Abstract
The objective measurement of knee motion during functional activities with 3D motion analysis has provided great insights into the restoration of knee biomechanics after ACL reconstructions and any remaining deficits. Several studies have documented that ACL reconstruction reduces but does not restore excessive internal knee rotation. This finding may provide a biomechanical rationale for the increased rate of knee osteoarthritis among athletes who had an ACL tear. More recent, anatomic ACL reconstruction techniques may ameliorate this problem and lead to better long-term outcomes.
Keywords
biomechanics, kinetics, kinematics, knee rotation
Keywords
biomechanics, kinetics, kinematics, knee rotation
Introduction
Anterior cruciate ligament (ACL) rupture is a serious injury of the knee joint that results in instability. The stability of the knee thought to have an ACL injury is traditionally evaluated with an arthrometer (i.e., KT-1000) while the patient is relaxed in a supine position. The arthrometer provides the clinician with a quantitative measure of the amount of passive anterior translation between the femur and the tibia. A minimal amount of joint laxity (within 3 mm of the contralateral knee with intact ACL) during the test is clinically and functionally acceptable. However, such an evaluation is a measure of passive joint stability and does not necessarily reflect the joint’s stability during daily physical activities. Dynamic functional joint stability , on the other hand, is defined as the condition in which the joint is stable during daily physical activities. Previous research has indicated that there is lack of a relationship between passive and dynamic functional joint stability.
To address the limitations of passive stability testing, motion analysis has been used to quantify the dynamic functional knee stability in ACL-deficient (ACL-D) and ACL-reconstructed (ACL-R) knees. Motion analysis is an advanced laboratory process by which present day electronics (i.e., video cameras) are used to integrate information from a variety of inputs in order to demonstrate and analyze the dynamics of functional activities such as gait, jump-landing, or pivoting ( Fig. 139.1 ).

Motion analysis has its roots in the early investigations of Eadweard J. Muybridge’s series of photographs in The Horse in Motion , which challenged common beliefs by demonstrating that all hooves leave the ground when they are tucked under the running horse. Current advanced, high-accuracy motion analysis systems have provided new insights and commonly disproved theories that were widely accepted. The advantages of these systems include the ease of application, safety, and quick data processing that frequently allows real-time viewing and immediate replay, while the main disadvantage is that there is relative motion between the markers and the skin. Kinematic data collection (the study of moving bodies without regard to forces) is primarily achieved with the use of high-speed and high-resolution cameras and skin markers in a laboratory environment. The markers can be either passive (reflecting light; e.g., Vicon or Motion Analysis systems) or active (generating light; e.g., Optotrak). Kinetic data are collected with the use of force transducers, commonly a force plate installed flush with the floor. As the subject performs a task on the force plate, ground reaction forces are calculated. With the use of inverse dynamics, kinetic and kinematic data can be combined to calculate joint forces and moments.
To improve the fidelity of kinetic and kinematic data collected during motion analysis, careful experimentation procedures need to be followed: (a) minimize interoperator error by having the same clinician place all markers and acquire all anthropometric measurements, (b) use a standing calibration procedure to correct for subtle misalignment of the markers that define the local coordinate system and to provide a definition of zero degrees for all movements in all planes, (c) maximize control conditions to test for true differences. (i.e., collect data from the intact leg of the ACL-R or ACL-D group and a healthy group of individuals with no history of knee injury), (d) always use the same instrumentation for all participants to maintain the same level of measurement noise, (e) increase statistical power by using an adequate sample size that is calculated by an a priori power analysis, (f) mark the location of markers when testing the same participants on different days, and (g) utilize current software to perform inverse dynamic calculations.
Reliability and validity studies with recent versions of motion analysis systems have shown that the errors generated are usually small enough to allow for accurate data interpretation. Even better validity is achieved with the use of intracortical pins that directly insert into bony segments and are attached to clusters of markers ; however, the invasiveness of this methodology has made it less popular than skin markers. Other methods of collecting kinematic data include accelerometers, electrogoniometers, electromagnetic devices, and fluoroscopy. In recent cases, important information on the mechanism of ACL injuries has been provided by data from injuries that were captured on video and subsequently analyzed. The future of in vivo biomechanical research is exciting, as new technologies have emerged that allow collection of data outside the laboratory, which opens up new avenues for research in more ecological environments such as the clinic or the sports field. These systems use either cameras that have been optimized for outdoor tracking of skin markers (e.g., Qualisys, Vicon) or markerless systems, and although promising, currently very few studies have utilized them.
