Eating and drinking are basic pleasures in life that most of us take for granted, yet the ease with which we perform these tasks belies their complex neurologic system of control. Recent studies of human swallowing have begun to unravel some of these complexities, evolving our understanding and thus ultimately helping to generate novel therapies for the treatment of swallowing problems after cerebral injury, such as stroke. This article provides a general overview of current knowledge of the neural control mechanisms that underlie the coordination of mastication, oral transport, swallowing, and respiration in humans.
Eating and drinking are basic pleasures in life that most of us take for granted, yet the ease with which we perform these tasks belies their complex neurologic system of control. This article provides a general overview of current knowledge of the neural control mechanisms that underlie the coordination of mastication, oral transport, swallowing, and respiration in humans.
Oral feeding
The ability of organisms to maintain adequate energy stores is critical for survival and achieved through feeding. In mammals, this encompasses many factors, including the sight, smell, taste, texture, and temperature of food, all of which involve complex and interrelated neural circuits and responses that have been developed and honed through individual experiences. Some of these homeostatic and hedonistic mechanisms are beyond the remit of this article; feeding is discussed beginning with mastication or chewing.
The masticatory sequence
In humans, the processing of solid ingested material, regardless of size and texture, occurs in a stereotyped manner as categorized by Hiiemae and Palmer . This process is termed the masticatory sequence and is composed of four main components.
- 1.
Stage I transport, during which food is ingested as a bite-sized portion and positioned on the occlusal surface (between the teeth) for further breakdown if necessary.
- 2.
Processing, during which time trituration occurs. The number of processing cycles depends on how tough the ingested material is to chew.
- 3.
Oropharyngeal accumulation time, during which adequately prepared food, ready for bolus formation, is moved distally through the fauces to the oropharynx (stage II transport) while processing of any remaining food continues.
- 4.
Hypopharyngeal transit time, during which the bolus is swallowed.
The masticatory sequence
In humans, the processing of solid ingested material, regardless of size and texture, occurs in a stereotyped manner as categorized by Hiiemae and Palmer . This process is termed the masticatory sequence and is composed of four main components.
- 1.
Stage I transport, during which food is ingested as a bite-sized portion and positioned on the occlusal surface (between the teeth) for further breakdown if necessary.
- 2.
Processing, during which time trituration occurs. The number of processing cycles depends on how tough the ingested material is to chew.
- 3.
Oropharyngeal accumulation time, during which adequately prepared food, ready for bolus formation, is moved distally through the fauces to the oropharynx (stage II transport) while processing of any remaining food continues.
- 4.
Hypopharyngeal transit time, during which the bolus is swallowed.
The neurophysiology of mastication
Mastication itself is an intermittent, rhythmic process during which the masticatory muscles—tongue, cheeks, lips, palate, and jaw muscles (masseters, temporalis, lateral and medial pterygoids)—coordinate to mechanically break down solid food by way of the teeth into smaller fragments that are mixed with saliva to form a cohesive bolus that can be easily swallowed. Mastication is not a prerequisite of ingestion, because liquid boluses do not require this preswallow step; however, it is the first step of solid bolus digestion and is performed to greatly increase the surface area of ingested foods for more efficient breakdown by digestive enzymes. Until recently this motor task was believed to be solely under reflexive control, but it is now known to be voluntarily controlled through the actions of higher brain centers on the central pattern generator in the brainstem . The fundamental rhythmic pattern of mastication (and therefore the jaw muscles) is controlled by the central pattern generator in the brainstem but is supplemented by the motor cortex, which initiates/stops mastication and also provides preprogrammed movement patterns based on expectations and sensory feedback in conjunction with the basal ganglia. Although under voluntary control, little conscious effort is required once mastication is initiated, with chewing largely occurring automatically. A recent study by Palmer and colleagues , however, demonstrated that food processing, transport, and bolus formation can be modified by conscious control. In particular, subjects were able to consciously inhibit stage II transport to the valleculae, thereby indicating a level of cortical control over the brainstem pattern generator. In another recent study by Onozuka and colleagues the authors mapped out regional brain activity seen with functional MRI (fMRI) during gum chewing in healthy volunteers. They demonstrated increased cerebral perfusion bilaterally in the sensorimotor cortex, supplementary motor area, insula, thalamus, and cerebellum, areas also activated during volitional swallowing, implying that there is significant recruitment of cortical regions during chew activity, which overlaps with that seen during the swallowing phase.
