Neutrophils are myeloid-lineage cells characterized by the presence of granules containing enzymes and other potentially toxic agents involved in host defense.
Neutrophils are short-lived, terminally differentiated cells that exist primarily in the bloodstream, where they participate in host surveillance of foreign organisms.
Neutrophils function in acute inflammation and provide an essential defense against acute bacterial infections; abnormalities of neutrophil function are uncommon and impair ability to respond to life-threatening infections.
Key functions of neutrophils include phagocytosis and degradation of foreign particles, through activation of proteases and other antibiotic molecules, as well as generation of toxic oxygen radicals.
Neutrophil extra-cellular traps (NETs) are extruded neutrophil chromatin and granule enzymes that can trap and destroy bacteria, but also promote and resolve inflammation and may propagate autoimmunity.
Neutrophils play roles in many rheumatic conditions, both as effectors and as contributors to disease processes.
Overview
Neutrophils (polymorphonuclear neutrophils, PMNs) belong to the family of polymorphonuclear leukocytes, hematopoietically derived cells that share the features of a multilobed nucleus and highly developed intracytoplasmic granules (hence the alternative term granulocytes for this family of cells). On the basis of the cytochemical staining properties of their respective granules, three classes of polymorphonuclear leukocytes can be identified: neutrophils, eosinophils, and basophils. Whereas neutrophil granules stain with neutral dyes, eosinophil granules are most effectively stained with acidic dyes such as eosin, and basophil granules stain with basic dyes. In a standard polychromatic Wright stain of a peripheral blood smear, the cytoplasm of neutrophils, eosinophils, and basophils appears blue-pink, pink, and blue, respectively. These classes of polymorphonuclear leukocytes differ with respect to not only appearance, but also biochemistry and function. Polymorphonuclear leukocytes constitute an important part of the organism’s system of innate immunity: their responses to foreign organisms, antigens, or both are preprogrammed and independent of prior exposure to the foreign particle.
Neutrophils are the body’s first line of defense against foreign invaders and constitute a major cell type involved in acute and some forms of chronic inflammation. The importance of neutrophils in bacterial defense is illustrated by patients who have hereditary defects in neutrophil function and are prone to repeated and often life-threatening infections (discussed later in this chapter). Neutrophils are the most prevalent leukocytes in the bloodstream, typically constituting greater than 50% of all bloodstream leukocytes. During bacterial infection, the percentage of neutrophils may increase to 80% or more. In contrast, tissue concentrations of neutrophils during periods of homeostasis are thought to be low, but increase in response to infection or other triggers. Neutrophils may be considered to be surveillance cells, sweeping through the bloodstream, scanning for tissue infections or other inflammatory events. Unfortunately, the capacity of neutrophils to destroy foreign organisms is matched in some circumstances by a capacity for host tissue destruction. In this chapter, we review neutrophil development, structure, and function; the role of neutrophils in protecting against infection; and the role and impact of neutrophils in the pathogenesis and pathophysiology of immune deficiency and autoimmune and autoinflammatory diseases.
Neutrophil Development, Morphology, and Content
Neutrophil Myelopoiesis and Clearance
The neutrophil majority in the bloodstream is duplicated in the bone marrow, where 60% of hematopoietic capacity may be dedicated to neutrophil production. Daily, 10 11 neutrophils are released into the bloodstream. Neutrophil development in the marrow takes about 14 days, originating with the hematopoietic stem cell. Stem cells fated to become neutrophils first differentiate into myeloblasts, which retain the capacity to develop into eosinophils, basophils, and neutrophils. Subsequent differentiation leads to the neutrophilic promyelocyte, a dedicated precursor of the neutrophil, and proceeds through the stages of neutrophilic myelocyte, metamyelocyte, band cell, and mature neutrophil. At the metamyelocyte stage, neutrophil mitosis ceases, whereas neutrophil development and organization of granules continue. Only the mature neutrophil demonstrates the classic feature of a multilobed nucleus. Neutrophils are terminally differentiated; they neither divide nor, as a general rule, alter their gross phenotype after their release from the marrow. However, in the setting of certain infections, such as helminths, some neutrophils may show a distinct profile of gene expression and a ring-form nucleus, in contrast to the typical multilobed cell seen in the peripheral blood.
Given the origin of neutrophils from pluripotent stem cells, as well as the precise phases of their development, the mechanisms regulating neutrophil differentiation are of considerable interest. Although this process remains incompletely understood, studies have emphasized the role of a particular complement of transcription factors and cytokines that seem to direct the early cells toward neutrophil development. Several myeloid factors are necessary for the transcriptional regulation of neutrophils including LEF-1, CCAAT enhancer binding proteins α, β, and ε (C/EBP-α, -β and -ε), and GFI-1. In contrast to other myeloid cells, lack of expression of the transcription factor GATA-1 also contributes to committed development of neutrophils. , Principal among the cytokines regulating granulopoiesis is granulocyte colony-stimulating factor (G-CSF). G-CSF effects include induction of myeloid differentiation, proliferation of granulocyte precursors, and release of mature neutrophils from the marrow. Biologic effects of G-CSF are mediated through its receptor (G-CSFR or CD114), a member of the class I cytokine receptor family. Although other hematopoietic cytokines contribute to granulopoiesis in vivo (including granulocyte-macrophage colony-stimulating factor [GM-CSF], IL-6, and IL-3), their individual presence is not essential, as demonstrated by murine knockout experiments.