In vivo biomechanics are nowadays used as a valuable tool for orthopaedic surgeons, physical therapists, and other members of the rehabilitation team involved in the prevention and treatment of ACL injuries. In recent years, important, clinically relevant biomechanical studies have greatly enhanced our understanding of the ACL-D and ACL-R knee and have influenced surgical and rehabilitation protocols. Motion analysis allows the in vivo evaluation of the ACL-D and ACL-R knee during dynamic activities (i.e., walking, pivoting, landing from a jump), something that static measures (i.e., arthrometer) are unable to do. In the following sections, we will discuss the most important adaptations after ACL injury and reconstruction, and the research findings from motion analysis studies.
Anterior Tibial Translation
The ACL is a primary restraint for anterior tibial translation, and ACL reconstruction (ACLR) has been traditionally successful in restoring normal limits during passive testing. Measuring tibial translation during functional activities is rather challenging. Patients with ACL deficiency and those at the early stages of recovery after ACLR frequently demonstrate a stiff gait strategy that involves increased co-contraction of knee muscles in an attempt to limit excessive anterior tibial translation. Tagesson et al. measured static and dynamic anterior tibial translation before, 5 weeks after, and 5 years after ACLR. They found that even though static tibial translation is not different in the ACL-R knee compared with the intact knee 5 years after surgery, dynamic translation during gait had increased over time and was significantly greater compared with the other knee.
Quadriceps Avoidance
Early biomechanical studies that assessed the motion patterns of ACL-D and ACL-R patients demonstrated that many ACL-D patients walk with a quadriceps avoidance gait characterized by decreased quadriceps activity and lower external knee flexion moment in an effort to control anterior translation of the tibia. However, Knoll et al. found that in patients with chronic ACL deficiency the quadriceps avoidance gait is less common than previously thought. It was also demonstrated that it takes 8 months after ACLR to return to preinjury gait patterns. In a study where volunteers with chronic ACL deficiency and matched healthy controls were compared, it was found that both groups demonstrated similar sagittal plane knee kinetics and kinematics during gait, step activity, and crossover hopping, despite the ACL-D group having significantly worse functional levels and significant strength deficits. A more recent study demonstrated that smaller knee excursions persist at 6 months after surgery and that some sagittal plane deficits are sex-specific. A systematic review of sagittal plane knee joint moments comparing ACL-D, ACL-R, and healthy controls supported the notion that ACL-D patients frequently demonstrate a quadriceps avoidance gait that is improved but not completely restored in ACL-R participants. In summary, the literature suggests that focusing on sagittal plane motion may not reveal the whole spectrum of neuromuscular adaptations in ACL-D and ACL-R athletes. Some patients with ACL deficiency demonstrate a quadriceps avoidance adaptation that can be detected by sagittal plane measures. ACLR results in improvement of most sagittal plane pathological deficits, although some adaptations remain.
Knee Internal Rotation
The importance of the ACL in controlling excessive knee internal rotation has been highlighted in recent years. During gait, ACL-D patients demonstrate greater tibial internal rotation. The restoration of knee internal rotation limits during functional activities is predictive of higher scores in the International Knee Documentation Committee score and the Lysholm score. In a series of experiments, Georgoulis et al. measured knee internal rotation during functional activities in ACL-D patients and after a variety of surgical reconstructions, with both the intact knees of the participants as well as a group with no history of knee injury used as controls.
In the first study, the ACL-D group exhibited significantly increased knee internal rotation range of motion during the initial swing phase of the gait cycle when compared with the ACL-R (bone–patellar tendon–bone [BPTB] autograft) and control groups. These differences did not exist when the ACL-R group was compared with the control group. Thus in this low-demand activity, ACLR restored tibial rotation to normal levels.