The role of sensory feedback in mastication
Peripheral information from receptor systems in the dentures, tongue, cheek, jaw elevator muscles, and temporomandibular joint are also of importance . Although they are not necessary during generation of the basic masticatory rhythm, they monitor the progress of chewing and modify commands sent to the appropriate effector muscles by significantly influencing the pattern generator directly and indirectly by descending motor pathways or through jaw reflexes. Degenerative diseases, lesions, infarction, and hemorrhages involving these areas can have profound effects on mastication and mandibular function . The modulation of mastication for different foods has been demonstrated by several research groups over the years.
Hiiemae and colleagues using videofluorography demonstrated that soft foods, such as banana and chicken paste, are processed much more quickly than hard foods, such as cookies or peanuts. In a separate study , they demonstrated that bite size also varied depending on food type and that food type influenced the parameters of the individual masticatory cycles and the overall duration of processing. Peyron and colleagues observed significant differences between males and females chewing standardized artificial but edible foods. Increases were seen in the number of masticatory cycles and the activity in jaw-closing muscles, including the amplitude and velocity of jaw movements, as food hardness progressively increased. The number of masticatory cycles per sequence was also seen to increase gradually with age.
Although other sensory modalities also influence the masticatory sequence, food hardness is the predominant factor making up one of the three major inputs into the central pattern generator. This peripheral feedback system related to food hardness is provided through sensory afferents in muscle spindles and periodontal mechanoreceptors . Nerve deafferentation studies in animals removing sensory feedback from periodontal receptors demonstrated reduced facilitation of masseter muscle activity, whereas destruction of spindle cell bodies almost completely removed that facilitation, thereby indicating the importance of these peripheral receptor systems in modulating masticatory muscle motoneuron activity.
Integration of the chemical senses
As mentioned earlier, normal feeding depends on the integration of several systems that include the chemical senses of smell (olfactory) and taste (gustatory). Together they give rise to flavor perception and are accounted for by three sensory systems: olfactory ( Fig. 1 ), gustatory ( Fig. 2 ), and trigeminal chemosensory. Olfactory information can influence feeding behavior, social interactions, and reproduction in many animals. The gustatory system provides information about the quality, quantity, pleasantness, and safety of ingested food, whereas the trigeminal chemosensory system provides information about irritating or noxious stimuli.
Smell
The olfactory epithelium (approximately 10 cm 2 in an average 70-kg male) contains sensory receptors that detect and transduce information about the identity, concentration, and quality of a wide range of chemical stimuli termed odorants. This information is projected directly to neurons in the olfactory bulb by way of cranial nerve I (olfactory), which in turn projects to the pyriform cortex (olfactory cortex) in the temporal lobe (see Fig. 1 ) and other brain regions, such as the amygdala, hypothalamus, and insula . The olfactory system is thus unique among the sensory systems in that the thalamus is not involved en route to the primary cortical region processing the sensory information. Projections from the pyriform cortex and other forebrain regions, however, do provide olfactory information to several additional regions of the cerebral cortex (by way of the thalamus), where further processing identifies the odorant and initiates appropriate motor, visceral, and emotional reactions to olfactory stimuli .
Depending on the origin of the odorant, either from outside (orthonasal) or inside (retronasal) the body, different neuronal processing pathways also exist . Orthonasal stimuli refer to odorants that are sniffed from the environment through the nares and are processed by the olfactory cortex and relayed to the primary olfactory cortex in the orbitofrontal cortex. Retronasal stimuli, however, refer to odorants released through the ingestion and breakdown of foods, causing volatile molecules to move backward from the oral cavity onto the olfactory epithelium through the nasopharynx. This olfactory pathway is only activated during nasal exhalation while masticating or swallowing and additionally incorporates taste, texture, and sound during the sensory processing.
Taste
The human taste system (see Fig. 2 ) acts in concert with the olfactory and trigeminal chemoreceptive systems to indicate whether or not food should be ingested. Once in the mouth, the chemical constituents of the food interact with (peripheral) taste receptor cells throughout the oral cavity, in lingual and extralingual locations, providing information about the identity (sweet, sour, salty, bitter, and umami), concentration, and pleasant or unpleasant quality . This information helps to prepare the rest of the gastrointestinal system to receive food by causing salivation and swallowing, or gagging and regurgitation if the substance is noxious. In humans, two thirds of all taste receptor cells are located in the tongue, with the remaining third being distributed throughout the epiglottis, soft palate, larynx, and oropharynx . Information regarding food temperature and texture (including viscosity and fat texture) is also relayed by way of sensory afferents in trigeminal and other sensory cranial nerves. This information is presented to cortical taste areas, such as the insula (primary gustatory cortex) and orbitofrontal cortex (secondary gustatory cortex), by way of the brainstem nucleus tractus solitarius and ventral posterior complex of the thalamus . Projections also connect the nucleus tractus solitarius by way of the pons to the hypothalamus and amygdala, influencing appetite, satiety, and other homeostatic responses associated with eating . These regions, also highlighted in human functional brain imaging studies , have close resemblance to cortical areas activated in human swallowing (see later discussion) and thus imply an important overlap of function.