Once mature, neutrophils exit the bone marrow through the sinusoidal endothelium and enter the circulation, a process called transcellular migration. Neutrophils released from the marrow have a bloodstream half-life of approximately 6 hours and a tissue half-life only marginally longer. Neutrophil life spans may be modulated by soluble signals; when exposed to stimuli such as TNF and Fas (CD95) ligand, neutrophils undergo apoptosis or programmed cell death. , The high output and short half-life of neutrophils imply that neutrophil clearance mechanisms must exist. Recently, the SDF-1/CXC chemokine receptor 4 (CXCR4) signaling system has been implicated in neutrophil clearance. CXCR4, a G-protein coupled receptor, is expressed at low levels in the mature neutrophil. As they age, neutrophils alter their phenotype and upregulate CXCR4. This change supports homing to the bone marrow via the chemoattractant stromal-derived factor 1 (SDF-1 or CXCL12). Once back in the marrow, senescent neutrophils are phagocytosed by stromal macrophages. Senescent or apoptotic bloodstream neutrophils are also cleared by liver and spleen macrophages (reticuloendothelial system). Although little is known about the molecular mechanisms underlying neutrophil clearance in the liver and spleen, upregulation of the adhesion molecule P-selectin on Kupffer cells appears to be relevant. It remains a matter of speculation whether tissue neutrophils are cleared primarily via local macrophages or first passaged back through the lymphatic drainage system.
Neutrophil Morphology and Contents
Neutrophil nuclei tend to have more lobes than nuclei of other polymorphs, typically three to five ( Figs. 11.1 and 11.2 ). In some circumstances, including vitamin B 12 deficiency, neutrophil nuclei may become hypersegmented, with as many as seven lobes. The multilobed nature of the neutrophil nucleus reflects a condensation of chromatin, suggesting that neutrophils might be incapable of transcription. It is now appreciated, however, that neutrophils retain the capacity for both constitutive and stimulated protein synthesis, albeit at a limited rate.


Neutrophil granules identifiable by classic histochemical staining comprise two classes ( Figs. 11.1 and 11.2 ). Two additional classes of granules require special techniques to recognize and identify them.
Primary Granules
Neutrophil primary granules form first (in myeloblasts and promyelocytes) and, by virtue of their staining tendencies (affinity for the basic dye azure A), are also referred to as azurophilic granules. These granules are oval or round and vary in size. They are similar, and functionally equivalent, to the lysosomes of other cells. Characteristic of azurophilic granules is the presence of myeloperoxidase (MPO), an enzyme that catalyzes the formation of hypochlorous acid from chloride in the presence of hydrogen peroxide (H 2 O 2 ) (see Respiratory Burst, later). The presence of large amounts of this enzyme in azurophilic granules gives collections of neutrophils (pus) their typical greenish yellow color. Consistent with their functional role as lysosomes, azurophilic granules also contain a variety of proteases and other enzymes including elastase, lysozyme, acid phosphatase, cathepsins, and enzymes directed at nucleic acids and sugars ( Table 11.1 ). At the membrane level, however, they differ from true lysosomes in their lack of lysosome-associated membrane proteins I and II (LAMP-I and LAMP-II) and the mannose-6-phosphate receptor system.
Secretory Vesicles | Gelatinase Granules | Specific Granules | Azurophilic Granules | |
---|---|---|---|---|
Relative size | Smallest | Intermediate | Intermediate | Largest |
Soluble (matrix-associated) components | Plasma proteins | Gelatinase Acetyltransferase Arginase 1 Lysozyme Ficolin 1 | Gelatinase MMP-3 MMP-8 MMP-9 Lactoferrin β 2 -Microglobulin NGAL α 1 -Anti-trypsin Lysozyme Haptoglobin hCAP-18 | Myeloperoxidase Glucuronidase Elastase Lysozyme Proteinase 3 α 1 -Anti-trypsin Defensins Cathepsin G BPI Azuricidin NSP4 |
Membrane-associated components | FMLP receptor SCAMP NRAMP2 VAMP2 CD11b/CD18 Cytochrome b 558 Alkaline phosphatase Uroplasminogen activator CD10, CD13, CD16, CD45 CR1 Decay accelerating factor | FMLP receptor CD11b/CD18 Deacylating enzyme MMP-25 SCAMP CD177 | CD11b/CD18 Cytochrome b 558 CD66, CD67, CD177 Fibronectin receptor TNF receptor | CD63, CD68 |
Secondary Granules
In contrast to primary or azurophilic granules, neutrophil secondary granules constitute a population unique to neutrophils, a fact reflected in the alternatively employed nomenclature of specific granules. Specific granules possess an extensive array of membrane-associated proteins including cytochromes, signaling molecules, and receptors. Specific granules constitute a reservoir of proteins destined for topologically external surfaces of phagocytic vacuoles and the plasma membrane (see Table 11.1 ). , One particularly important family of proteinases found in neutrophil-specific granules are the matrix metalloproteinases (MMPs), including neutrophil collagenase-2 (MMP-8), gelatinase-B (MMP-9), stromelysin (MMP-3), and leukolysin (MMP-25). MMPs are stored as inactive proenzymes and undergo proteolytic activation after specific granule fusion with, and interaction with azurophilic granule contents in, the phagocytic vacuole. , , MMP activation confers upon neutrophils the ability to alter and degrade integral membrane components of phagocytosed bacteria. Neutrophil MMP function is not limited to bacterial killing, however. For example, MMPs are also important for neutrophil extravasation and diapedesis (discussed in this chapter).