In a subsequent investigation with a higher demand activity (stair descend and pivoting), tibial rotation was significantly greater in the ACL-R knee as compared with the contralateral intact leg and the healthy control group (see Fig. 139.1 ). Thus ACLR did not restore knee internal rotation to normal levels, even though anterior tibial translation was restored. In a follow-up evaluation of the ACL-D participants from the previous study utilizing a within-subject design, it was found that increased tibial rotation was not restored to normal limits after ACLR with BPTB during pivoting after stair descend and drop landing. Similarly, increased knee internal rotation during pivoting was observed when ACLR was performed with a hamstrings autograft. Finally, when pivoting was assessed in subjects with the two most commonly used ACL-R grafts (hamstrings and BPTB) in the same study, it was confirmed that significantly increased tibial rotation persisted when compared with healthy controls, whereas no differences were found between the two ACL-R groups. More recent studies, however, have suggested that an oblique graft is more effective in controlling knee internal rotation during gait. Additionally, when more attention was provided to the restoration of normal tibiofemoral alignment during surgery (as indicated by the posterior cruciate ligament index), knee internal rotation during gait was more effectively restored.
In summary, when transverse plane biomechanics were assessed in a series of studies, it was consistently found that ACL-D patients demonstrate greater knee internal rotation, which is decreased but not restored to normal levels after ACLR. It was also found that the deficits are more apparent when high demand activities placing rotational stress on the knee are investigated. Current ACLR surgical techniques that place the ACL graft in a more anatomic position and restore the tibiofemoral relationship intraoperatively demonstrate promise in restoring normal knee internal rotation limits.
Gait Variability
Walking is a rhythmic activity; however, a closer examination reveals that each step is not identical to the previous or to the next one. These variations that exist among subsequent strides derive from the underlying mechanisms that produce gait. Stergiou et al. developed the optimal amount of movement variability proposition, suggesting that under healthy conditions each motor task is characterized by an optimal amount of variability that provides the human body with flexibility, adaptability, and the capacity to respond to unpredictable stimuli and stresses and environmental demands. The achievement of this optimal variability is desired during human motor development and motor learning. Stride-to-stride variability , defined as fluctuations in walking movement patterns from one stride to the next, provides a quantitative measure of functional joint stability. Evidence suggests that neuromuscular pathology is related to an increased amount of stride-to-stride variability. On the other hand, numerous studies in diverse medical areas have shown that a decreased amount of variability is also related to pathology. These investigations indicate that variations in the behavior of the biological system may be necessary to provide flexible adaptations to everyday stresses placed on the human body. Alternatively, a lack of healthy flexibility is associated with rigidity and the inability to adapt to stresses. Thus it is possible that injury or pathology can result in a loss of healthy flexibility that may not be regained despite surgical treatment (loss of complexity hypothesis).
This contradiction in the literature may be due to the usage of linear tools (e.g., standard deviation) to assess stride-to-stride variability. Linear tools only provide a measure of the amount of variability that is present in the gait pattern and may mask the true structure of motor variability. Masking occurs when strides are averaged to generate a mean picture of the subject’s gait. This averaging procedure may lose the temporal variations of the gait pattern.
Recent studies have overcome the problems of linear measures by using nonlinear tools such as the Approximate Entropy (ApEn), which allows for the identification of meaningful patterns that characterize the behavior of the locomotive system. Specific to the ACL, the ACL-D knee of participants with unilateral ACL deficiency had significantly smaller values than the intact contralateral knee ( Fig. 139.2 ). This indicated more regular and repeatable movement patterns for the injured knee and a decrease in healthy flexibility, as mentioned previously. A similar pattern was observed when the ACL-D knee was compared with healthy controls, indicating that steps are more similar to each other than in healthy knees. The decreased gait variability indicates that a patient with ACL deficiency is more careful when walking in order to eliminate any extra movements, exhibiting increased rigidity in movement patterns. When backward (instead of forward) walking was assessed, the same finding was confirmed, with ACL-D subjects demonstrating less variability in their gait not only in the ACL-D knee but also in the contralateral, uninjured leg. Backward walking is a common rehabilitation exercise after ACLR and a frequent athletic activity during sports, such as basketball and tennis; therefore the clinical significance of these findings should be emphasized for clinicians working with ACL-D patients. Subsequent studies have demonstrated that after ACLR with either a BPTB or a hamstrings autograft, there is increased variability in knee flexion–extension during gait. Thus ACL-R patients exhibit greater divergence in the movement trajectories. This could signify that patients after ACLR feel secure to increase and add extra movement. However, because the innate proprioceptive channels are damaged, gait variability and knee function are not restored to normative levels.

