcrânienne répétitive et excitabilité du cortex moteur
Au milieu des années 1990, il a été montré qu’une répétition des stimulations magnétiques avait la capacité de modifier l’excitabilité corticale . Ainsi, à l’aide de stimulations répétées à haute fréquence (≥ 5 Hz), l’excitabilité corticale est augmentée ; avec des stimulations à basse fréquence (≤ 1 Hz), l’excitabilité corticale est diminuée. Cette modification d’excitabilité dépasse le temps de stimulation.
Les applications thérapeutiques potentielles découlent directement de ce constat : toute pathologie neurologique ou psychiatrique s’accompagnant d’une modification de l’excitabilité corticale est une cible potentielle. On a donc assisté ces 15 dernières années à une explosion du nombre d’études dont l’objectif est d’explorer l’effet thérapeutique supposé d’une rTMS, essentiellement en neurologie, neurorééducation (dystonies, Parkinson, AVC), en psychiatrie (dépression, schizophrénie) mais aussi dans d’autres domaines (acouphènes, douleurs chroniques) .
2.3
Stimulation cérébrale et rééducation neurologique post AVC : état des connaissances dans le cas de l’atteinte motrice du membre supérieur
Notre objectif est de synthétiser et d’illustrer les approches proposées pour la rééducation de la motricité du membre supérieur à l’aide des techniques de neurostimulation, que ce soit en rTMS ou en tDCS.
La revue de la littérature a porté sur les publications de langue anglaise indexées dans PubMed portant sur l’utilisation de l’une de ces techniques à visée d’amélioration fonctionnelle chez des patients présentant une hémiparésie post-vasculaire. Pour la recherche, les termes suivants ont été combinés : « rTMS OU tDCS OU repetitive transcranial magnetic stimulation OU transcranial direct current stimulation OU cerebral stimulation » ET « stroke OU rehabilitation OU hemiparesis ». L’auteur principal a sélectionné, à la lecture des titres et des résumés, les articles originaux ou les revues de la littérature existantes. Les bibliographies des articles sélectionnés ont été étudiées afin de trouver des articles additionnels. Les Tableaux 1–4 recensent les différentes études retenues concernant l’utilisation clinique de l’une ou l’autre des techniques pour améliorer la fonction du membre supérieur en post AVC. Certaines de ces études seront décrites précisément ci-dessous, afin d’illustrer chacune des stratégies qui ont pu être proposées (tDCS ou rTMS, inhibitrice, excitatrice, ou comparaison des deux stratégies, session unique ou sessions multiples).
Auteurs, année | Type d’étude | Paramètres de stimulation | Patients | Délai post AVC | Évaluations | Résultats significatifs |
---|---|---|---|---|---|---|
Khedr et al., 2005 | Essai thérapeutique randomisé contrôlé contre placebo, en double insu | M1 ipsilésionnel, 3 Hz, 10 trains de 10s séparés de 50s, 1/j pendant 10j. Condition placebo : coil à distance du scalp | 52 patients, AVC ischémique | 5–10 jours | Indice de Barthel, Scandinavian Stroke Scale, NIHSS | Amélioration des paramètres cliniques persistant 10 jours |