Azurophilic and specific granules additionally contain antimicrobial proteins and peptides that are the cornerstone of innate immunity. A detailed description of the neutrophil’s armamentarium against foreign invaders is beyond the scope of this chapter, but a few whose mechanisms of action have been elucidated warrant mention. Elastase, mentioned previously, aids in the killing of gram-negative bacteria via degradation of bacterial outer membrane protein A. Elastase-deficient mice are more susceptible to infection with gram-negative (but not gram-positive) organisms than wild-type mice. The defensins, stored in azurophilic granules, accumulate in mg/mL concentrations in phagocytic vacuoles (discussed later) and render target (e.g., bacterial) cell membranes permeable. Based on their content, primary granules may be further subdivided into defensin-rich and defensin-poor granules, respectively.
Bactericidal/permeability-inducing protein (BPI), also stored in azurophilic granules, acts in concert with the defensins; it potently neutralizes endotoxin and is cytotoxic to gram-negative bacteria. BPI also enhances the activity of secretory phospholipase A 2 , which has activity against gram-negative and gram-positive bacteria. Lactoferrin, found in specific granules, deprives microorganisms of iron and has antiviral and antibacterial effects. Other granule-associated proteins, such as cysteine-rich secretory protein 3 (CRISP3) and ficolin 1, have recently been described, although their functions are still unclear.
Gelatinase Granules and Secretory Vesicles
Special techniques have confirmed the existence of two additional classes of vesicles. Gelatinase granules are identical in size to specific granules and share some common proteins. As their name implies, however, gelatinase granules are distinguished by their high concentrations of gelatinase, a latent enzyme with the capacity for tissue destruction. Secretory vesicles are smaller and lighter than the other classes and do not seem to contain proteolytic enzymes. Rather, secretory vesicles are noteworthy for an extensive complement of membrane-associated proteins, including receptors otherwise identified with the plasma membrane. These and other data suggest that the secretory vesicle is a reservoir of neutrophil plasma membrane and other membrane proteins (see Table 11.1 ).
Neutrophil granule contents play important roles beyond their direct antimicrobial effects, including amplifying or dampening innate and adaptive immune responses. Lactoferrin released during phagocytosis inhibits proliferation of mixed lymphocyte cultures in vitro by decreasing release of IL-2, TNF, and IL-1β. On the other hand, proteinase 3 has been found to augment release of active TNF and IL-1β in monocyte/neutrophil co-cultures by releasing the membrane-bound forms of these cytokines. Similarly, gelatinase B converts latent IL-1β into its active form, and potentiate IL-8 activity by truncating this chemoattractant and increasing its release, consequently amplifying neutrophilic influx. , Neutrophil elastase may also play a pro-inflammatory role by virtue of its ability to cleave and disrupt phosphatidyl serine receptors on macrophages. Apoptotic cells undergo membrane alterations that lead to expression of phosphatidyl serine on their outer membrane surface, and interaction of phosphatidyl serine with its receptor on macrophages leads to macrophage responses that downregulate inflammation through the generation of transforming growth factor (TGF)-β. By disrupting these interactions, neutrophil elastase may permit inflammation to continue.
Neutrophil Activation and Signal Transduction
For bloodstream neutrophils to destroy foreign targets in the periphery, they must first sense the presence of such targets at a distance. They must then attach to the activated endothelium of blood vessels through multiple interactions involving adhesion molecules and their receptors (rolling and adhesion). After passing through the endothelium of postcapillary venules (diapedesis), neutrophils migrate to the source of the signal (chemotaxis). Finally, neutrophils must encounter a target, engulf it, and destroy it. Collectively, the processes that allow neutrophils to respond in these manners are referred to as neutrophil activation. Because of the potential for tissue destruction, neutrophil activation must be carefully regulated. The internal responses through which a cell translates an encounter with a stimulus into a particular phenotypic response are termed signal transduction ( Fig. 11.3 ).