Surgical Techniques: Double-Bundle Reconstruction
In the past few years, the rotational role of the ACL has been studied more thoroughly. Recent cadaveric studies of the ACL have shown that it consists of two major components: the anteromedial (AM) bundle and the posterolateral (PL) bundle ( Fig. 139.3 ). The two-bundle description of the ACL has been accepted as a basis for understanding the function of the ACL. The ACL does not function as a simple band of fibers with constant tension as the knee moves; the two bundles seem to exhibit different tension patterns, and they seem to be susceptible to different forces. When the knee is extended, the PL bundle is taut and the AM bundle is moderately lax. When the knee is flexed, the femoral attachment of the ACL takes a more horizontal orientation, causing the AM bundle to tighten and the PM bundle to loosen. However, it seems that this structural morphology of the ACL cannot be restored with traditional ACLR techniques. Therefore recent techniques have been developed to better approximate the actual anatomy and physiology of the ACL. One very promising technique is the double-bundle ACLR.

The advantage of double-bundle reconstruction is that it can better replicate the function of the ACL. This is accomplished due to the reinstatement of the dual-bundle anatomy of the ligament. It is generally agreed that current ACLR techniques using BPTB or semitendinosus (ST)/gracilis (Gr) grafts, anchored in one femoral and one tibial tunnel, achieve this goal partially because they mostly replicate the AM bundle of the ACL. The role of this bundle has been well documented as resisting anterior translational loads. However, the PL bundle has received limited attention. An in vitro study has revealed that the PL bundle is important for the stabilization of the knee against rotational loads. Thus it is possible that the lack of restoration of tibial rotation after an ACLR is related to the lack of proper replication of the two ACL bundles and specifically of the PL bundle.
Even though the notion that a double-bundle ACLR will better restore dynamic knee internal rotation is based on solid biomechanical principles and it appears to decrease static rotational laxity as measured by the pivot shift test, motion analysis studies to date have not supported this proposal. When a cutting task was examined, participants with double-bundle ACLR did not demonstrate lower knee internal rotation than those with the traditional single-bundle ACLR. Similarly, when patients with chronic ACL deficiency were randomly assigned to receive either a single- or a double-bundle ACLR, they demonstrated similar knee internal rotation when tested 6 months after surgery. Another study confirmed that there are no differences in the total amount of knee rotation during a dynamic cutting task, but the single-bundle group had a greater external rotation shift at the midpoint of the range of movement, while the double-bundle group was more similar to the control group. Future studies should evaluate the long-term effects of double-bundle reconstruction on knee kinematics and kinetics during functional activities and, more importantly, on the prevention of posttraumatic osteoarthritis.
Surgical Techniques: Tunnel Placement
Another very promising technique that has been developed recently to better approximate the actual anatomy and physiology of the ACL is the more oblique femoral tunnel placement. A more oblique placement of the femoral tunnel can also affect rotational stability. The basic advantages of this technique are that it is not as surgically demanding as others (i.e., a two-bundle reconstruction) and that the only difference from the current techniques is in the setting of the femoral tunnel in a more oblique location (between 9 and 10 o’clock for a right knee). Previous techniques use a vertical orientation approximately at the 11-o’clock position ( Fig. 139.4 ). Several studies used in vitro methodology to examine the more oblique placement of the femoral tunnel using either the BPTB or the ST/Gr autograft. They found that the more oblique placement of the femoral tunnel more effectively resisted rotational loads. This can be attributed to the fact that the PL bundle of the ACL is located more horizontally and toward the 9-o’clock position of the femur (for the right leg) and is important for the stabilization of the knee against rotational loads. Thus a more oblique placement can better replicate the PL bundle and result in increased resistive ability to rotational forces.

As mentioned previously, early results demonstrate great promise that a more oblique ACL graft orientation in combination with careful restoration of the tibiofemoral anatomy intraoperatively results in superior clinical outcomes and better restoration of knee internal rotation during dynamic activities.
