Morphology and Whiplash Injuries
Brian D. Stemper
Brian D. Corner
Whiplash injuries lead to significant worldwide disability, affecting a variety of individuals from younger to older age groups, men and women, and vehicle occupants of varying sizes. Across a number of clinical investigations, it has become clear that certain populations report significantly higher numbers of whiplash injuries. This implies an underlying cause for increased susceptibility in those groups. The cause is likely to include biomechanical, anatomical, physiological, psychological, or sociological factors. The most often cited group of individuals with high susceptibility is the female gender. Women have been consistently identified as being more susceptible to whiplash injuries than men. In fact, clinical and epidemiological literature has indicated that women are 1.4-3.0 times more likely to sustain whiplash injury [7, 11, 27, 28, 31, 40]. Experimental research has, for the most part, supported this clinical finding by identifying different biomechanics of the head, neck, and cervical spine in female volunteers and postmortem human subjects (PMHS). Explanations for these biomechanical differences have included differing body anthropometry, spinal kinematics, or cervical spine geometry between men and women. This chapter outlines biomechanical differences between men and women subjected to low velocity rear impacts, and highlights possible biomechanical and morphological explanations.
Literature reviewed is not intended to be comprehensive, but to outline concepts related to biomechanical susceptibility.
Literature reviewed is not intended to be comprehensive, but to outline concepts related to biomechanical susceptibility.
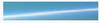
A fundamental understanding of head-neck and cervical spine biomechanics during low velocity rear impacts is required to understand the method by which biomechanical differences between men and women affect the risk and severity of injury. Low-speed automobile rear-impact collisions lead to motions of the occupant’s head-neck complex that are unique to other vehicular loading vectors (i.e., frontal or side impact). These motions were studied on the occupant, component, and spinal levels using experimental and computational modeling techniques. At the occupant level, the impacted vehicle is accelerated forward, and the seat begins to interact with the occupant primarily through the seat back. The seat back begins to drive the occupant’s thorax anteriorly. However, inertia of the body deforms the seat back, and deflects it rearward. Deflection magnitude is dependent upon the mass and sitting height of the occupant, as well as the seat back characteristics. Eventually, the seat back overcomes the inertial loads from the torso and begins to rebound forward, driving the occupant’s body forward and eventually into the shoulder harness of the seat belt.
Occupant interactions with the seat back, as described above, drive head-neck biomechanics during the rear impact, which, in turn, produce signature kinematics of the cervical spine. Specifically, torso interaction with the seat back results in anterior acceleration of the thorax [49], which is quantified at the upper thoracic level (i.e., T1) [59, 71]. The magnitude of anterior thoracic acceleration is generally correlated with the rear-impact severity, measured as the forward acceleration or change in velocity (δ-V) of the vehicle. The head lags as the thorax is accelerated anteriorly because there are no forces placed on the head during the initial stages of the impact. This leads to retraction motion of the head relative to the thorax, which is characterized as posterior displacement of the head without an appreciable change in angular orientation (i.e., flexion or extension) [38, 39]. As the magnitude of
retraction motion increases, forces are eventually transferred up the cervical spine, and the head begins to rotate posteriorly into extension to strike the head restraint. Smaller head-to-head restraint horizontal distances (i.e., backset) or active head restraint designs can lead to head restraint contact during the retraction phase and prior to head rotation. Rebound from the head restraint occurs and the head rotates forward into flexion. Forward flexion is eventually limited by contraction of the neck muscles or chin-to-chest interaction.
retraction motion increases, forces are eventually transferred up the cervical spine, and the head begins to rotate posteriorly into extension to strike the head restraint. Smaller head-to-head restraint horizontal distances (i.e., backset) or active head restraint designs can lead to head restraint contact during the retraction phase and prior to head rotation. Rebound from the head restraint occurs and the head rotates forward into flexion. Forward flexion is eventually limited by contraction of the neck muscles or chin-to-chest interaction.
Inertial loading of the head-neck complex drives cervical spine kinematics during the rear impact. Head retraction produces the signature S-shaped curvature of the cervical spine, which is characterized by flexion at cranial cervical segments (i.e., C0-C3) and extension at caudal segments (i.e., C3-C7), with a transition region in the middle cervical spine (Fig. 3-1) [23, 53]. This nonphysiologic curvature results in abnormal and increased motions of the facet joints that can produce elevated strains in the capsular ligaments [33, 37, 52, 54] that may exceed subcatastrophic failure thresholds [43] and initiate a nociceptive response [12]. Other cervical spine injury theories center around the retraction phase/S-shaped curvature of the cervical spine or head extension phase, and include localized hyperextension resulting in injury to anterior spinal structures [9, 58, 71], and pressure gradients in the cervical spinal canal that can produce nerve root injury [1, 3, 64].