Stimuli and Receptors
Classic neutrophil chemoattractants include lipid mediators (e.g., leukotriene B 4 [LTB 4 ], platelet-activating factor) and proteins/peptides (e.g., formylated peptides, the complement split product C5a, and the chemokine IL-8) In vivo, chemoattractants are formed at sites of inflammation, either produced at the site by inflammatory cells (e.g., LTB 4 or IL-8) or liberated from presynthesized proteins, as in the case of C5a. The ability of formylated peptides such as N -formyl-methionyl-leucyl-phenylalanine to stimulate neutrophils probably represents a particularly ancient arm of the innate immune response because prokaryotic, but not eukaryotic (e.g., human), cells synthesize proteins whose first amino acid is a formylated methionine, allowing the more advanced organisms to recognize the more primitive ones. CXC chemokines are a group of chemoattractants characterized by the presence of two N-terminal cysteines (C) separated by any other amino acid (X) at the carboxy terminus. CXC chemokines that play a role in neutrophil recruitment include IL-8 (CXCL8), KC (CXCL1), and MIP-2 (CXCL2). In addition to their attractant activities, chemoattractants also stimulate most other aspects of neutrophil activation. Their individual potencies for particular responses differ, however, suggesting that they serve overlapping but distinct functions in neutrophil activation. ,
Bloodstream activation of neutrophils depends on the presence of specific surface receptors. Most chemoattractant receptors belong to a class known as seven-transmembrane-domain receptors (also called serpentine seven receptors or G protein-coupled receptors [GPCRs]); these receptors are composed of a single protein chain whose hydrophobic domains snake across the plasma membrane a total of seven times. Binding of chemoattractants to GPCRs occurs in a pocket on the extra-cellular face of the plasma membrane, at or below the level of the lipid hydrophobic head groups. Receptors for soluble ligands other than chemoattractants have also been identified on neutrophils, including receptors for growth factors, colony-stimulating factors, and cytokines. Growth factor receptors are members of the protein tyrosine kinase receptor family, in which ligand interaction with two identical or related receptors brings them into proximity, causing their cross-phosphorylation and activation. Receptors for a variety of inflammatory stimuli become highly expressed only in mature neutrophils. These include CXC and CC chemokine receptors such as IL-8R-α and β; CXCR4 and CCR-1, 2, and 3; receptors for TNF and interferon (IFN)-α and -γ; and interleukin receptors IL1R, IL4R, IL6R, IL10R, and IL17R. Some nonchemoattractant ligands do not directly activate neutrophils but modulate their function. For example, pre-treatment of neutrophils with either insulin or GM-CSF results in amplification of subsequent neutrophil responses to chemoattractants, a process referred to as priming.
Guanosine Triphosphate-Binding Proteins
Ligation of seven-transmembrane-domain receptors results in the activation of a class of intra-cellular effectors known as heterotrimeric guanosine triphosphate (GTP)–binding proteins , or G proteins. G proteins are composed of α, β, and γ subunits, and individual G protein types are distinguished by the particular α, β, and γ subunits they employ. In neutrophils, the predominant G proteins are of the G i family. G protein γ subunits are modified by the addition of prenyl (polyisoprene) and carboxy-terminal methyl groups, which anchor them to the plasma membrane. All G proteins share the capacity, localized to their α subunits, to bind GTP and hydrolyze it to guanosine diphosphate (GDP). G proteins are active when GTP bound, but inactive in the GDP-bound form. Engagement of the appropriate serpentine-seven receptor promotes the binding of GTP on the α subunit. As a consequence of GTP binding, heterotrimeric G proteins dissociate into α and β/γ components, each with specific effector functions.
A monomeric class of low-molecular weight (20 to 25 kDa) GTP-binding proteins (LMW-GBPs) is also important in neutrophil signal transduction. Because the first LMW-GBP described was the proto-oncogene Ras, these also are referred to as Ras-related or Ras superfamily proteins, or simply small GTPases. Small GTPases combine, in one molecule, the prenyl and methyl modifications of the G protein γ subunit with the GTP-binding capacity of the α subunit. At least four families of small GTPases have been described: the Ras family, whose members play roles in activation as well as cell growth and division; the Rho family, which functions in cytoskeletal rearrangements; and the Rab and Arf families, crucial for vesicular and endomembrane trafficking. All four classes of small GTPases are represented in neutrophils. The Rho family is thought to play the most direct role in neutrophil chemotaxis, with the Rho-family proteins Rac and Cdc42 regulating cytoskeletal rearrangements at the leading edge of neutrophil migration, and RhoA itself regulating arrangements at the uropod or trailing end. (See also the section on chemotaxis.)