Swallowing
For most people, swallowing or deglutition is a normal and effortless task, but despite its ease, it is a complex and dynamic sensorimotor activity involving 26 pairs of muscles and five cranial nerves. This complexity emerges as a consequence of the common shared pathway between the respiratory and gastrointestinal tracts and has arisen to avoid the threat of food or liquid entering the airway. Swallowing thus enables the safe delivery of ingested food, as a bolus, from the mouth to the stomach while ensuring protection of the airway. It is an integral component of feeding, learned during gestation, organized at birth , and essential for the continuation of life.
Traditionally, swallowing is divided into three conventional phases under volitional and reflexive control. They are the (1) oral, (2) pharyngeal, and (3) esophageal phases. Briefly, mastication and the oral phase refer to the volitional transfer of ingested material, as a prepared bolus, from the mouth into the oropharynx and are controlled by the discrete areas of the cerebral cortex. The pharyngeal phase is the first semi-reflexive component triggered by activation of cortical and subcortical brain regions, mainly the central pattern generator in the brainstem, which subsequently controls muscles in the oropharynx to deliver the bolus from the oropharynx to the relaxed cricopharyngeal muscle. The third and final phase, the esophageal phase, begins following closure of the upper esophageal sphincter. This later reflexive component serves the primary function of transporting food to the stomach by a sequential peristaltic contraction of muscles initiated in the pharynx and relaxation of the lower esophageal sphincter.
The neurophysiology of swallowing
The central neural control of swallowing is described as being “multidimensional in nature” recruiting at all levels of the nervous system, and hypothesized to be organized in a hierarchical manner as shown in Fig. 3 . The brainstem swallowing center, which contains the central pattern generator, is at the core of the system and represents the first level of control. Rostral to this and representing a second level of swallowing control are the subcortical structures, such as the basal ganglia, hypothalamus, amygdala, and tegmental area of the midbrain. Representing a third level of swallowing control are the suprabulbar cortical swallowing centers.
The importance of sensation in swallowing
Appropriate preparation of food relies on the continuous feedback of sensory information from receptors in the tongue, soft palate, floor of mouth, and tooth pulp, which detect the size and texture of the bolus, thereby determining the chewing action required from the muscles of mastication . The pharyngeal phase of swallowing also relies on sensory input from the posterior oral regions and pharynx to initiate a response . The intensity of pharyngeal muscle activity and overall duration of the pharyngeal phase of swallowing are not constant; they vary in response to sensory information relayed from afferent receptors about the unique characteristics of the bolus . Anaesthetizing these mucosal regions locally has been shown to increase the time to evoke repeated swallows and may disrupt swallowing modulation, but does not eliminate swallowing completely .
Sensory input to the swallowing tract therefore has three primary functions (1) to assist in initiating swallowing, (2) to modify the threshold for a pharyngeal swallow, and (3) to alter the level of muscle recruitment during swallowing . Sensory information is carried by three cranial nerves: the trigeminal (V), glossopharyngeal (IX), and vagus (X) ; however, the most potent stimulus for triggering swallowing is delivered by way of the superior laryngeal nerve, a branch of the vagus nerve.
Innervation of the swallowing musculature
The motor innervation of the different swallowing musculature is provided through five cranial nerves by the release of acetylcholine at neuromuscular junctions. A brief description follows, but for an in-depth review of the deglutitive anatomy and its innervation, the reader is directed to an excellent review by Jonathan Cichero .
- •
Cranial nerve V (trigeminal). Sensory: conveys most sensory modalities (touch, temperature, pressure, and pain), except taste, from the anterior two thirds of the tongue, face, mouth, and mandible. Motor: innervates muscles of mastication and backward bolus propulsion.
- •
Cranial nerve VII (facial). Special sensory: conveys taste from the anterior two thirds of the tongue and soft palate by way of greater petrosal and chorda tympani, which also stimulates saliva secretion. Mixed-motor: supplies muscles of facial expression, particularly the lips, which prevent spillage during the oral phase of swallowing.