Closely related to graft orientation is the use of an AM or transtibial portal during ACLR. The transtibial technique where the femoral tunnel is drilled through the tibial tunnel is the most traditional and popular technique, with its main advantage being less surgical time. The AM technique where the femoral tunnel is drilled through an arthroscopic portal with the knee in maximum flexion allows the surgeon more flexibility to place the graft in an oblique position. A meta-analysis comparing the outcomes of patients with ACLR using either the transtibial or the AM technique found that the AM group had greater range of motion and better passive knee stability in the initial 2 years after reconstruction. Higher functional scores and better outcomes in lateral agility were also found in the AM group in a study that controlled for the postsurgical rehabilitation program. A motion analysis study also demonstrated that the AM portal group had better control of anterior translation and knee internal rotation during gait compared with the transtibial group.
Excessive Knee Internal Rotation and Posttraumatic Osteoarthritis
Degeneration of the knee joint and eventual development of osteoarthritis are associated with ACL deficiency. Longitudinal follow-up studies have shown that ACL deficiency leads to the development of chondral injuries, meniscal tears, degeneration of the articular cartilage, and eventually posttraumatic arthritis. However, similar problems have also been found longitudinally in the ACL-R knee. Even more disturbingly, such findings are commonly observed shortly after the reconstruction. Therefore traditional ACLR does not appear to have a protective effect from degenerative changes.
Excessive tibial rotation in the ACL-D or ACL-R knee may be an abnormal movement mechanism that degenerates cartilage, resulting in osteoarthritis. Traditional ACLR procedures cannot exactly replicate normal ACL anatomical complexity or restore normal tibiofemoral kinematics at the knee joint, thus leading to pathological movement patterns. The abnormal rotational movements of the articulating bones at the knee could result in the applications of loads at areas of the cartilage that are not commonly loaded in a healthy knee. Normal functional loading results in increased resistance of the cartilage by improving the mechanical stiffness and the proteoglycan content of the tissue. Furthermore, in joints that are prone to arthrosis, it has been found that the best-preserved cartilage areas are those of higher loading. Therefore in a healthy knee there are areas that are commonly loaded and others that receive a lower load. These latter areas, due to lack of sufficient cartilage, may not be able to withstand the newly introduced loading that is the result of the abnormal rotational movements of the articulating bones. Over time, this could lead to knee osteoarthritis.
Although the majority of those who tear their ACL goes on to develop early, posttraumatic knee osteoarthritis, there are some who manage to maintain a healthy and active lifestyle for several decades. This observation is promising, as it suggests that there may be aspects of the management of the ACL-injured patient that may protect from future disability. It is currently unclear if these aspects are surgical, biomechanical, genetic, or other. Future studies should investigate if patients who experience a normalization of knee internal rotation during functional activities are protected from developing knee osteoarthritis.
Conclusion
In this chapter, we presented how motion analysis and in vivo biomechanics have been used to examine dynamic motion in ACL-D and ACL-R patients. Athletic activities that include landing from a jump, pivoting, and cutting are more demanding than walking and involve both anterior and rotational loading of the knee. Patients with ACL deficiency demonstrate greater knee internal rotation that is reduced but not restored after traditional ACLR techniques. Excessive knee rotation may lead to the abnormal loading of cartilage areas that are not commonly loaded in the healthy knee and subsequent osteoarthritis. More recent, anatomic ACLR techniques appear to restore knee internal rotation to more normal levels and result in better function; it remains unclear if this has a protective effect from early, posttraumatic knee osteoarthritis.
In addition, we discussed how motion analysis can assist in the improvement and development of new surgical procedures and grafts that could restore not only the pathological anterior drawer but also the increased knee rotation. Attempts to achieve this include a more horizontally oriented femoral tunnel or a double-bundle ACL reconstruction. Experimental methodology such as that presented in this chapter can examine the advantages and disadvantages of these different surgical procedures, whether it be the graft material or the tunnel positioning, keeping always in mind the importance of reproducing the actual ACL anatomy during the reconstruction.