Second Messengers
Second messengers are small, diffusible molecules that are generated in response to stimuli and transmit signals from membrane receptors to downstream effector proteins. In the classic model of neutrophil activation, engagement of receptors results in the activation of phospholipase C, which cleaves phosphatidylinositol triphosphate (PIP 3 ) into diacylglycerol (DAG) and inositol 1,4,5-triphosphate (IP 3 ). DAG and IP 3 mediate the influx of cytosolic calcium and the activation of PKC, respectively. Other phospholipases present in the neutrophil include cytosolic phospholipase A 2 (cPLA 2 ), which cleaves phosphatidylcholine and/or ethanolamine from nuclear membrane lipids to generate arachidonic acid (AA), and phospholipase D, which cleaves phosphatidylcholine into phosphatidic acid and choline. Although many second messengers are implicated in neutrophil activation, others may have inhibitory effects. For example, sphingosine and ceramide inhibit neutrophil phagocytosis.
In addition to lipids, other organic and inorganic second messenger molecules have been characterized. Intra-cellular concentrations of cyclic adenosine monophosphate (cAMP), a classic second messenger, increase rapidly in neutrophils exposed to both stimuli and inhibitors. cAMP in these settings is likely to provide a negative regulatory (off) signal because direct exposure to cAMP inhibits most neutrophil responses, primarily through the activation of protein kinase A (PKA). (The anti-inflammatory effects of the phosphodiesterase 4 inhibitor apremilast are based on its ability to maintain cAMP levels and therefore sustain PKA activation. ) In contrast, increases in cyclic guanosine monophosphate (cGMP) have a modest enhancing effect on some neutrophil responses. Nitric oxide (NO), an important molecule in the regulation of host defense, is produced in neutrophils at low levels. Studies have documented the capacity of NO to exert a variety of second messenger effects, including inhibition of reduced nicotinamide adenine dinucleotide phosphate (NADPH) oxidase, actin polymerization, and chemotaxis (discussed later). Conversely, excessive NO production has been implicated in many rheumatic diseases.
Kinases and Kinase Cascades
Multiple kinases, proteins capable of enzymatically adding phosphate groups to target molecules, contribute to signaling in myeloid and nonmyeloid cells. PKC, now understood to be a family of kinases, was among the first kinases implicated in neutrophil activation in response to chemoattractants. The ability of phorbol myristate acetate (PMA), a synthetic activator of PKC, to stimulate neutrophil responses supports a role for PKC in neutrophil activation. Conversely, inhibitors of PKC block stimulation of neutrophil functions.
The mitogen-activated protein kinases (MAPKs) are a family of serine threonine kinases, including the extra-cellular regulating kinase (ERK), p38, and c-Jun amino terminal kinase (JNK) families. In neutrophils, chemoattractants and other stimuli are capable of activating ERK, p38, and JNK, with time courses consistent with neutrophil activation. A role for ERK activation in signaling for both neutrophil O 2 − and neutrophil adhesion and phagocytosis has been demonstrated. , , Phosphatidylinositol 3-kinase (PI3K) is a family of related enzymes that are found in abundance in neutrophils and primarily catalyze the phosphorylation, not of proteins, but of the three-position of phosphatidylinositol phospholipids. One of the main bioactive products of PI3K is PIP 3 . Chemoattractants such as formyl-methionyl-leucyl-phenylalanine (FMLP) rapidly activate PI3K in neutrophils, where it participates in diverse neutrophil functions including O 2 − generation, adhesion, and degranulation. PI3K also may regulate neutrophil survival and apoptosis.
Neutrophil Function
Adhesion
One of the earliest, crucial aspects of the inflammatory response is the ability of bloodstream neutrophils to adhere to vascular endothelium preparatory to movement into the tissues ( Fig. 11.4 ). Stimulated neutrophils also possess the ability to adhere to each other (homotypic aggregation), which can bring bloodstream neutrophils into proximity with neutrophils already adherent to the vessel, or concentrate them at a site of inflammation. Several families of interacting adhesion molecules are displayed by neutrophils and endothelial cells, including selectins, integrins, intercellular adhesion molecules (ICAMs), and sialylated glycoproteins.

Selectins and Sialylated Glycoproteins
The selectin family includes three related molecules (L-selectin on leukocytes, E-selectin on endothelial cells, and P-selectin on activated platelets and endothelial cells). Selectins share a common structure of two or more complement regulatory domains, an epidermal growth factor–like domain and a lectin domain. Each selectin binds to a specific sialylated glycoprotein on the surface of its interacting cell: E-selectin binds to the sialyl Lewis x antigen on neutrophils, P-selectin binds P-selectin glycoprotein-1 (PSGL-1) on neutrophils, and L-selectin binds PSGL-1 and GlyCAM-1 on the endothelium. Selectin/sialylated glycoprotein interactions are of low affinity and transient, and leukocytes therefore repetitively bind to, and release from, the endothelial surface. The result is a pool of bloodstream neutrophils that is, at any given time, loosely adherent to the vascular surface (i.e., marginated) and moving along it slowly in a rolling, tumbleweed-like motion. These neutrophils are therefore not sampled during routine clinical blood measurements. Exposure of neutrophils and endothelium to appropriate stimuli (e.g., adrenergic discharge, corticosteroids) leads to shedding of selectins and neutrophil release (demargination), with apparent increases in the measured peripheral neutrophil count.