- •
Cranial nerve IX (glossopharyngeal). General sensory: mediates all sensation from posterior one third of tongue, oropharyngeal mucosal membranes, palatine tonsils, and faucial pillars. According to Zemlin, a lesion affecting this nerve can impair the gag reflex unilaterally . Special sensory: conveys taste from posterior one third of tongue. Motor: in conjunction with the vagus nerve innervates the stylopharyngeus, which elevates and pulls anterior the larynx to aid cricopharyngeal relaxation. Secretomotor: stimulates saliva secretion from parotid gland.
- •
Cranial nerve X (vagus). Motor: responsible for raising the velum as it innervates the glossopalatine and the levator veli palatine muscles. Together with cranial nerve IX, pharyngeal branch innervates the pharyngeal constrictors and with cranial nerve XI (cranial root of the accessory nerve) innervates intrinsic laryngeal musculature. It is also responsible for vocal fold adduction during swallowing and cricopharyngeal relaxation. Muscles involved in the esophageal stage of swallowing and those that control respiration are also innervated by the vagus. Sensory: superior and recurrent laryngeal nerves carry information from the velum and the posterior and inferior portions of pharynx, and mediate sensation in the larynx. The superior laryngeal nerve is also important for swallowing and has been shown to potentiate the swallow response when combined with cortical stimulation . At the thyroid cartilage, it divides into two branches: the internal, which supplies the mucous membrane of the larynx above the vocal cords, and the external, which supplies the inferior pharyngeal constrictor and the cricothyroid muscles.
- •
Cranial nerve XII (hypoglossal). Motor: innervates all intrinsic and extrinsic tongue muscles (except palatoglossus innervated by cranial nerve XI).
Brainstem control of swallowing
The brainstem swallowing center is located in the upper medullary and pontine areas of the brain and is bilaterally distributed within the reticular formation. This network of neurons is made up of three functional components: an afferent component, an efferent component, and a complex organizing system of interneurons known as the central pattern generator. Although the cortex is recognized to be responsible for the initiation of swallowing , the central pattern generator organizes the sequential excitation of motor neurons controlling the swallowing muscles . These interneurons are believed to be organized in a temporal manner and have been referred to as early, late, and very late neurons corresponding with their counterparts in swallowing: the oral cavity, pharynx, and striated esophagus . They are also separated into dorsal and ventral groups. The dorsal neurons lie within the nucleus of the tractus solitarius and the adjacent reticular formation, whereas the ventral neurons lie within the reticular formation and adjacent to the nucleus ambiguus. Dorsal neurons integrate both descending inputs from the cerebral cortex and afferent inputs arriving from cranial nerves V, IX, and X, of which the superior laryngeal branch, the superior laryngeal nerve, is the most important. Stimulation of any of these cranial nerves can initiate or modulate a swallow ; however, the most potent trigger for stimulating a swallow is carried by the superior laryngeal nerve. This trigger is matched only by direct stimulation of the nucleus of the tractus solitarius, suggesting that the solitary system is the major contributor of swallowing afferent input .
The afferent fibers also ascend by way of a pontine relay to the level of the cortex. The dorsal neurons activate the ventral neurons, which in turn activate the motor nuclei of cranial nerves V, VII, and X , thus triggering the swallowing motor sequence. Concurrent afferent excitation can facilitate swallowing in an intensity- and frequency-dependent manner . Anesthesia of the areas innervated by these afferents disrupts, but does not completely abolish, the ability to swallow, therefore suggesting that sensation from the swallowing tract can have a modulatory effect on swallowing circuitry .
Evidence from animal studies suggests a significant role for subcortical structures in the control and modulation of swallowing . These structures in the hindbrain (cerebellum), midbrain (substantia nigra and ventral tegmentum), and basal forebrain (hypothalamus, amygdala, and basal ganglia) have been shown to either induce swallowing or modulate the swallowing sequence after initial triggering by the cortex or superior laryngeal nerve. In humans, supportive evidence from clinical observations in patients who have neurologic disorders or lesions of the basal ganglia, such as in Parkinson’s Disease, show marked difficulties in the coordination of oropharyngeal swallowing with changes in the neurology of the subcortical structures, which can disrupt the normal feeding process and lead to dysphagia.