![]() FIGURE 3-1 The phases of cervical spine kinematics during a low velocity vehicular rear impact include an s-shaped curvature between 25 and 100 ms after the initiation of thoracic acceleration that is characterized by flexion at cranial segments and extension at caudal segments. (Used with permission from grauer et al. [23].) |
The remainder of this chapter will focus on developing an under standing of how occupant and cervical spine morphology can affect rear impact biomechanics, as outlined above. These comparisons will be primarily focused on gender due to the inherent differences in body shape/size between men and women, and the significant amount of research dedicated to that factor.
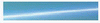
Gender differences provide a suitable model for investigation of morphology-related differences in cervical spine biomechanics during low velocity rear impacts. Although other differences exist, as highlighted below, average female body size is smaller than that of men for a majority of metrics [19, 20]. Outlining metrics specific to rear impact biomechanics is a primary focus of this chapter. However, the case for increased female susceptibility must be made, and an understanding of biomechanical differences between men and women subjected to low velocity rear impact must be provided prior to outlining differences in morphology that can contribute to those changes.
Clinical studies have provided sufficient evidence to indicate that female occupants of motor vehicles sustain a higher number of whiplash injuries. However, due to the focus on injured patients and an inability to control or measure exposure characteristics, those studies provide limited information on the mechanism by which women become more susceptible. In contrast, experimental studies demonstrate specific biomechanical changes between men and women that can contribute to greater injury susceptibility during highly repeatable and identical exposures. Accordingly, outlining biomechanical differences between those two populations has been the focus of a number of experimental investigations.
Experimental investigations that have defined biomechanical differences between men and women have included human volunteers, PMHS, and computational modeling. Studies incorporating volunteers have recreated rear impact accelerations up to 8 km/h using vehicles [4] or horizontal acceleration sleds [6]. Due to ethical considerations,
those studies were intentionally focused on producing rear impacts below the threshold for injury. Nonetheless, the clinical condition of the volunteers prior to and following experimentation was reported. Although sample sizes were generally small and statistical analysis was limited, Brault and colleagues [5] identified a significantly longer time period until recovery from initial whiplash-type symptoms (12 compared to 2 hours) following 4 km/h rear impacts. Duration of symptoms was also greater in women following 8 km/h rear impacts (24 compared to 8.8 hours), although not statistically significant.
those studies were intentionally focused on producing rear impacts below the threshold for injury. Nonetheless, the clinical condition of the volunteers prior to and following experimentation was reported. Although sample sizes were generally small and statistical analysis was limited, Brault and colleagues [5] identified a significantly longer time period until recovery from initial whiplash-type symptoms (12 compared to 2 hours) following 4 km/h rear impacts. Duration of symptoms was also greater in women following 8 km/h rear impacts (24 compared to 8.8 hours), although not statistically significant.
From a biomechanical standpoint, studies incorporating human volunteers also reported significant differences in head-neck and torso kinematics between men and women. For example, although initial head-to-head restraint distance (i.e., backset) was typically shorter [6, 65], female volunteers demonstrated horizontal head accelerations with higher magnitude and earlier peak values than their male counterparts [6, 24, 31]. Smaller backset was thought to be the result of shorter sitting height in female volunteers, which placed the head closer to the posteriorly inclined head restraint than in men (Fig. 3-2) [6]. Female volunteers also demonstrated greater thoracic acceleration than men [6, 24, 31]. This finding is of particular interest in the light of rear impact severity being identical for male and female volunteers. Greater thoracic accelerations indicate that the driving force for headneck inertial loading was greater in women when subjected to identical rear impacts than in men. An explanation for this finding was hypothesized to be that women generally had lower body mass and a lower center of mass that contributed to decreased compression of the seat back foam/springs and an overall decrease in seat back deflection due to a shorter moment arm with regard to the pivot point [69]. Therefore, the seat back absorbed less energy, and female occupants experienced more severe loading of the head-neck complex for the same rear impact severity.