Kim et al., 2006 | Randomisée, contre placebo, en cross-over, en simple insu | M1 ipsilésionnel, 10 Hz, 80 % du seuil moteur, trains de 2s, 8 trains Stimulation placebo : coil à 90° | 15 patients, AVC épargnant M1 | > 3 mois | Précision et rapidité de frappe sur un clavier numérique ; amplitude des potentiels évoqués moteurs (PEM) | Amélioration de la performance dans le groupe traité, corrélée à l’augmentation d’amplitude des PEM |
Malcolm et al., 2007 | Randomisée, contre placebo, en aveugle | M1 ipsilésionnel, 40 pulses à 20 Hz, 90 % du seuil moteur, toutes les 30s, 50 trains, soit 2000 pulses Placebo : coil factice. Associé à thérapie induite par contrainte | 19 patients | > 1 an | Wolf Motor Function Test, Box and Block Test, Motor Activity Log | Amélioration dans les deux groupes sans différence statistique, mais groupes hétérogènes initialement Amélioration BBT meilleure dans groupe rTMS |
Yozbatiran et al., 2009 | Étude pilote de sécurité | M1 ipsilésionnel, 20 Hz pendant 20 minutes, session unique | 12 patients déficit modéré à sévère | 4,7 ans en moyenne | Force de préhension, Purdue Pegboard Test | Amélioration modeste de la force de préhension, de la dextérité, persistant après une semaine |
Ameli et al., 2009 | Contrôlée contre placebo | M1 ipsilésionnel, 10 Hz, 80 % du seuil moteur, 5s toutes les 30s (1000 pulses) Placebo : idem, sur le vertex | 29 patients | 1–88 semaines | Analyse du mouvement de frappe de l’index et de la main Analyse IRMf | Patients répondeurs à la rTMS (AVC sous-corticaux) : normalisation du pattern IRMf Amélioration corrélée à l’activité initiale de M1 côté lésé |
Koganemaru et al., 2010 | Randomisée, contrôlée, en cross-over | 3 conditions Eex : exercices d’extension des doigts et poignet TMS : rTMS 5 Hz, représentation corticale des extenseurs des doigts, 100 % seuil moteur ; 1 train de 8s toutes les minutes, 15 min Eex-TMS : association des deux interventions | 9 patients, AVC sous-cortical 9 sujets sains témoin | > 5 mois | Exp1 : amplitude d’extension active, spasticité Exp 2 (sujets sains) : paramètres d’excitabilité corticale Exp3 (patients) : 2 sessions/semaine, 6 semaines ; spasticité et extension active | Exp 1 : extension active, spasticité et force de préhension améliorées dans la condition Eex-TMS Exp2 : excitabilité non modifiée en condition Eex, augmentation diffuse en condition TMS, augmentation pour les extenseurs en condition Eex-TMS Exp3 : amélioration de l’extension active (10–15°), de la flexion active (5–10°), de la spasticité, de la force de préhension |
Khedr et al., 2010 | Randomisée, contrôlée contre placebo, en aveugle | M1 ipsilésionnel, 3 ou 10 Hz, ou stimulation placebo 1/j 5 jours consécutifs | 48 patients | < 1 semaine | Excitabilité corticale Échelle d’incapacité motrice, suivi pendant 1 an | Amélioration meilleure dans groupes stimulés, corrélée aux modifications de l’excitabilité corticale |
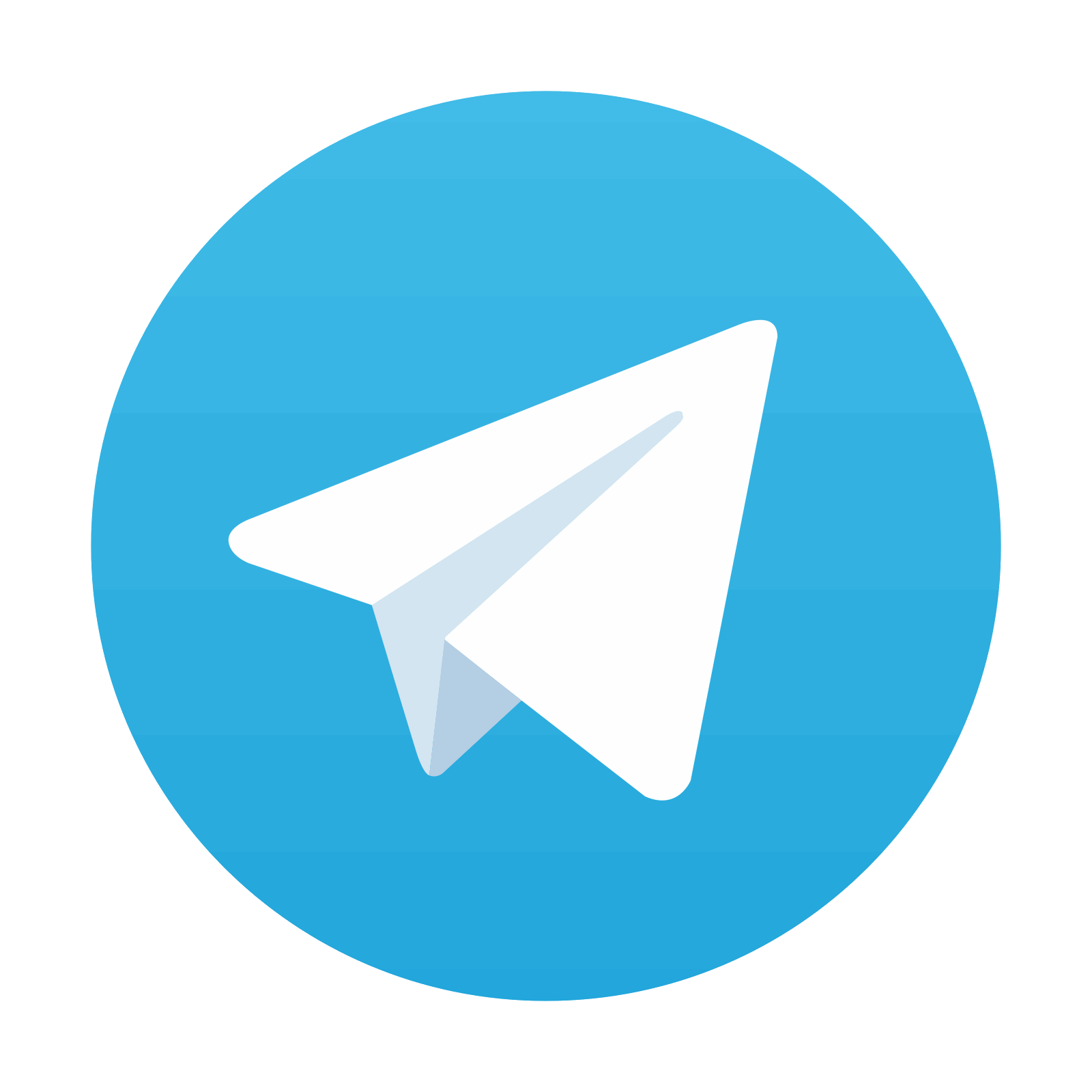
Stay updated, free articles. Join our Telegram channel

Full access? Get Clinical Tree
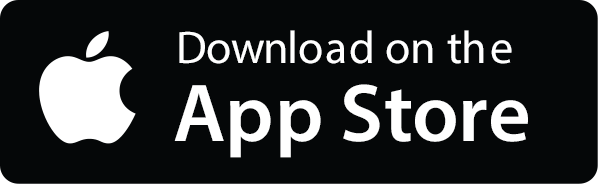
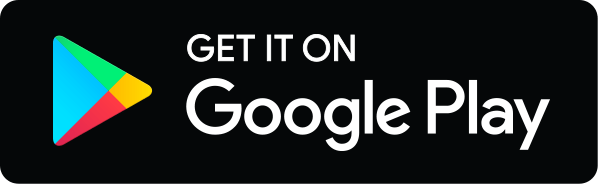