Integrins and Intercellular Adhesion Molecules
The integrins are a large family of heterodimeric molecules generated by various combinations of α and β chains. Like the selectins, integrins require divalent cations (Ca 2+ or Mg 2+ or both) to engage their ligands. Neutrophils express three β 2 -type integrins, each constructed from a distinct α subcomponent (CD11a, CD11b, or CD11c) and a common β 2 chain (CD18). Integrins primarily use ICAMs as their counter-ligands. CD11b/CD18 (also called Mac-1 or CR3 ) binds to fibrinogen, factor X, heparin, and the complement component iC3b in addition to ICAMs and is most strongly implicated in neutrophil/endothelial and neutrophil/neutrophil interactions. In contrast to the selectins, neutrophil CD11b/CD18 is constitutively expressed but inactive; stimulation of neutrophils results in changes in the CD11b/CD18 activation state and increases its affinity for ICAMs and other ligands. Stimulation of endothelium with cytokines such as IL-1β results in increased expression of ICAM-1 and ICAM-2, providing a coordinate mechanism for the regulation of adhesion. In contrast to selectin-mediated adhesion, integrin/ICAM interactions are high-affinity and persistent. Stimulation of rolling neutrophils therefore results in their tight adhesion to vessel walls and constitutes the first committed step in the movement of neutrophils into tissues. Additionally, engagement of integrins by their counter-ligands sends signals into the cell (“outside in” signaling) that regulate selective cell responses such as cytoskeletal reorganization, oxidant production, and degranulation. Outside-in signaling through CD11b/CD18 also coordinates with signaling through Fc receptor FcγRIII (discussed later) to regulate a number of different functions, including phagocytosis of particles opsonized by IgG and the complement component iC3b and neutrophil-dependent adhesion in immune complex-mediated vascular inflammation. Cross-talk between neutrophils and endothelial cells is a CD11b/CD18-dependent event: cross-linking of CD18 on neutrophils leads to increased endothelial permeability, probably through the release of neutrophil proteases.
Data also strongly support that interactions between ICAMs and CD11a/CD18 (also known as lymphocyte function-associated antigen-1 , or LFA-1 ) are necessary for neutrophil adhesion and emigration. During neutrophil rolling on inflamed endothelium, neutrophil engagement by PSGL-1 leads to CD11a/CD18 activation, which in turn may contribute to neutrophil arrest. Evidence suggests that CD11a/CD18 and CD11b/CD18 play slightly different roles, with CD11a/CD18 primarily regulating initial tight adhesion, and CD11b/CD18-ICAM mediating interactions allowing the cell to crawl over the endothelium to identify a possible location for transmigration (see below). The function of CD11c/CD18 on neutrophils remains less clear.
Diapedesis and Chemotaxis
Diapedesis
The mechanism by which neutrophils pass through blood vessels (diapedesis) is not fully established. Although many experts consider that neutrophil diapedesis occurs between endothelial cells by disrupting cell-cell junctions, some evidence suggests that neutrophils pass directly through pores generated within the endothelial cells themselves ( Fig. 11.5 ). , Diapedesis requires homotypic interactions between adhesion molecules found on both neutrophils and endothelial cells which are known as platelet-endothelial cell adhesion molecules (PECAMs). PECAMs are concentrated at endothelial cell junctions, and antibodies that bind PECAMs inhibit transmigration in vitro by limiting neutrophils to the apical surface of the endothelium. Transmigrating neutrophils undergo upregulation of α6β1, an integrin that mediates binding to laminin (a key component of the perivascular basement membrane). Antibodies to α6β1 generally block neutrophil transmigration but fail to do so in a PECAM knockout mouse, implicating α6β1 and PECAM as both crucial to the passage of neutrophils out of the vasculature. CD47, otherwise known as integrin-associated protein, and CD99, expressed on neutrophils and endothelial junctions, have also been implicated in neutrophil passage through the endothelium.

Once beyond the endothelium, neutrophils typically pause before traversing the basement membrane (basal lamina). Classic studies by Huber and Weiss suggest that neutrophils pass through the basement membrane via transient disruption of its patency, without utilization of known proteases or oxygen radicals. The disruptions are rapidly reversed, through mechanisms likely involving endothelial tethering and release of the basement membrane. Speaking metaphorically, the endothelial cells may hold open the door of the tent (basement membrane) as the neutrophil enters, and then release it again after the neutrophil has passed through.
Chemotaxis
Chemotaxis in the direction of a molecular gradient is achieved by the extension of membrane ruffles (lamellipodia), followed by anchorage of the ruffles to the substrate and withdrawal of the trailing edge of the cell in the direction of movement. These changes are accomplished primarily through rearrangement of the actin cytoskeleton after sensing chemotactic gradients that signal through GPCRs and PI3K. Actin is a 41 kDa protein that exists as a soluble, globular monomeric form (G-actin) and as an insoluble linear polymer (F-actin). F-actin may be assembled (extended) at one end (barbed end) and disassembled at the other, under the control of regulatory molecules. During chemotaxis, F-actin formation and extension is concentrated at the leading edge of the neutrophil, permitting extension of the cell membrane (see Fig. 11.5 ). Chemoattractant receptors also concentrate at the leading edge, defining the cell’s directional response to the gradient (headlight phenomenon). As the neutrophil moves along, receptors that were formerly at the leading edge are swept to the tail and internalized.