Significant differences in cervical spine biomechanics were also identified between men and women. Using a limited number of male and female volunteers and high-speed X-ray imaging, Ono and colleagues [35] identified exaggerated S-shaped curvature in the cervical spines of female volunteers (n = 2) compared to their male counterparts (n = 4).
Stemper and colleagues [53] incorporated intact head-neck specimens and demonstrated significantly (P < 0.05) greater cervical spine intervertebral rotations and enhanced cervical S-curvature. The same group also reported significantly greater absolute facet joint motions [54] and significantly greater facet joint motions normalized by the magnitude of segmental extension [56] in female volunteers. The importance of measuring spinal kinematics lies in the indirect quantification of soft tissue distortion. Intervertebral motions lead to a specific pattern of soft tissue distortion. Extension results in tension of anterior tissues, whereas flexion leads to tension of the posterior tissues. Inertial loading of the head-neck complex during low velocity rear impacts involves a large component of shear force [49], which leads to shear loading across the entire joint that is resisted in large part by the facet joint capsular ligaments due to their sagittal orientation in the cervical spine [36]. Quantification of these motions provides an indirect
measure of tissue distortion and injury risk, with greater motions associated with a greater risk or severity of soft tissue injury. Therefore, greater spinal motions demonstrate greater injury risk for women subjected to identical rear impact loading. Possible explanations for those differences are provided in the following sections.
Stemper and colleagues [53] incorporated intact head-neck specimens and demonstrated significantly (P < 0.05) greater cervical spine intervertebral rotations and enhanced cervical S-curvature. The same group also reported significantly greater absolute facet joint motions [54] and significantly greater facet joint motions normalized by the magnitude of segmental extension [56] in female volunteers. The importance of measuring spinal kinematics lies in the indirect quantification of soft tissue distortion. Intervertebral motions lead to a specific pattern of soft tissue distortion. Extension results in tension of anterior tissues, whereas flexion leads to tension of the posterior tissues. Inertial loading of the head-neck complex during low velocity rear impacts involves a large component of shear force [49], which leads to shear loading across the entire joint that is resisted in large part by the facet joint capsular ligaments due to their sagittal orientation in the cervical spine [36]. Quantification of these motions provides an indirect
measure of tissue distortion and injury risk, with greater motions associated with a greater risk or severity of soft tissue injury. Therefore, greater spinal motions demonstrate greater injury risk for women subjected to identical rear impact loading. Possible explanations for those differences are provided in the following sections.
![]() FIGURE 3-2 Studies have identified decreased backset in women due to their relatively shorter sitting height and rearward sloping seat back. However, injury risk remains higher in women than in men. (Used with permission from Carlsson et al. [6].) |
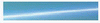
In 2003, Farmer and colleagues [15] reported that newer head restraint designs with active mechanisms or improved geometric attributes were reducing the risk of whiplash injury among women while having very little effect on male drivers. While clinical studies since that time continue to demonstrate female preponderance [28, 61], changes in women more than in men point to a fundamental difference in automobile seat interaction with the occupant. Seats are designed under a “one size fits all” approach and must provide protection for adult occupants of all sizes from the 5th percentile woman (59-inch stature, 108-pound body weight) to the 95th percentile man (74-inch stature, 223-pound body weight) and beyond. However, automobile safety is often based on the 50th percentile male, meaning that occupants with body sizes further away from that size may not be as well protected.
Given identical seat configurations, experimental studies reported that head-to-head restraint backset in women was less than in men due to rearward incline of automobile seats and decreased sitting height for average women compared to average men. Sitting height for average women is 2.4 inches shorter than sitting height for average men [19, 20]. This means that the posterior aspect of the head for the comparatively shorter woman is positioned closer to the rearward sloping head restraint than the posterior aspect of the male head (Fig. 3-2). Studies demonstrated that decreased backset is associated with decreased injury risk [32, 55, 66]. Therefore, it would be logical to assume that decreased backset in female occupants should be associated with a lower injury risk. However, clinical studies demonstrate that this is not the case.
![]() FIGURE 3-3 Vertical mismatch between the sitting height of the occupant and head restraint position may be one explanation for increased injury risk in female occupants. (Used with permission from DeRosia et al. [10].)
![]() Stay updated, free articles. Join our Telegram channel![]() Full access? Get Clinical Tree![]() ![]() ![]() |