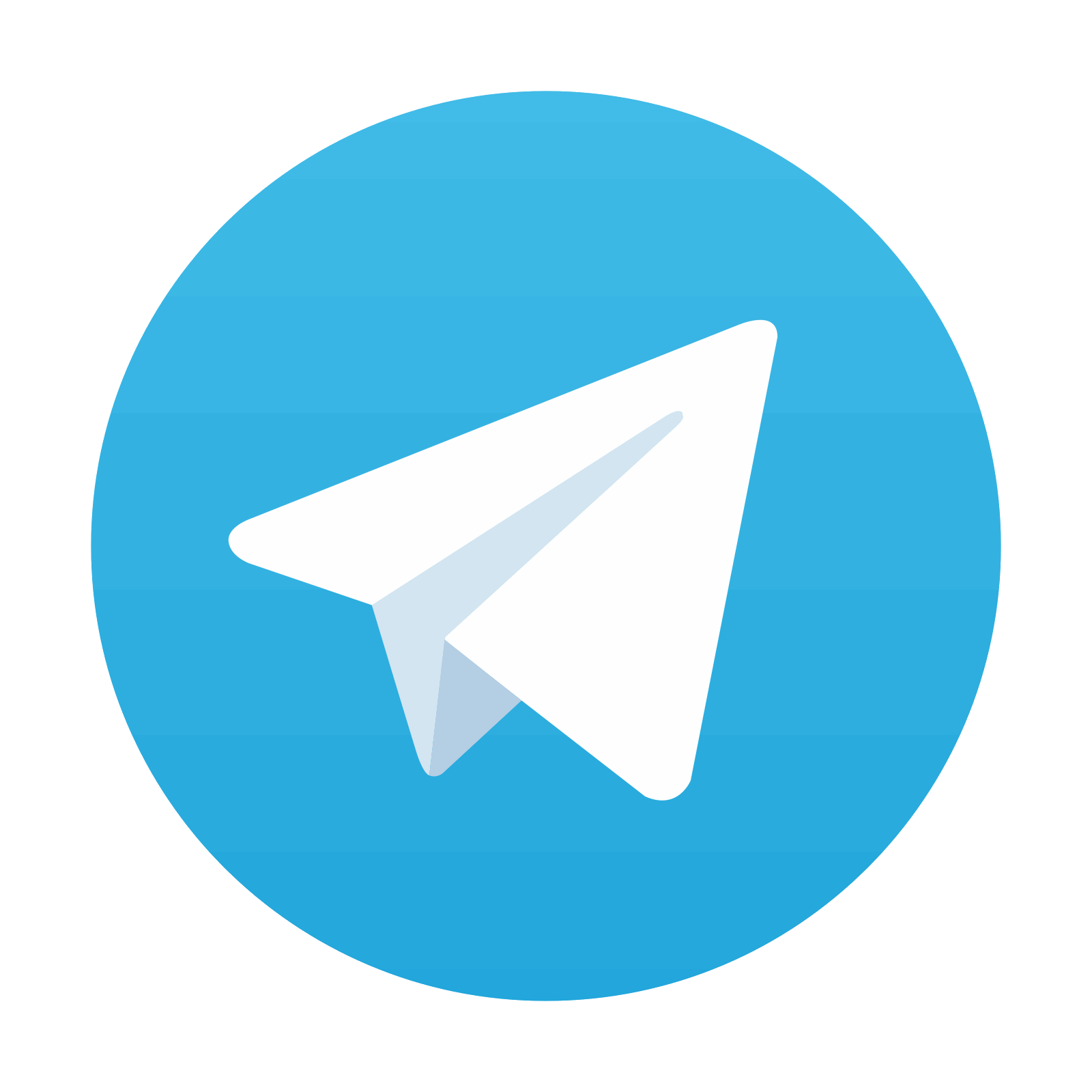
Stay updated, free articles. Join our Telegram channel

Full access? Get Clinical Tree
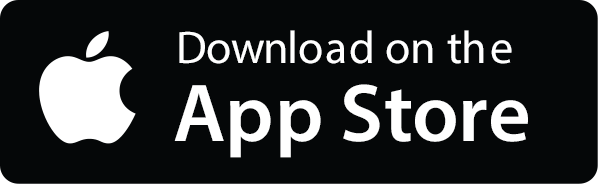
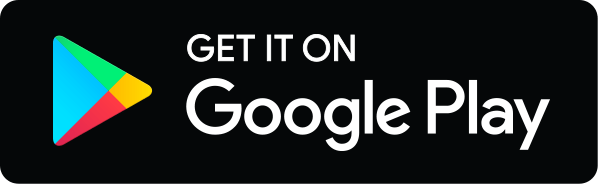