Phagocytosis and Degranulation
Phagocytosis
Neutrophil phagocytosis of an encountered bacterium or other particle requires direct contact. Phagocytosis can be activated by direct neutrophil recognition of pathogen-associated molecular patterns (PAMPs), which are small molecule motifs found on or in bacteria or viruses (but not typically mammalian cells). PAMPs are recognized by Toll-like receptors (TLRs) and other pattern recognition receptors (PRRs). Human neutrophils express all TLRs except for TLR3, and TLR activation stimulates human neutrophil phagocytosis by promoting structural and conformational changes. However, neutrophils are generally poor at phagocytosing unmodified targets, particularly encapsulated bacteria. Phagocytosis is greatly enhanced by opsonization (from the Greek, “to prepare for the table”), the modification of a target via its decoration with immunoglobulin, complement components, or both ( Fig. 11.6 ).

Neutrophils express two families of receptors for the Fc portion of complexed or aggregated IgG: low-affinity FcγRIIa and high-affinity FcγRIIIb. During some infections, or after in vitro stimulation with interferon or G-CSF, neutrophils also express the high-affinity receptor FcγRI, which binds monomeric IgG.
FcγRIIa binds subclasses of IgG with varying efficiency depending on a polymorphism at amino acid position 131. FcγRIIIb polymorphisms of neutrophil antigens NA1 and NA2 also determine binding to IgG subclasses. Individuals homozygous for the NA2 allele have a lower capacity to mediate phagocytosis than individuals homozygous for the NA1 allele. These differences have important implications for rheumatic diseases in which immune complexes play an important role.
Phagocytosis is an active process involving simultaneous extension of the neutrophil membrane (filopodia and lamellipodia formation) around and invagination of the neutrophil at the locus of the target. Engagement of FcγR and complement receptors results in the activation of different signaling pathways that play distinct roles in phagocytosis. Whereas engagement of CR3 (CD11b/CD18) results in actin stress fiber formation and invagination, engagement of FcγRII results primarily in extension of membranes out from, and around, the target. Signaling by these receptors depends on the activation of distinct members of the Rho family of LMW-GBPs.
Degranulation
Upon engaging a target, neutrophils degranulate, a term reflecting two possible and distinct events. Vesicles can fuse with the plasma membrane, spilling their contents into the extra-cellular space (see Fig. 11.1 ), or they can fuse with the phagocytic vacuole to form a phagolysosome. The former type of degranulation is regulated differentially from the latter and favors mobilization of lighter granules in response to stimuli (secretory vesicles > gelatinase granules > specific granules > azurophilic granules). In the latter type of degranulation (phagolysosome formation), the preferential fusion of azurophilic granules with the phagocytic vacuole results in the delivery of proteolytic proenzymes, MPO, and antibacterial proteins to the site of an ingested bacterium. Fusion of specific granules with the phagocytic vacuole then permits the delivery of collagenase, activation of azurophilic granule enzymes, and the appropriate localization of cytochrome b 558 , a requisite for NADPH oxidase (discussed later). These regulated processes permit the maintenance of potentially toxic agents in inactive forms until they are required, at which point they are brought into proximity with each other and activated. Containment of the activated substances within the phagolysosome concentrates them near target bacteria, and minimizes host tissue damage and neutrophil autodestruction. However, control of granule content is imperfect, and toxic molecules may still spill into the extra-cellular milieu, particularly when the target is large (frustrated phagocytosis).