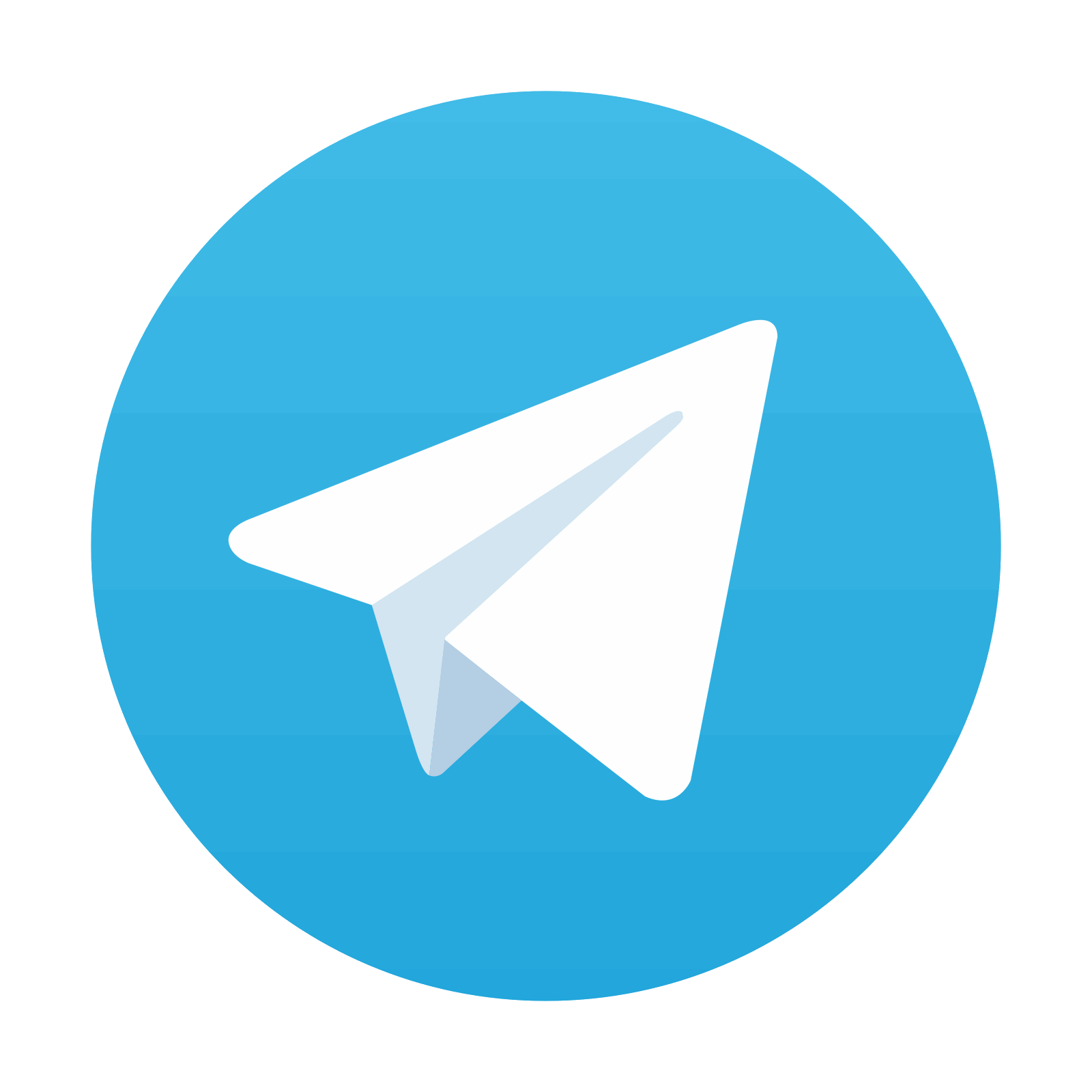
Stay updated, free articles. Join our Telegram channel

Full access? Get Clinical Tree
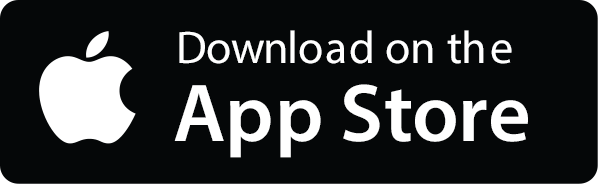
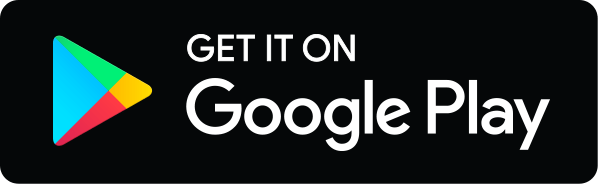