Respiratory Burst
In addition to the proteases and other antibacterial proteins contained in their granules, neutrophils have the capacity to kill bacteria through the generation of toxic oxygen metabolites such as NO, superoxide anion (O 2 − ), and hydrogen peroxide (H 2 O 2 ). This process, mediated by the NADPH oxidase system and frequently referred to as the oxidative or respiratory burst, is extremely potent and requires tight regulation to prevent neutrophil autodestruction. The central component of NADPH oxidase is flavocytochrome b 558 , which is localized to the membranes of specific granules and consists of two subunits: a 22-kDa component (gp22 phox , for ph agocyte ox idase) and a 91-kDa component (gp91 phox ). This flavocytochrome lacks independent activity, however, and several cytosolic proteins are additionally required for oxidase activation, primarily a 47-kDa and a 67-kDa component (p47 phox and p67 phox ). On neutrophil stimulation, the p47 phox and p67 phox components translocate to the membranes to form an active complex with the flavocytochrome ( Fig. 11.7 ). A fifth protein, p40 phox , associates with p47 phox /p67 phox and plays a less well-defined role in phagocytosis-induced superoxide production. , Finally, the small GTPase p21 rac also translocates to the complex in response to stimuli, and appears to contribute to oxidase activity in some way. ,

When assembled and activated, the NADPH oxidase transfers electrons from NADPH to generate O 2 − :
2O2+NADPH→NADPHOxidaseO2−+NADP++H+
A subsequent, spontaneous dismutase reaction rapidly produces hydrogen peroxide:
2O2−+H2→H2O2+O2
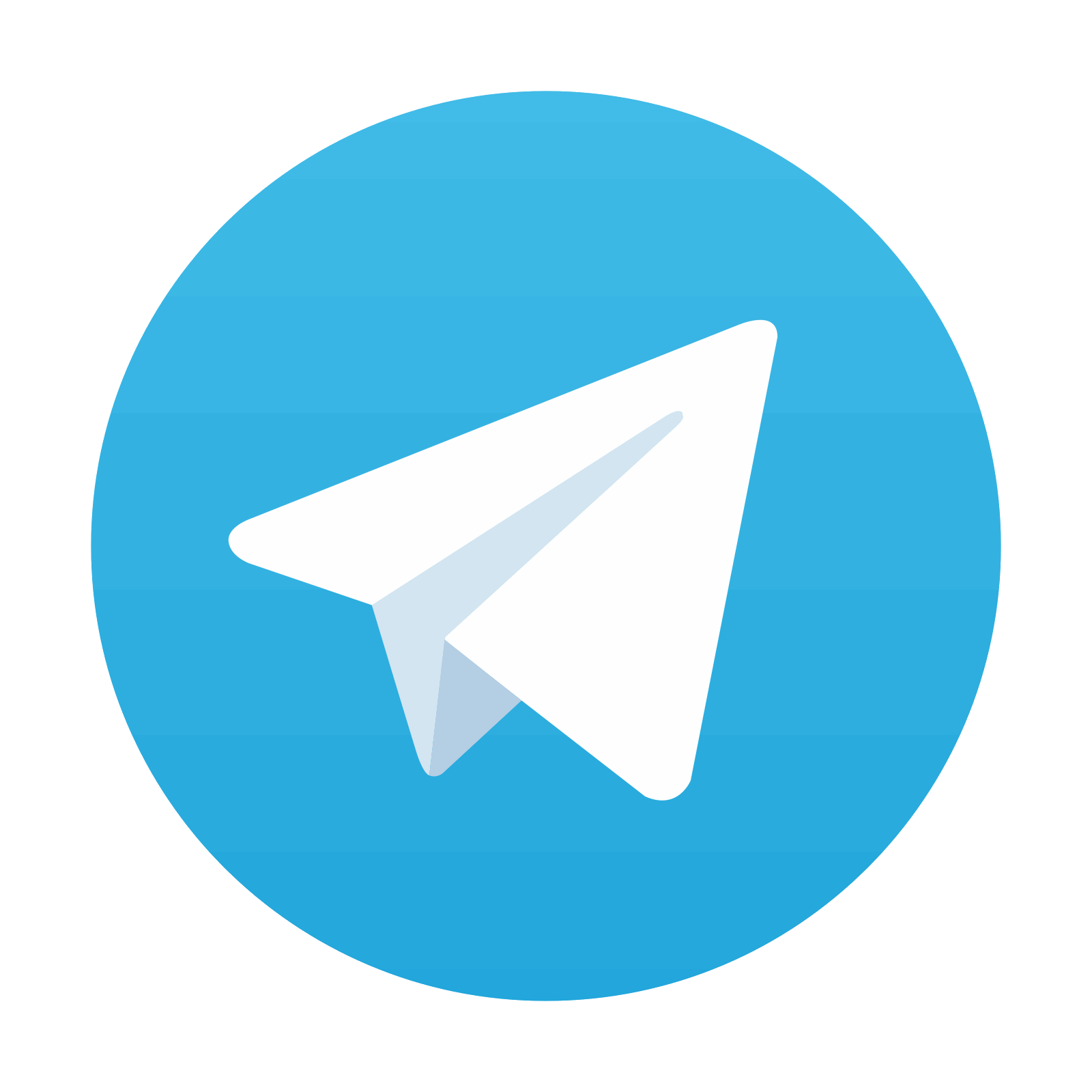
Stay updated, free articles. Join our Telegram channel

Full access? Get Clinical Tree
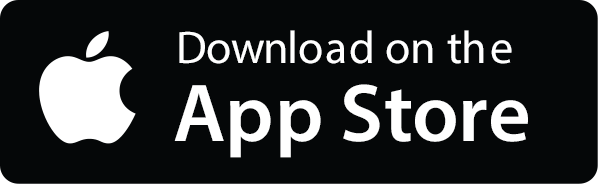
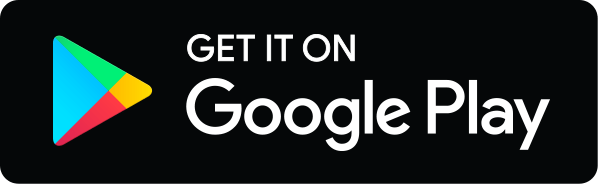
